Fig. 1.1
Incidence by histology of thyroid cancer and mortality of thyroid cancer, 1975–2011. Data are from the Surveillance, Epidemiology, and End Results (SEER) program (www.seer.cancer.gov) SEER*Stat Database: Incidence—SEER 9 Regs Research Data, Nov 2012 Sub (1973–2011) 〈Katrina/Rita Population Adjustment〉—Linked To County Attributes—Total US, 1969–2011 Counties, National Cancer Institute, DCCPS, Surveillance Research Program, Surveillance Systems Branch, released April 2014, based on the November 2013 submission. Underlying mortality data for 1975–2010 are provided by NCHS (www.cdc.gov/nchs)
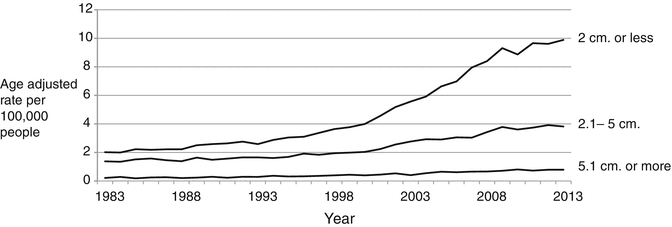
Fig. 1.2
Differentiated thyroid cancer incidence trends by size, 1983–2011. Data are from the Surveillance, Epidemiology, and End Results (SEER) program (www.seer.cancer.gov) SEER*Stat Database: Incidence—SEER 9 Regs Research Data, Nov 2012 Sub (1973–2011) 〈Katrina/Rita Population Adjustment〉—Linked To County Attributes—Total US, 1969–2011 Counties, National Cancer Institute, DCCPS, Surveillance Research Program, Surveillance Systems Branch, released April 2014, based on the November 2013 submission
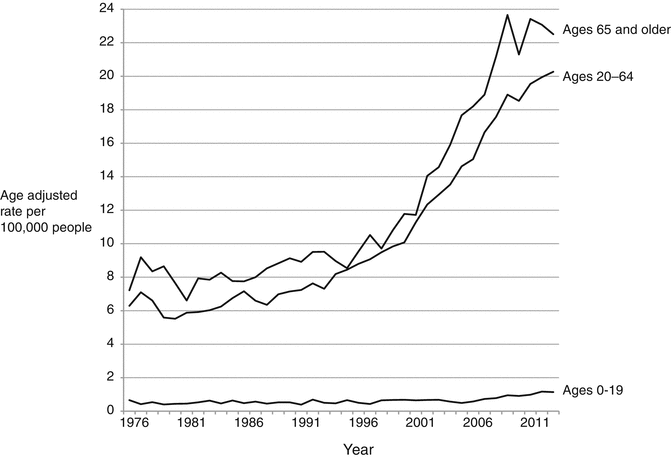
Fig. 1.3
Thyroid cancer incidence trends by age group, 1975–2011. Data are from the Surveillance, Epidemiology, and End Results (SEER) program (www.seer.cancer.gov) SEER*Stat Database: Incidence—SEER 9 Regs Research Data, Nov 2012 Sub (1973–2011) 〈Katrina/Rita Population Adjustment〉—Linked To County Attributes—Total US, 1969–2011 Counties, National Cancer Institute, DCCPS, Surveillance Research Program, Surveillance Systems Branch, released April 2014, based on the November 2013 submission
Mortality from thyroid cancer is stable, hovering around 0.5 per 100,000 people (Fig. 1.1). It does fluctuate: between 1975 and 2010, the highest was recorded in 1977 at 0.57 per 100,000 people (approximately 1,710 deaths per year nationwide), and the lowest was recorded in 1994 at 0.42 per 100,000 people (approximately 1,260 deaths per year nationwide). The annual rate of thyroid cancer mortality in the USA has not varied outside the range observed for the past 30 years. Recently, statistical tests have shown a very small 0.9% increase in the most recent years of data, but the clinical relevance of this increase is not yet clear and is under investigation.
Increased Detection of Subclinical Disease
A great proportion of the dramatic increase in the incidence of thyroid cancer over the past two decades may be due to detection of tumors that are subclinical. There is a large reservoir of thyroid cancer for which the value of treatment is uncertain, since papillary thyroid cancer is commonly found at autopsy in people who died of other causes [9–12]. These tumors are referred to as subclinical disease, and this phenomenon is termed “overdiagnosis” in cancer epidemiology. Overall a number of changes in healthcare practice have led to the increased detection of thyroid cancer through increased imaging, more frequent biopsy and surgery, and more thorough analysis of tissue by pathologists. These healthcare practices are reviewed in Table 1.1 .
