Fig. 34.1
Tumor overlap map overlaid on a standard Montreal National Institute (MNI) T1 for all 198 patients, presented according to the neurological convention (left to left and right to right). The color range indicates the number of patients for whom the voxel is lesioned. Each brain section is presented with its z-coordinate in the MNI space
The third approach consists in the construction, by means of a novel probabilistic method, of a graph-based spatial position mapping [7, 11]. We applied this methodology in a consecutive series of 210 DLGG patients at diagnosis, and confirmed the symmetrical distribution of the tumors and the preferential location within frontal (33%), insular (37%) and temporal (18%) areas, with very few tumors located in the occipital and prefrontal lobes (Fig. 34.2).
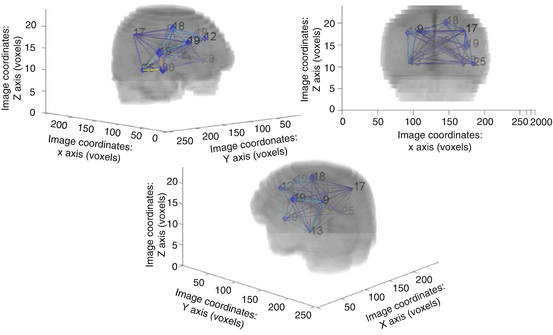
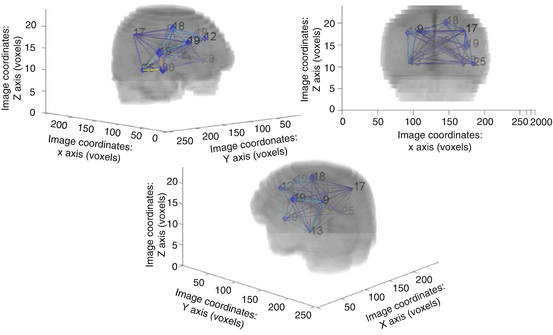
Fig. 34.2
3D-representation of the complete clustered graph superimposed to the mean registered image. The numbers of nodes in each cluster are reported
Whatever the methodology used to assess the preferential locations for DLGG, two main interesting findings should be highlighted.
On one hand, there are very few DLGG located in the posterior regions of the brain, including the occipital lobe. This finding was previously reported [6, 10]. It has been further confirmed by the works of our team, and leads to several biological hypotheses regarding DLGG genesis. First, the cytoarchitectony of the visual cortex is not the same, since the insula is constituted by a mesocortex, making a link between the allocortex and the neocortex [4]. Second, differences linked to developmental processes, including the myelination processes, could be involved. Indeed, it is interesting to observe that fronto-temporal areas are among the last myelinated areas during development, the myelination processes occurring until the second decade of life, particularly in the frontal lobe [12, 13]. In the study published by Paus et al. in 111 children and teenagers (4–17 years old), there was an age-dependent increase in white matter density in several areas including the posterior part of the arcuate fasciculus connecting the frontal and temporal areas and involved in language [13]. Interestingly, the myelination processes seem to occur earlier in the posterior regions of the brain (including the occipital lobe), which are only rarely affected by DLGG. It could thus be hypothesized that these temporal differences between regions myelinated at varied periods in the development could participate to the differences in terms of risk of DLGG according to the location. Finally, it seems possible that tumors of different locations could arise from different molecular pathways (“molecular hypothesis”). Indeed, differences in terms of molecular markers, including the mutation of the IDH gene and the 1p19q codeletion, have been reported by several studies [8, 14–22], and further confirmed by a recent work by our team. We will review these data in the present chapter.
On the second hand, DLGG are preferentially located within the so-called “eloquent” areas, including the insula and the supplementary motor area (SMA), which are both functional interfaces between the limbic system (mesiotemporal structure and cingulum) and the temporal pole (for the insula) or the prefrontal cortex (for the SMA). On the contrary, the occipital lobe is not a “transitional” area, and it does not link the limbic system and the neocortex. Moreover, from a functional point of view, both the insula and the SMA play a role in the planning of movements and language [23, 24], while the occipital lobe is not involved in planning. Overall, it can thus be hypothesized that the risk of DLGG is linked, among other factors, to the eloquence of the area involved and that there may be an impact of the microenvironment on DLGG development (“functional theory”).
