With the advent of multiple highly effective treatment options, minimal residual disease (MRD) measurements gained interest as a prognostic marker in chronic lymphocytic leukemia. This article provides a model explaining the clinical significance of MRD in different clinical situations and reviews available data to support that model. Factors with a possible impact on the clinical significance of MRD are discussed: technique for MRD quantification, treatment regimen, clinical response, time-point for sampling, and the additional impact of molecular risk features. Also described are data supporting the use of MRD assessments as a surrogate end-point in randomized clinical trials.
Key points
- •
The tumor load that remains after therapy in patients with chronic lymphocytic leukemia can be quantified by modern minimal residual disease (MRD) technology with a 1000-fold higher sensitivity compared with clinical staging.
- •
Quantitative multicolor flow cytometry or ASO primer real-time quantitative IGH PCR should be applied for standardized MRD monitoring with results evaluated with respect to thresholds at 10 −4 and additionally at 10 −2 .
- •
MRD is an independent predictor of progression-free and overall survival after conventional induction therapy adding significantly to the prognostic power of known pretreatment prognostic factors.
- •
MRD kinetics after allogeneic stem cell transplantation identifies patients who experience efficacious graft-versus-leukemia activity that leads to complete eradication of the malignant clone.
- •
MRD qualifies as a surrogate marker to identify the superior treatment arm in randomized clinical trials by a lower proportion of MRD-positive patients, but its general application for tailoring therapy in individual patients outside clinical trials is currently discouraged.
Rationale for minimal residual disease assessments in chronic lymphocytic leukemia
The number of leukemic cells in the body of patients with chronic lymphocytic leukemia (CLL) decreases when they respond to treatment. Considering residual leukemic cells as the source of relapse, one would intuitively hypothesize that a high tumor burden after therapy is associated with a short progression-free survival (PFS). Patients in whom regrowth of the leukemic clone starts from a larger number of malignant cells suffer an earlier clinical relapse, because a faster reoccurrence of clinical signs and symptoms of the leukemia is expected.
Minimal residual disease (MRD) is a very sensitive measurement of the tumor load that remains in the body of a patient after therapy. Therefore, the attainment of an MRD-negative status (ie, no detection of leukemia by sensitive methods) is expected to predict for long PFS. Because current MRD methods are approximately 1000-fold more sensitive than clinical staging, MRD status should much more exactly predict time to relapse. Moreover, modern MRD assessments are considered more accurate than clinical staging to quantitatively reflect the number of residual leukemia cells after treatment.
Current conventional therapy cannot cure CLL, as exemplified by a lack of a plateau in Kaplan-Meier plots for PFS. It is expected, therefore, that all patients, even those from good risk subgroups treated with intensive therapy, eventually relapse. Consequently, the efficacy of therapies in CLL is primarily judged by median PFS (with overall survival used as a secondary end-point), thus resembling current concepts in many other peripheral B-cell malignancies (eg, multiple myeloma, follicular and mantle cell lymphomas ). Most current treatment strategies in CLL are characterized by an induction phase to reduce the tumor load followed by treatment-free observation. The general concept of MRD as a predictor of PFS after such a conventional induction therapy is depicted in Fig. 1 A.
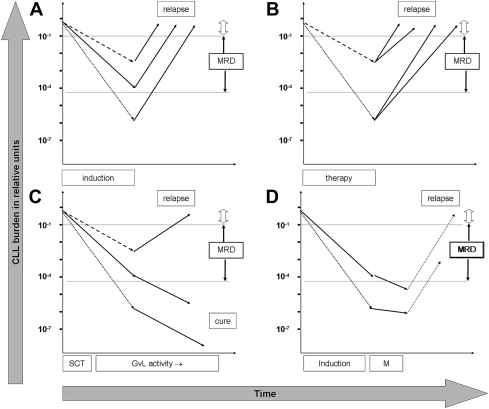
The predictive power of MRD in this situation depends on the assumption that regrowth kinetics are similar between different patients, do not relate to the MRD level achieved, and are constant over time. Logically, patients or patient cohorts who show faster regrowth kinetics are expected to present earlier with clinical signs or symptoms of relapse even if they have achieved low MRD levels directly after induction therapy (see Fig. 1 B).