Table 1.1
Potential healthcare practices contributing to the increasing incidence of thyroid cancer
Contributor | References |
---|---|
Healthcare system access | |
Increased use of imaging tests | |
Changing thresholds to work up thyroid nodules | |
Increase in number and extent of surgery | |
Increased scrutiny of pathology specimens |
One key piece of evidence for overdiagnosis is that the majority of increasingly detected thyroid cancers are small tumors. Small cancers are typically asymptomatic at discovery and are detected within the size range commonly found in the autopsy studies described above. The detection of early stage thyroid cancer has rapidly outpaced the detection of late stage disease for the past three decades in the SEER database [13]. Larger and more advanced (node-positive) tumors have also increased in incidence [7, 14, 15]. This may also be due to changes in healthcare practice as even 40% of tumors larger than 4 cm may be discovered incidentally on imaging. Improvements in technology also frequently upstage disease by detecting what would be previously occult lymph nodes [16, 17]. A meta-analysis of 35 separate autopsy studies since 1949 has shown that differentiated thyroid cancer is common and has been identified at a steady rate of around 11% when whole specimens are evaluated, with no time trend observed [18]. This strongly suggests that the increasing incidence of thyroid cancer is due to diagnostic detection and does not reflect population-level increases in tumorigenesis.
Subclinical disease may be more frequently detected as imaging technology is more frequently utilized. High-resolution ultrasound has become commonplace in the evaluation of thyroid nodules and detects five times the number of lesions detected by palpation [19]. Access to healthcare services correlates with thyroid cancer incidence rates [20–22] as access makes it more likely that a subclinical cancer is found. Private funding of healthcare is also significantly associated with thyroid cancer incidence [23]. Even when patients with a history of disease are followed longitudinally, the SEER database has shown that increases in ultrasound and PET scans increase the likelihood of undergoing additional therapy with no effect on mortality [24]. Iodine 131 is associated with improved disease-specific survival in this study, probably because it is used to detect advanced disease amenable to radioactive iodine therapy.
One particularly studied example of the interplay between healthcare delivery systems, advanced imaging, economic incentives, and thyroid cancer is South Korea. Between 1996 and 2010, the age-adjusted incidence of thyroid cancer in some South Korean provinces increased dramatically, with a notable tenfold increase from 10.6 to 111.3 per 100,000 women. During this time, thyroid cancer mortality remained unchanged, while asymptomatic cancers increased from less than 5% to more than 50% of all cancers [25]. This coincided with a rapid increase in ultrasound screening for thyroid nodules. A 2009 survey showed 13% of South Korean adults had undergone a screening thyroid ultrasound, with the highest rate among middle-aged women [26]. At this time a number of hospitals and providers were offering ultrasound screening as a $30–50 “add-on” to a nationally approved, government-subsidized screening program for other cancers. Previous public health campaigns for early detection of cancer in South Korea had been vigorous, and later media attention to the “overdiagnosis” of thyroid cancer caused national controversy [27]. Since intense media coverage of this issue in 2014, there has been a 30% decrease in the incidence of thyroid cancer based on insurance data and a 35% reduction in the number of thyroid operations in South Korea [28].
Subclinical disease may also be increasingly reported due to an increase in diagnosis from sampled tissues. Among thyroid specimens removed for benign disease, up to 50% harbor small, incidental papillary thyroid cancers [29]. Over the past several decades, guidelines have shifted toward recommending biopsy for smaller nodules [30]. This trend has only recently reversed as investigations have shown that lesions less than 1 cm are low risk and should not be routinely biopsied [30]. Similarly, recommendations for thyroid surgery for small papillary cancers historically trended toward performing total thyroidectomy instead of lobectomy. Hemithyroidectomy has recently returned to consideration in the American Thyroid Association guidelines for tumors up to 4 cm [30, 31]. More frequent biopsies and larger specimens provide more opportunities for pathologists to find cancer, thus it is likely that these two phenomena are contributing to the increasing incidence of thyroid cancer. Thyroidectomy volumes have increased in parallel with increases in imaging rates and physician behavior. A study using the population-based Nationwide Inpatient Sample and National Survey of Ambulatory Surgery databases estimated that annual thyroid surgery volumes increased 39% over 10 years, from 66,864 in 1996 to 92,931 in 2006 [32]. Resident case logs show similar results, even doubling for otolaryngology trainees over a few decades [33]. The evaluation of greater volumes of tissue through both biopsy and surgery contributes to increased diagnosis of thyroid cancer.
