© Springer International Publishing AG 2017
Robert M. Hoffman (ed.)Patient-Derived Mouse Models of Cancer Molecular and Translational Medicinehttps://doi.org/10.1007/978-3-319-57424-0_22. The Revival of Patient-Derived Xenograft Mouse Models of Cancer: Way Back to the Future
(1)
AntiCancer, Inc., 7917 Ostrow Street, San Diego, CA 92111, USA
(2)
Department of Surgery, University of California, San Diego, CA, USA
Keywords
Nude micePatient tumorsSubcutaneousOrthotopicMetastasisTransgenicRygaard and Povlsen established the first patient tumor cancer nude-mouse model with a colon cancer surgical specimen. This was the first use of nude mice to grow a human tumor. During the 1970s and 1980s, there was much worldwide use of nude mice to grow both patient tumors and human cells lines. However, xenograft mouse models went out of fashion for almost 20 years after the introduction of “OncoMouse” in 1984, the first of a long line of transgenic mouse models of cancer. Halfway through the first decade of the present century, there was a revival of xenograft models that were basically the same as Rygaard and Povlsen’s subcutaneous tumor model of 1969, in which the majority of human solid tumors do not metastasize. Orthotopic implantation of tumors enabled metastasis to occur. Although orthotopic metastatic mouse tumor models were first described in 1982 and further developed to be able to mimic metastasis in the patient in 1991, the use of orthotopic model remains limited despite their far superiority to subcutaneous or genetically-engineered mouse models of cancer in current and previous use.
Before the use of the athymic nu/nu mouse (nude mouse) for the growth of human tumors in 1969, there was no systematic way to grow human patient tumors in mice. Rygaard and Povlsen [1] implanted tumors in mice from a colon cancer from a 74-year-old patient subcutaneously (s.c.) in nude mice, which grew similar to the donor patient. The tumors grew locally and did not metastasize over 70 passages [1, 2].
Throughout the 1970s and 1980s, many authors noted that despite the metastatic behavior of tumors in the patient, s.c.-transplanted xenografts in nude mice were benign. This is still the case of PDX models today [2–4].
Wang et al. [5] in 1982 transplanted a human colon cancer cell line suspension orthotopically (literally “correct surface”) in nude mice rather than “heterotopically” (literally “different surface,” such as s.c.). A suspension of colon cancer cells was injected within the descending part of the large bowel of nude mice, resulting in metastases as well as local tumor growth [2]. However, orthotopic implantation of cancer-cell-line suspensions usually resulted in a low frequency of metastasis [2].
Patient-derived orthotopic xenograft (PDOX) models, which were implanted using intact tumor tissue with the technique of surgical orthotopic implantation (SOI) [2, 6], were established from patients with colon [7–9], pancreatic [10–21], breast [22], ovarian [23], lung [24], and stomach cancer [25] and mesothelioma [26] in the early 1990s in our laboratory, resulting in primary and metastatic tumor growth very similar to that of the patient [25]. Recently, PDOX models of sarcoma [27–31] have been developed, cervical cancer [32–34] as well as melanoma [35, 36].
A clinical study of 20 patients having stomach cancers that grew orthotopically in nude mice after SOI showed direct correlation of the metastatic pattern of the patient and mice [25]. In another case, a patient-derived colon cancer lung metastasis grew in the lung, but not in the colon or skin of nude mice [37].
In the 1980s, the Leder group published their famous “OncoMouse” paper [38] describing a transgenic mouse in which the normal mouse Myc gene was driven by a hormonally inducible mouse mammary tumor virus promoter to generate spontaneous mammary adenocarcinomas [38]. OncoMouse started the era of transgenic mouse cancer models, which would dominate the cancer mouse model field for almost 25 years. The tumors in these models were usually driven by “oncogenes” constructed with super-active viral promoters. More sophisticated techniques were later developed to establish transgenic tumor mouse models, including homologous recombination and the use of a Cre–loxP system for activating “oncogenes” or deactivating (knocking out) “tumor suppressor” genes [2].
The transgenic mouse models of cancer were touted as the “real” mouse models of cancer, and at the same time xenograft models were roundly denigrated and “xenograft” became a taboo word. For example:
Sharpless and Depinho [39] described these two reciprocal phenomena:
Their paper starts out by blaming the lack of effective drugs for cancer on xenograft models:
“Most hold the view that the use of xenograft models in the cancer drug discovery and development process has proved to be problematic, with few predictive achievements and many notable failures.”