The absence of MRD is a prerequisite for but not identical to cure. Any MRD measurement has a finite sensitivity, so that the persistence of residual CLL cells below that limit of detection is possible. The minimum number of residual CLL cells necessary to eventually cause a relapse is unknown. However, patients who were MRD-negative at the lowest level measurable with the most sensitive available technology (1 CLL cell in more than 100,000 benign leukocytes) can show relapse. That means that if there is a threshold for cure in CLL, it must be lower than 10 −5 . The likelihood of relapse is therefore not only influenced by the treatment effects one can directly measure but also by treatment effects that occur in CLL cells below the limit of detection. It is assumed that treatment effects are similar below and above the detection threshold of MRD. To predict what will likely happen below the MRD limit of detection it is important to consider the duration and efficacy of treatment when interpreting MRD results.
Patients successfully treated with an allogeneic stem cell transplantation receive a very effective and long-lasting therapy mediated by graft-versus-leukemia effects, thus contrasting with the short-term efficacy in the induction therapy setting. After allogeneic transplantation the disappearance of CLL cells below the lowest measurable MRD level is likely a surrogate marker for effective graft-versus-leukemia activity. Current data suggest that this activity not only reduces the malignant clone within the range of measurable MRD levels but is equally potent in suppressing or eradicating the disease at even lower levels, thus finally leading to cure in many patients (see Fig. 1 C).
An intermediate situation between allogeneic stem cell transplantation and conventional therapy might be envisaged when patients receive an effective maintenance therapy that should lead to tumor suppression while given, thus modulating the kinetics of CLL regrowth (see Fig. 1 D).
MRD assessments about 1000-fold extend the ability to directly measure treatment effects in CLL compared with clinical assessments, thus allowing better prediction of PFS after conventional therapy and better evaluation of graft-versus-leukemia activity after allogeneic stem cell transplantation.
Rationale for minimal residual disease assessments in chronic lymphocytic leukemia
The number of leukemic cells in the body of patients with chronic lymphocytic leukemia (CLL) decreases when they respond to treatment. Considering residual leukemic cells as the source of relapse, one would intuitively hypothesize that a high tumor burden after therapy is associated with a short progression-free survival (PFS). Patients in whom regrowth of the leukemic clone starts from a larger number of malignant cells suffer an earlier clinical relapse, because a faster reoccurrence of clinical signs and symptoms of the leukemia is expected.
Minimal residual disease (MRD) is a very sensitive measurement of the tumor load that remains in the body of a patient after therapy. Therefore, the attainment of an MRD-negative status (ie, no detection of leukemia by sensitive methods) is expected to predict for long PFS. Because current MRD methods are approximately 1000-fold more sensitive than clinical staging, MRD status should much more exactly predict time to relapse. Moreover, modern MRD assessments are considered more accurate than clinical staging to quantitatively reflect the number of residual leukemia cells after treatment.
Current conventional therapy cannot cure CLL, as exemplified by a lack of a plateau in Kaplan-Meier plots for PFS. It is expected, therefore, that all patients, even those from good risk subgroups treated with intensive therapy, eventually relapse. Consequently, the efficacy of therapies in CLL is primarily judged by median PFS (with overall survival used as a secondary end-point), thus resembling current concepts in many other peripheral B-cell malignancies (eg, multiple myeloma, follicular and mantle cell lymphomas ). Most current treatment strategies in CLL are characterized by an induction phase to reduce the tumor load followed by treatment-free observation. The general concept of MRD as a predictor of PFS after such a conventional induction therapy is depicted in Fig. 1 A.
The predictive power of MRD in this situation depends on the assumption that regrowth kinetics are similar between different patients, do not relate to the MRD level achieved, and are constant over time. Logically, patients or patient cohorts who show faster regrowth kinetics are expected to present earlier with clinical signs or symptoms of relapse even if they have achieved low MRD levels directly after induction therapy (see Fig. 1 B).
The absence of MRD is a prerequisite for but not identical to cure. Any MRD measurement has a finite sensitivity, so that the persistence of residual CLL cells below that limit of detection is possible. The minimum number of residual CLL cells necessary to eventually cause a relapse is unknown. However, patients who were MRD-negative at the lowest level measurable with the most sensitive available technology (1 CLL cell in more than 100,000 benign leukocytes) can show relapse. That means that if there is a threshold for cure in CLL, it must be lower than 10 −5 . The likelihood of relapse is therefore not only influenced by the treatment effects one can directly measure but also by treatment effects that occur in CLL cells below the limit of detection. It is assumed that treatment effects are similar below and above the detection threshold of MRD. To predict what will likely happen below the MRD limit of detection it is important to consider the duration and efficacy of treatment when interpreting MRD results.