Evaluation of thyroid specimens by pathologists has also changed with time. Suggested descriptive areas have increased from 5 in 1982 to a minimum of 14 in 2012, suggesting that each thyroid specimen is examined much more closely compared to the past [34, 35]. Individual institutions have also reported increased scrutiny of specimens over time [36]. An increase in the total diagnosis of cancer has also occurred as a shift toward labeling cases “follicular variant of papillary carcinoma” rather than follicular adenoma [37, 38]. This phenomenon has been investigated, and the very low risk of adverse outcomes associated with this diagnosis has led to a reclassification of this variant to “noninvasive follicular thyroid neoplasm with papillary-like nuclear features” and thus not a true invasive cancer [39]. The phenomena described in this section clearly compound on each other. Over the past several decades, thyroid lesions are increasingly more likely to be identified by imaging; identified lesions are more likely to undergo biopsy or surgical removal, and specimens are more thoroughly evaluated for cancer.
Biologic Risk Factors for Thyroid Cancer
The biologic development of thyroid cancer is still incompletely understood, and the strong effects of healthcare system practices on detection of thyroid cancer complicate epidemiologic investigations. It is commonly thought that cancer develops as a result of genetic mutation. Thyroid cancer has one of the lowest rates of underlying mutations [40]. Recent genetic characterization of papillary thyroid cancer has now identified driver mutations for over 96% of cases [41]. Ionizing radiation causes DNA double-strand breaks that often lead to somatic mutations that drive the development of thyroid cancer [42, 43]. Given the indolent course of differentiated thyroid cancer, it is expected that exposure to radiation is most important in early childhood and epidemiologic studies substantiate this expectation. As most thyroid cancers remain small, even until the point of death by other causes, additional risk factors may play a role in the progression of thyroid tumors to clinical significance. Thyroid-stimulating hormone is well known to stimulate the growth of thyroid cancer, and suppression of TSH is an established practice in thyroid cancer patients [44]. A number of proposed risk factors for thyroid cancer include obesity, nutrition, underlying endocrine disease, and smoking. All may theoretically influence the hormonal milieu that enables thyroid cancer to progress. These studies are typically observational and highly complicated by difficulty controlling for the influence of healthcare practices described above. Biologic contributors to the incidence of thyroid cancer are summarized in Table 1.2.
Table 1.2
Potential biologic factors that may cause thyroid cancer and potentially contribute to the increasing incidence of thyroid cancer
Contributor | References |
---|---|
Radiation exposure | |
Obesity and diabetes | |
Estrogen | |
Iodine insufficiency or excess | |
Dietary nitrates | |
Autoimmune thyroid disease | |
Smoking |
Ionizing Radiation as a Risk Factor for Thyroid Cancer
Exposure to ionizing radiation is the most extensively studied and clearly defined risk factor for thyroid cancer. Radiation exposure mainly increases the risk of thyroid cancer for exposures during childhood, especially for those younger than 5 years old [45]. Much of the understanding of these exposures comes from studies of survivors of catastrophic nuclear events [46–49]. In a cohort of just over 45 thousand survivors of Hiroshima and Nagasaki under the age of 20 at the time of the bombings, 191 cases of thyroid cancer have been reported, compared to 154 expected by population-matched controls (HR 1.28 per Gy of radiation exposure). Most of these cases however occurred once survivors were over the age of 40. Adults at the time of the atomic bombings have statistically similar rates of thyroid cancer to unexposed individuals [48]. The radiation effect is clearly a biologically established effect as thyroid cancer is also increasingly identified at autopsy in nuclear bomb survivors [50]. The effects of radiation influence the entire continuum of thyroid neoplasms with a dose-response effect established for benign thyroid nodules and cysts as well as malignant disease [51].