34.2.2 The Functional Theory
The functional theory relates to the impact of the microenvironment or stroma on the tumor development. This hypothesis may be considered to interpret the reasons for the preferential brain locations of DLGG, such as the paralimbic system—in comparison with their non-preferential brain locations, such as the occipital lobe. Indeed, it could be hypothesized that interactions between neurons and glia are different in the SMA and insula compared with those in the occipital lobe. Indeed, glial cells are known to play a role (1) in neuronal migration, which may explain the existence of migration disorders in some cortical epilepsy, including the extra-temporal epilepsy that often originates from the SMA and insula – but rarely from the occipital region; (2) in the regulation of synaptic transmission; (3) in the control of synapse numbers; and (4) in the energy metabolism of the neuron, explaining the neurovascular and metabolic decoupling in gliomas. Therefore, if we consider that the insula/SMA and the occipital lobe have different structural and functional profiles, repercussions concerning the biology of the local glial cells are likely.
Arguments for the influence of functional parameters and of the subject’s activities on the glial cells can be found in the literature on training-induced macroscopic structural changes (of both white and grey matter) in human and animals. A number of neuroimaging studies in healthy volunteers showed that learning could generate a significant increase of gray matter volume in areas specifically involved in tasks extensively repeated [25]. The microscopic mechanisms involved will be discussed farther in this chapter. Interestingly, an implication of the glial cells, either direct (i.e., proliferation of the glial cells [26, 27]) of indirect (i.e., synaptogenesis [28] or myelination [29]) has been suggested. Thus, we might suggest that such modifications in the local glial properties may favor or prevent DLGG development in some specific brain locations.
First, we will shortly review data from the literature regarding training-induced changes in human and animal. Early studies from the 60’s have suggested a link between the environmental demands and the brain structure in animals [30, 31]. In the past 15 years, a number of in vivo studies in human have been performed thanks to new imaging techniques, mostly magnetic resonance imaging (MRI) [25]. With these techniques, both the grey (“voxel-based morphometry” or VBM based on a 3D and T1-weighted sequence [32]) and the white matter (“diffusion-tensor imaging » or DTI) were explored. Beyond these morphological parameters, the changes in the functional connectivity induced by training have also been studied using “resting-state” methods, as it can be modulated by the expertise of the subject for a task (see for example the study by Fauvel et al.: modulation of the functional connectivity at rest between several brain areas in 16 musicians compared to 17 matched non-musician controls [33]).
Using these techniques, the macroscopic changes induced by various tasks or activities have been studied in human, from navigation skills in London licensed taxi-drivers [34–36] to cognitive training tasks in students [37] or visuo-spatial tasks with varying complexity (i.e., juggling [25, 38]). These macroscopic changes constitute a powerful phenomenon. They have been reported for a high number of tasks (motor tasks [25, 38–42], pure visual or visuospatial tasks [43], spatial memory and navigation [34, 36], memory tasks [44–46], linguistic tasks [47, 48], calculation [49, 50], reasoning tasks [51], creative and “artistic” tasks [52, 53], and music [54]); from simple tasks [42] or more complex tasks (visuo-motor tasks such a juggling, golf, dance…); on healthy brain (most studies are performed on healthy voluntary subjects) or on damaged brain (i.e., after stroke [55] or in multiple sclerosis patients [56]); in young subjects [25] (including children [57]) or elderly subjects [39, 58]); with controlled tasks or in “ecological” conditions (uncontrolled leisure activities [58] or cognitive tasks [45]); in the long term (i.e., London licensed taxi drivers [34–36] or in the short term [25, 59–61]); for over-training or for suppression of a task (the withdrawal of the task, even short, is associated with a focal reduction of the grey matter in the corresponding areas [42]); and for both the grey and white matter. However, this phenomenon also seems reversible, with a possible regression of the macroscopic changes after a period of training withdrawal [25], even though a more persistent effect is possible [38, 44]. Therefore, if the hypothesis of an impact of the subject’s activities on the genesis of DLGG is true, it can only concern regular activities, such as professional activities, but probably not activities performed