Patients successfully treated with an allogeneic stem cell transplantation receive a very effective and long-lasting therapy mediated by graft-versus-leukemia effects, thus contrasting with the short-term efficacy in the induction therapy setting. After allogeneic transplantation the disappearance of CLL cells below the lowest measurable MRD level is likely a surrogate marker for effective graft-versus-leukemia activity. Current data suggest that this activity not only reduces the malignant clone within the range of measurable MRD levels but is equally potent in suppressing or eradicating the disease at even lower levels, thus finally leading to cure in many patients (see Fig. 1 C).
An intermediate situation between allogeneic stem cell transplantation and conventional therapy might be envisaged when patients receive an effective maintenance therapy that should lead to tumor suppression while given, thus modulating the kinetics of CLL regrowth (see Fig. 1 D).
MRD assessments about 1000-fold extend the ability to directly measure treatment effects in CLL compared with clinical assessments, thus allowing better prediction of PFS after conventional therapy and better evaluation of graft-versus-leukemia activity after allogeneic stem cell transplantation.
Techniques to detect MRD
Overview on Available Technology for MRD Detection
“Minimal residual disease” is not a registered trademark. The only feature common to all methods labeled “MRD” is the ability to detect CLL cells in patients without cytologic and histologic evidence of disease. However, clinicians have witnessed continuous technologic improvements over the last 30 years so that today’s advanced methods are up to 100-fold more sensitive than the very early assays. In addition, state-of-the-art MRD technology is still not applied in all treatment protocols so that results with different sensitivity, quantitative accuracy, and reproducibility are reported. Differences in applied technology and data interpretation considerably contribute to different findings on the clinical significance of MRD. To allow clinicians a profound appraisal of the published data, general principles and performance features of MRD methods are reviewed next and summarized in Table 1 . For reasons outlined in the following sections it is mandatory that sensitive, quantitatively accurate, standardized methods are used today.
Method | Typical Sensitivity | Quantitative | Typical Quantitative Range | Sample Requirements | Assay Performance Influenced by | Standardization of Laboratory Procedures and Data Interpretation | Selected References on Methodologic Aspects |
---|---|---|---|---|---|---|---|
Consensus IGH PCR | 10 −3 | No | n/a | 10 6 total leukocytes needed | Clone (PCR product size), number of benign B cells | Yes | |
Nested ASO IGH PCR | Up to 10 −6 | No | n/a | 10 6 total leukocytes needed | Clone (CDR3 region of patient) | None | |
ASO IGH RQ-PCR | 10 −5 | Yes | 10 −4 | 10 6 total leukocytes needed | Clone (CDR3 region of patient) | Yes | |
Flow cytometry CD19/CD5 | 5 × 10 −2 | No | n/a | Fresh (48 h) | Number of benign B cells Activation of benign B cells by previous therapy | None | |
Flow cytometry CD19/CD5/κ/λ | 10 −2 | No | n/a | Fresh (48 h) | Number of benign B cells Activation of benign B cells by previous therapy | None | |
Quantitative four-color MRD flow | 10 −4 | Yes | 10 −4 | Fresh (48 h), ≥10 7 total leukocytes needed | Number of available leukocytes | Yes |
Sensitive detection of MRD in CLL is currently possible using either the rearranged immunoglobulin genes of the CLL clone (polymerase chain reaction [PCR]-based MRD detection) or the unique immunophenotype of CLL cells (flow cytometric MRD detection). Both methods have advantages and drawbacks. Flow cytometry is generally more broadly available; faster (results available in 6 hours); less labor-intensive; and requires less specialized equipment. However, sensitive flow cytometry requires 20-fold more sample material than PCR. PCR-based MRD approaches target DNA, which is stable even at room temperature. PCR-based MRD assessments can therefore successfully be performed on older and even stored samples, whereas flow cytometry requires analysis within 48 hours from sample collection. Advanced PCR approaches can require several weeks before the results become available.