The carcinogenic effects of the Chernobyl power plant disaster have also been well studied. A large increase in the incidence of thyroid cancer was observed for two decades following the disaster, with a number of characteristic mutations attributed to radiation identified [52]. Longitudinal studies of Eastern Europeans exposed to the Chernobyl nuclear accident suggest an additional 4/100,000 male cases and 16/100,000 female cases of thyroid cancer in exposed individuals [53]. Age at exposure to the power plant catastrophe is the most notable modifier of thyroid cancer risk, similar to the Japanese bombings. A slight increase risk for adults exposed to radiation has been reported, but this may also be confounded by aggressive postexposure screening.
Patients undergoing radiation treatment also present the opportunity to understand the effects of radiation dose and age at exposure on the development of thyroid cancer. Pooled analysis of pediatric patients undergoing radiation therapy suggests a linear relationship between the risk of thyroid cancer and radiation dose up to 10 Gy with this effect plateauing from 20 to 30 Gy [54]. This dose-effect curve is also related to age at exposure with the steepest relationship between dose and relative risk in the youngest patients (β = 9.17 for patients <1 year old vs. β = 1.51 for patients 15–19 years old). Lower dose radiation below 1 Gy is less certain to have any effect on thyroid cancer. In a Swedish cancer registry including patients undergoing radiation for benign spinal conditions, sub-gray doses of radiation were only associated with increased thyroid cancer when delivered directly to the cervical region [55]. This represented about eight additional cases in an exposed population of 8000, and most cases were diagnosed >15 years after exposure.
Changes in exposure to ionizing radiation are responsible for a small proportional increase in the overall thyroid cancer incidence at most. Annual radiation exposure for people who do not experience the exceedingly rare events of a nuclear disaster or radiation treatment for cancer has increased from 3.6 to 6.2 mSv since the 1980s. This dose is roughly equivalent to the same values in mGy, 1000 to 10,000 fold less than the exposures described in the previous paragraph. This increase is nearly entirely attributable to medical imaging, predominantly due to the use of CT scanning [56]. In the USA, the number of CT scans performed annually has increased over tenfold in the past several decades [57], but most CT scans are performed in patients over 55 years of age [45]. Multiplying CT utilization numbers [58] by mean excess lifetime thyroid cancer risk per scan [45] suggests that 150–350 additional thyroid cancers annually are attributable to pediatric CT scans in the USA. Adult exposure to ionizing radiation through background or occupational exposure remains uncertain, with a few studies reporting an association [59–62]. These studies typically do not control for healthcare utilization or report highly attenuated results when doing so.
Obesity, Estrogen, and the Metabolic Syndrome
Obesity has rapidly increased during this same period of rising thyroid cancer, and the metabolic syndrome has had a pervasive effect on innumerable aspects of healthcare [63]. Data are conflicting over the relationship between diabetes, obesity, and thyroid cancer [64]. Numerous studies have demonstrated a correlation between body mass index (BMI)-defined obesity and thyroid cancer with a hazard ratio ranging from 1.09 to 1.5 depending on the BMI cutoff value [65, 66]. Proposed mechanisms include exposure to adipokines such as leptin and adiponectin or thyroid-stimulating hormone and estrogens, insulin resistance and the insulin-IGF-1 axis, increased aromatase activity, chronic inflammation, and oxidative stress [67]. Leptin, a neuroendocrine regulator, stimulates thyroid cancer cell line proliferation through phosphatidylinositol 3-kinase/Akt signaling [68, 69]. A number of small cohort studies have suggested increased levels of leptin [70, 71] and decreased levels of adiponectin [72] in patients with thyroid cancer. Excess weight and BMI has been associated with larger and more aggressive thyroid tumors [73, 74]. However a causal relationship between obesity and thyroid cancer has not been proven. There may even be a common factor between genetically determined body size and thyroid cancer risk as adult thyroid cancer risk was associated with both childhood BMI and height in a large Danish population study [75]. A study of obesity-related genetic polymorphisms did not demonstrate an association with thyroid cancer risk [76]. The association between obesity and thyroid cancer may also be due to increased medical attention given to obese populations and thus a higher rate of detection [77]. More prospective studies and studies designed to assess causality would be needed to establish a relationship between the risk of thyroid cancer and obesity-related markers of thyroid function.