Sharpless and Depinho [39] describe the problem:
“Critics who comment on the failure of ‘mouse models’ are being dismissive of xenograft testing in particular” [39].
“This approach [xenograft] has notable flaws, but because of its ease and low cost it has been used extensively in academia and the pharmaceutical industry during the past three decades…” [39]
“The problem with xenograft analyses, however, is that many agents that show consistent and potent anticancer activity in specific xenograft models prove to be of limited use in the therapy of human cancer. This single fact is a major contributor to the low success rate of novel therapeutics when first tested in humans” [39].
“Third, and perhaps most significant, is the fact that these systems [xenograft] model cancer as if it was [sic] a disease of homogeneous rogue cells” [39].
“By failing to recapitulate the complex and evolving tumor-host stroma interactions, which could be further complicated by the immunodeficient state of the animal, xenograft analyses are reductionist and fall short of fully capturing the potent modulating effects of the tumor microenvironment in drug response” [39].
“…xenograft studies typically use only a few human tumor cell lines, the oncogenomic profiles of which represent only isolated combinations of the wide spectrum of genetic and epigenetic mutations that are resident in a given tumor type presented in the clinic” [39].
“As the specific genetic profile can alter a tumor’s response to a drug… the inability to predict the outcome of clinical trials probably results in part from a failure to represent the enormous genetic diversity of tumors in patients [by xenografts]” [39].
“…novel inhibitors of angiogenesis (endostatin and angiostatin) showed potent anticancer activity when given alone or in combination against a large variety of xenografted human and murine cell lines, but so far have not demonstrated single-agent activity in human cancers…” [39]
“…by the observation that most compounds entering human clinical testing fail because of lack of efficacy, despite showing promise in preclinical xenograft testing” [39].
These “criticisms” of xenograft models by Sharpless and Depinho were typical of what was said in published scientific papers and in scientific meetings by leaders of the transgenic mouse field. In major meetings on mouse models of cancer, xenograft presentations were discouraged or not allowed. The National Cancer Institute’s “Mouse Models of Human Cancer Program” was funding essentially only grant applications on transgenic mouse models of cancer. Thus, for approximately a quarter century, the great work of mouse xenograft models of cancer, especially human patient tumor xenografts (see later chapters in this book), was ignored or described as worthless and blamed for the failure to find effective drugs for cancer.
In 2006, a “way back to the future” event occurred, all the way back to 1969, Rygaard and Povlsen [1]. The s.c.-transplanted human patient xenograft mouse model was heavily promoted by Hidalgo et al. [40] and his company, Champion’s. At first, the term “tumorgraft” [41] was used so as not to use the taboo term “xenograft” [2].
The reborn s.c. models sometimes used more immunodeficient, such as nonobese diabetic, severe combined immunodeficiency (NOD-SCID) mice. However, the tumors were still s.c. and did not metastasize. In order not to seem to be going back to the 1960s, the born-again s.c.-transplanted mouse models were given even more exotic new names such as “xenopatients” or “avatars” [4] in order to exaggerate their capability and novelty [2]. The October 3, 2014 issue of Science had an “avatar” on the cover, which stated: “To make mice better mirrors of human cancer, researchers are building ‘avatars’ with the cancer of a particular patient…. The work marks a sea change in cancer biology and is stirring hope that new mouse models will pave the way to more personalized care” [42]. However, orthotopic patient models are hardly mentioned in the “xenopatient” and “avatar” papers [2, 4, 43]. Patient-derived xenografts, simply referred to as PDX models of cancer, are now the hot fad and “transgenic” cancer models appear to be in eclipse [2].
After 52 years in science, I have seen many scientific fads that come and go and come back again [2, 44]. We are now back to the late 1960s with the so-called patient-derived xenograft “PDX” model. Orthotopic models attained a modicum of popularity in the late 1980s and early 1990s [2], due in large part to the great efforts of Fidler [45]. It seems that most cancer researchers have either forgotten about or are unaware of orthotopic models [2], especially PDOX models, which are metastatic and resemble the patient’s tumors [2]. It is the goal of the present book to give a better appreciation of the history as well as state of the art of patient mouse models of cancer, in particular the patient-derived orthotopic xenograft (PDOX) models.
References
1.