PCR-Based Methods for MRD Detection
PCR-based approaches in CLL are based on the detection of the clones’ unique rearranged immunoglobulin gene. This so-called “genetic fingerprint” is inherited from the founder B-cell of the CLL clone in an individual patient.
The simplest technique to detect rearranged immunoglobulin genes uses primers matching conserved regions adjacent to the most diverse regions of the immunoglobulin gene (the complementary determining region 3 [CDR3]). PCR products of different lengths are obtained when such consensus primers are used in polyclonal samples. In contrast, CLL samples consisting of genetically uniform cells give rise to one single PCR product of defined length. The sensitivity of this method, consensus-primer PCR, depends on the length of the monoclonal PCR product that represents the patient with CLL. The CLL clones with CDR3 lengths that are rarely represented in the benign B-cell repertoire can be more sensitively detected than PCR products of average size. Moreover, the abundance of residual benign B-cells impacts on sensitivity. Therefore, this method can be rather sensitive in certain clinical situations but its sensitivity differs by sample and is often inferior to 10 −4 .
More sensitive are approaches that use primers directly matching the highly diverse CDR3. As opposed to the consensus PCR, which measures the length of the CDR3, this method identifies a part of the CDR3’s sequence. However, the design of such an allele-specific oligonucleotide (ASO) primer requires sequencing of the CDR3 in diagnostic material of each patient before the design of a suitable, individual assay. Although the sensitivity (up to 10 −5 ) and specificity of ASO primer PCR is high and largely independent of the number of polyclonal B-cells in the sample, ASO primers differ in affinity to background cells. The sensitivity and specificity of individual ASO primer assays are consequently different from patient to patient. ASO primer PCR is not quantitative. The combination of a consensus PCR with a ASO primer–based PCR (nested ASO IGH PCR) can further improve specificities and sensitivities (reportedly up to 10 −6 ) but is a nonquantitative method.
Real-time quantitative (RQ) PCR measures the release of fluorochrome from a labeled probe during the PCR amplification process. The number of PCR cycles required until this fluorescence signal becomes detectable can be converted into the tumor content of the sample. When RQ technology was combined with the ASO primer approach PCR-based quantification in lymphoid malignancies became possible. Although ASO primer IGH RQ PCR is a very sensitive method for MRD quantification, it requires testing of the ASO assay for each individual patient. Two parameters, the quantitative range and the sensitivity, have to be determined experimentally for each individual ASO primer assay, making the method expensive and labor-intensive. However, definitions for quantitative range and sensitivity, and a standardized procedure for ASO RQ PCR-based MRD detection have been widely accepted, so that results are reproducible between laboratories.
Flow Cytometric Methods to Detect MRD
Flow cytometric MRD analysis identifies CLL cells as a population of leukocytes that exhibit a combination of markers that are not observed in benign hematopoiesis. The following features are used for flow cytometric MRD detection in CLL:
- •
Expression of CD5 (otherwise seen on T cells and on a minor subpopulation of benign B cells)
- •
A predominance of either kappa or lambda immunoglobulin light chains (light chain restriction)
- •
Underexpression or overexpression of select antigens on CLL B cells when compared with benign CD5 + B cells.
The simplest method to measure MRD by flow cytometry relies on the simultaneous detection of CD5 together with the B-cell markers CD19 or CD20. Cases with more than 25% CD5 + B cells of the total B cells were considered MRD positive. The approach lacks quantitative accuracy and specificity because the proportion of mature benign B cells that coexpress CD5 can reach at least 80% after intensive therapy. The combination of light chain restriction with CD5/CD19 assessments can improve the specificity, but is still hampered by a low sensitivity.
Current quantitative multicolor MRD flow is based on expression differences between benign CD19 + CD5 + B cells and CLL cells. First systematic investigations in those expression differences revealed the use of CD20, CD79b, and CD38 for identification of CLL cells in samples that also contained normal B cells. Later, the overexpression of CD43 and the combined underexpression of CD81 and CD22 were described as useful for MRD detection. An international consortium under the auspices of the European Research Initiative on CLL tested 10 different four-color combinations and found the antibody-combinations CD20/CD38/CD19/CD5, CD22/CD81/CD19/CD5, and CD43/CD79b/CD19/CD5 to yield the most reproducible and specific results. The international consortium also developed standard operating procedures for MRD cell identification and quantification to further improve reproducibility between laboratories. The combined evaluation of expression levels (ie, number of molecules per cell) of the aforementioned antigens allows the distinction between benign B cells and minute CLL cell populations without overlap, so that the accurate flow cytometric quantification of residual leukemic cells at a level of 10 −4 became possible. Because CLL cells and all benign cells can be reliably distinguished using the standardized antibody combinations, the sensitivity of MRD flow primarily depends on the availability of sufficient numbers of leukocytes in a sample. Virtually all available data on the clinical significance of sensitive MRD quantification in CLL are based on the harmonized quantitative four-color MRD flow approach or, in case of studies that started before its publication, on slight modifications of it (see Table 2 for an overview).