A slight positive correlation exists between type 2 diabetes and thyroid cancer [64, 78]. Beyond the mechanisms described previously for BMI, elevated insulin, long-term exposure to high levels of glucose or triglycerides, and use of antidiabetic medications have been proposed [79]. Insulin is homologous in structure to insulin-like growth factor-1 (IGF-1), which drives follicular cell proliferation in some animal studies [80]. Overall cancer mortality in diabetics using insulin is twice that of diabetics using metformin, but this has not been specifically studied in thyroid cancer [81]. Hyperglycemia has been associated with increased incidence and mortality of numerous malignancies including thyroid cancer in a large European population study [82]. Associations between diabetes and thyroid cancer may also be due to screening bias rather than a true biologic cause, as there is a positive association between diabetes and the likelihood of undergoing thyroid ultrasound and thyroid fine-needle aspiration [83]. As with obesity, more work is necessary to establish any direct causal mechanism between diabetes and thyroid cancer.
Estrogen has historically been proposed as a mechanistic cause of thyroid cancer, given the higher prevalence of thyroid cancer among women. It is interesting that a number of the previously described studies of obesity have shown a stronger association between obesity and thyroid cancer in men [65, 66, 81, 84] noting that abdominal fat causes excess estrogen through the aromatization of androgens [85]. Research supporting a direct hormonal cause of thyroid cancer is predominantly limited to cell line studies. Laboratory studies using thyroid cancer cell lines show cancer cell proliferation is enhanced by estrogen stimulation through various mechanisms including modulation of ER-α, ER-β, cyclin D1, GPR30 activity, and MAPK activity [86–89]. Clinical studies linking estrogen to thyroid cancer are less compelling. One case control study showed higher estrogen-DNA adducts in the urine of women with thyroid cancer [90]. Another study showed thyroid cancers with ER-α-positive, ER-β-negative, and positive androgen receptor expression were associated with a more aggressive disease course [91]. In one meta-analysis, factors related to endogenous estrogen exposure including parity, breastfeeding, and age at menopause moderately correlate with thyroid cancer risk, but exogenous exposures such as oral contraceptive use and estrogen replacement therapy do not [92]. More clinical and epidemiologic work is necessary to support estrogen as a cause of the increasing incidence of thyroid cancer.
Other Proposed Modulators of Thyroid-Stimulating Hormone and Thyroid Cancer Risk
Iodine deficiency and excess is known to affect the proportions of thyroid cancer histologies [93, 94]. Animal studies support iodine deficiency as a risk factor for thyroid cancer [95], but data in humans are lacking. Two epidemiologic studies comparing iodine-sufficient and iodine-insufficient areas have shown opposite effects of iodine intake on thyroid cancer rates [93, 96]. Iodine supplementation is difficult to study as this typically coincides with modernization and expansion of healthcare with greater use of imaging technology and detection [97–99]. Nitrates compete with the uptake of iodine by the thyroid, impairing thyroid hormone synthesis and thereby driving chronic elevation of thyroid-stimulating hormone. Dietary nitrate is a contaminant of drinking water in agricultural areas and is found at high levels in some vegetables such as beets. Epidemiologic studies linking dietary nitrate to thyroid cancer risk show mixed results, failing to establish a dose-dependent response, and can also be confounded by associated changes in screening [100–103]. A recent systemic review and meta-analysis has concluded that no association between dietary nitrate exposure, thyroid hormone function, and thyroid cancer exists [104]. Overall, a clinically relevant and directly causal relationship between diet and thyroid cancer remains to be proven.
Autoimmune thyroid disease has been proposed as a cause of thyroid cancer. Elevated levels of TSH are found in hypothyroid patients with autoimmune thyroid disease and may stimulate follicular epithelial proliferation, promoting the development of papillary carcinoma. Studies examining FNA biopsy for patients with autoimmune thyroid disease have not shown a statistically significant correlation between Hashimoto’s thyroiditis and papillary thyroid cancer [105, 106]. Retrospective studies of patients with autoimmune thyroid disease undergoing thyroidectomy typically report a statistically significant positive correlation [107–109]. This study design is highly flawed and confounded by indication as patients with autoimmune thyroiditis who have features worrisome for malignancy are highly more likely to elect thyroidectomy. More prospective studies with longer follow-up would be needed to test the relationship between autoimmune thyroid disease and thyroid cancer.