Rygaard J, Povlsen CO. Heterotransplantation of a human malignant tumor to ‘nude’ mice. Acta Pathol Microbiol Scand. 1969;77:758–60.CrossrefPubMed
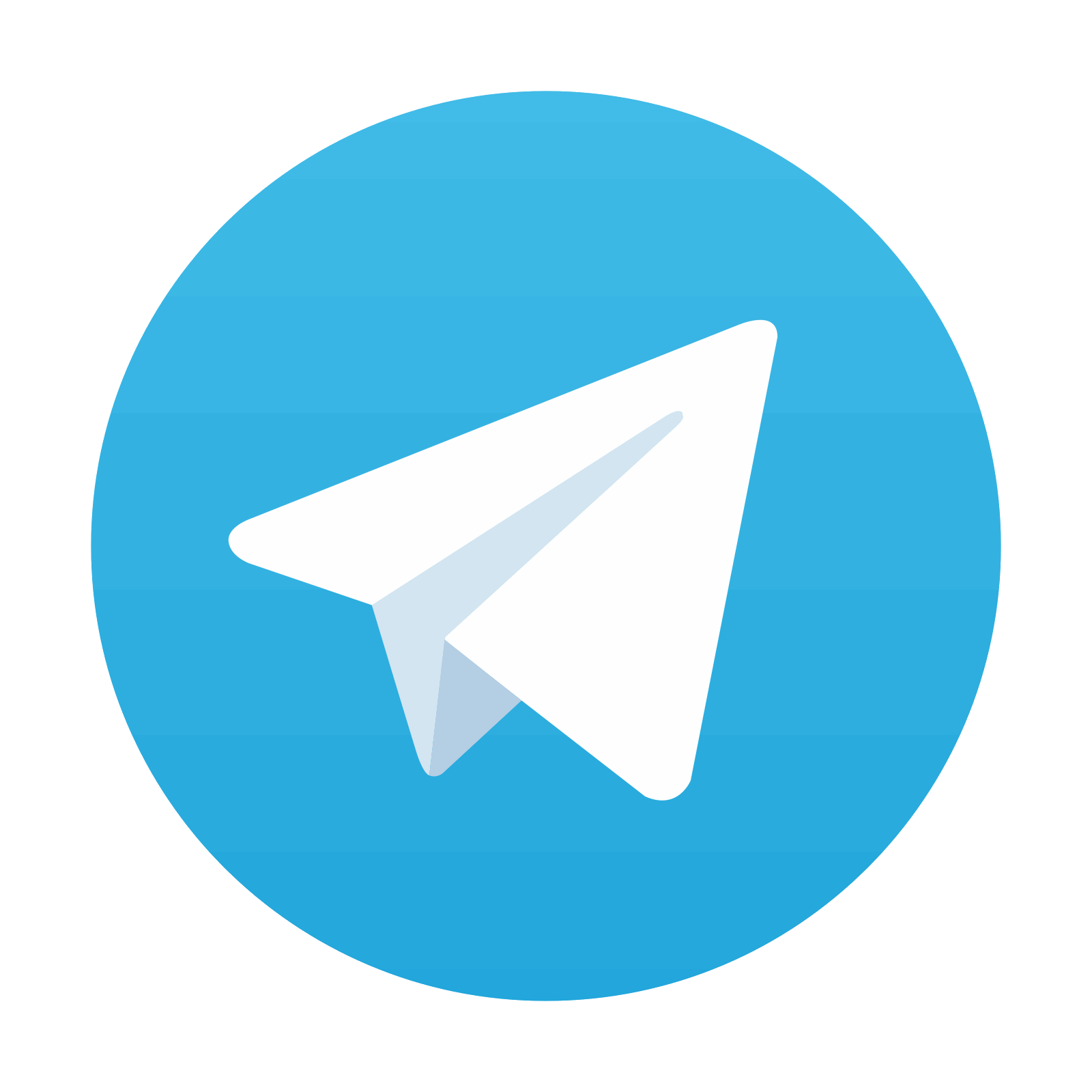
Stay updated, free articles. Join our Telegram channel

Full access? Get Clinical Tree
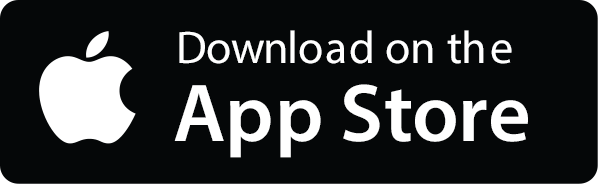
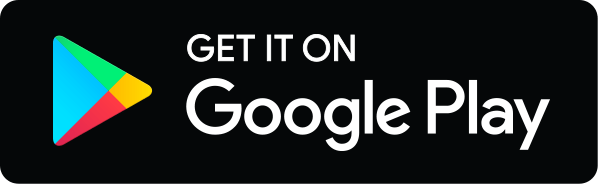