No. | Study (ref) | Number of Patients Assessed Using MRD | Therapy | Technique | MRD Threshold | PFS by MRD Group (MRD+ vs MRD−) | P Value |
---|---|---|---|---|---|---|---|
1 | Moreno et al, 2006 | 17 | Auto SCT | ASO RQ-PCR | 10 −5 | 19 mo vs NR | .02 |
22 | Quantitative three- or four-color MRD flow | 10 −4 | 16 mo vs 75 mo | <.001 | |||
2 | Bosch et al, 2008 | 44 | FCM | Quantitative four-color MRD flow | 10 −4 | MRD+ CR < MRD− CR (response duration) | .2 |
3 | Kwok et al, 2009 | 137 | Various a | Quantitative four-color MRD flow | 10 −4 | 24 mo vs 91 mo | <.001 |
4 | Böttcher et al, 2012 | 290 b | FC/FCR | Quantitative four-color MRD flow | 10 −4 and 10 −2 | ≥10 −2 15 mo ≥10 −4 to <10 −2 41 mo <10 −4 69 mo | <.001 |
5 | Fischer et al, 2012 | 45 | BR | Quantitative four-color MRD flow | 10 −4 and 10 −2 | ≥10 −2 12 mo ≥10 −4 to <10 −2 32 mo <10 −4 NR | <.001 |
6 | Pettitt et al, 2012 | 29 | alemtuzumab-HDMP | Quantitative four-color MRD flow | 10 −4 | 10 mo vs 24 mo | .009 |
a For example, chlorambucil, fludarabine, FC (-R), FCM (-R), alemtuzumab, auto SCT, or various others.
b Considering only 290 patients with MRD assessments in peripheral blood after induction therapy (final restaging).
A comparative analysis in 530 samples subsequently demonstrated that four-color MRD flow allows CLL quantification down to the 10 −4 threshold with equal sensitivity as ASO primer RQ-PCR. The PCR method was more effective in detecting CLL cells below that threshold. There was a high degree of quantitative correlation between PCR and MRD flow ( r = 0.95), finally proving by cross-evaluation that both methods are quantitatively reliable. MRD flow showed identical accuracy compared with ASO IGH RQ-PCR regardless of the therapeutic regimen (FCR, fludarabine-cyclophospamide-rituximab, or FC, fludarabine-cyclophosphamide), thus corroborating and extending earlier investigations.
Quantitative four-color MRD flow and ASO RQ IGH PCR are the current reference methods for sensitive and quantitative MRD assessments in CLL. Either of the two techniques should be applied for MRD monitoring. Novel technologies for MRD quantification (eg, next generation sequencing, eight-color MRD flow) have to be thoroughly evaluated on clinical samples against these standards before clinical application.
MRD predicts PFS
The prognostic significance of MRD is usually explained by an inverse correlation of tumor load after therapy and PFS (see Fig. 1 A). That concept was inspired by data identifying clinical response or clinical complete remissions (CR) as predictors for longer time to progression. For instance, in the German CLL study group (GCLLSG) CLL8 trial 75.9% of patients achieving a CR remained progression-free at 3 years postrandomization compared with 45.2% patients achieving only a partial response (PR) or nonresponse ( P <.0001). One can therefore conclude that residual tumor burden when assessed by the insensitive method, clinical staging, predicts PFS and hypothesize that this will be more accurately possible using MRD.
A survey of major investigations reporting a correlation between MRD and clinical outcome revealed data on 1328 patients with CLL included into 17 series or trials (see Table 2 ; Table 3 ). All those patients received induction therapy, but neither allogeneic transplants nor maintenance therapies.