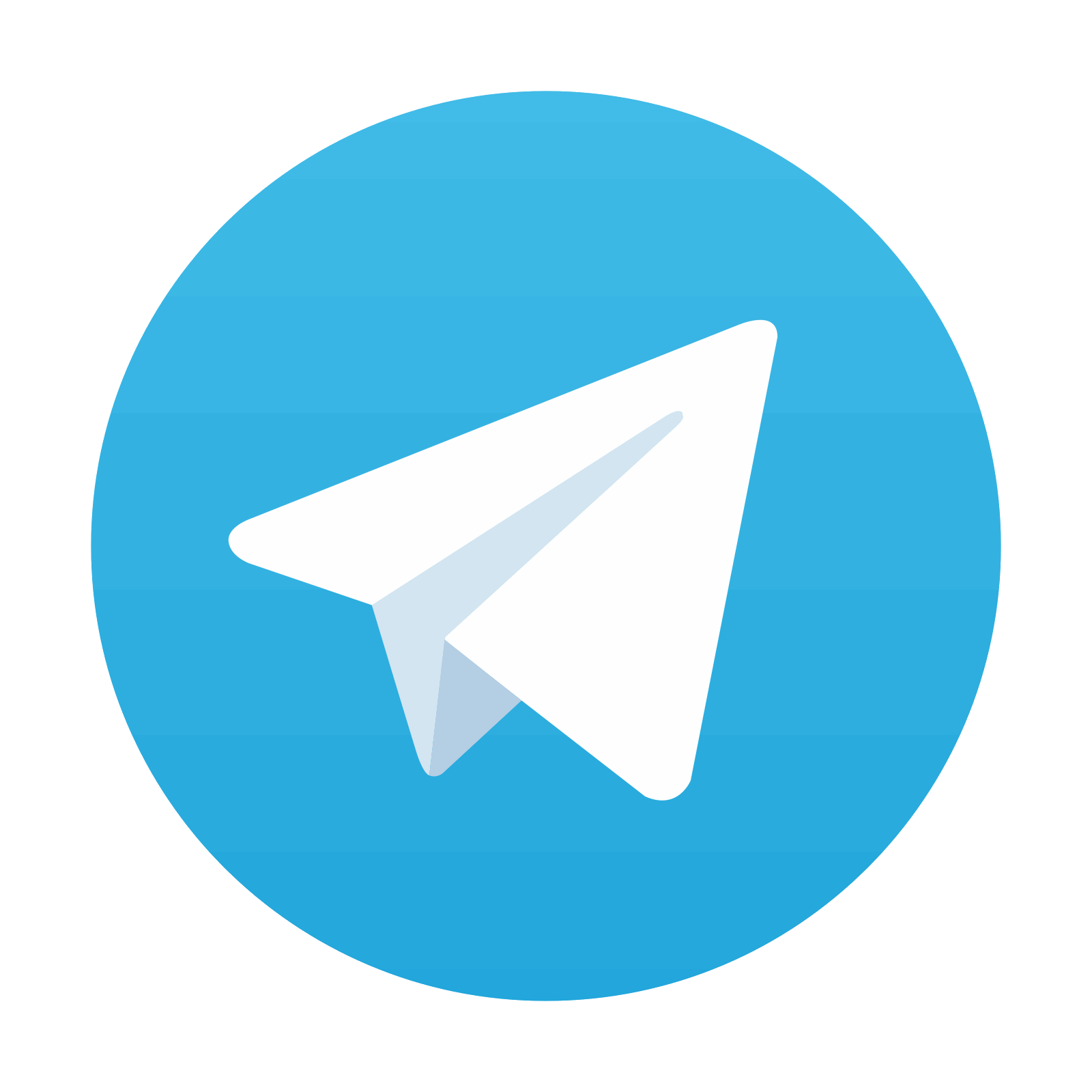
Stay updated, free articles. Join our Telegram channel

Full access? Get Clinical Tree
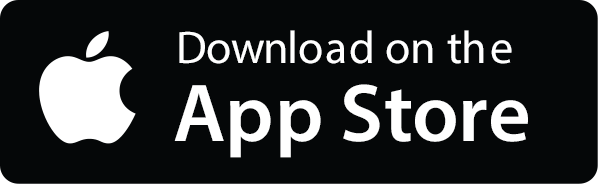
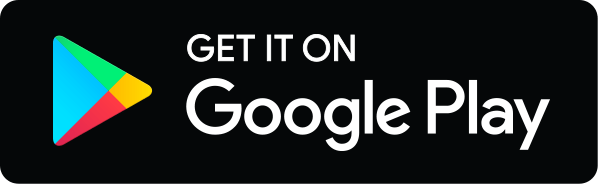