punctually. Moreover, it is not completely clear yet whether the morphological changes are linked to a skill itself, or to the fact of learning a new skill. Indeed, correlations between the intensity of the macroscopic changes in MRI and the subject’s performance at the task are inconsistently found [25, 43]. Moreover, some studies have reported a regression of the morphological changes without concomitant decrease in the subject’s performance at the task [25, 43], suggesting that these macroscopic changes reflect the fact of learning more than the skill itself. This would explain that, in human, there is no significant modification of the brain volume in relation with developing new skills or performing a higher number of activities. However, some discordant data have been reported in animals, among which an increased brain volume and weight in rats placed in an enriched environment [30]. In human, the correlations between the subject’s activities (including leisure activities) and brain volume have also been studied in non-demented healthy subjects. In 16 healthy elderly subjects, the grey and white matter volumes were correlated with a composite score considering the education level, activities and leisure [62]. In a larger study in 331 non-demented elderly subjects, a significant association was found between the grey and white matter volumes and education, but not with the activity and leisure score [63]. Finally, if the macroscopic changes visible in MRI are in relation with the learning of a task rather than with the skill/the expertise itself, how could we explain the differences between expert groups and novices in cross-sectional studies? One hypothesis is that expert subjects constantly learn and improve their skills: new streets, new traffic rules etc. for London licensed taxi drivers, new dance steps or choreographies for dancers, for example, which is less true for novice subjects.
To date, the microscopic changes underlying the macroscopic changes induced by training are still uncertain [64]. These changes most probably not only affect one isolated area but rather the whole functional network involved in the task. Microscopically, they probably occur both in the grey and white matter. Microscopic data is lacking and most of it comes from animal studies [26, 64, 65]. Various mechanisms are currently suspected, many of them involving glial cells in a direct or indirect manner: neurogenesis [66, 67], gliogenesis [26, 30, 68], glial hypertrophy (possibly mediated by the interactions between astrocytes and neurons; a recent study on cerebellar cortex in mice suggested that the molecular and functional profiles of astrocytes are regulated by the neurons, through the “sonic hedgehog” pathway [69]) [27, 70, 71], and synaptogenesis (mediated by astrocytes) [70–74] for the grey matter changes and increased glial cells density, increased myelination (mediated by oligodendrocytes) [26, 75, 76], axonal sprouting (possibly involving NogoA which is mainly expressed by the oligodendrocytes [77]) [65, 67, 78] or vascular modifications for the white matter changes. Thus, glial cells seem involved in training-induced macroscopic changes, either directly (gliogenesis and glial hypertrophy) or indirectly, through the regulation of synapses, axonal sprouting or myelination. It can be hypothesized that these changes could impact DLGG genesis.
As DLGG have preferential locations in “functional” areas, and macroscopic white matter and grey matter changes, possibly mediated by glial cells, can occur after training at a task, it can be hypothesized that an over-solicitation of a functional network by a task or a specific environmental demand may impact DLGG genesis. This hypothesis was named the “functional theory”, on the basis of the eloquence of the cerebral regions involved in DLGG. In a recently published study, mice exposed to an enriched environment for over three weeks showed a higher resistance to glioma development after being brain-transplanted with glioma cell lines (glioma development in 73.5% of the transplanted mice in enriched environment group compared to 96.5% in the standard environment group), suggesting a link between glioma development and the amount of brain solicitations [79]. Mice submitted to an enriched environment also showed smaller tumor sizes and reduced proliferation rates in this study. In human, only few studies have investigated the links between the subject’s activities and the risk of glioma so far. A small number of studies have reported conflicting results regarding the correlation between the education level and the risk of glioma [80–82]. A Swedish population-based case-control study in 494 glioma patients (and 321 meningioma patients) and 955 controls reported no correlation between the risk of glioma and the education level [81]. In a North-American study, the education level of 600 controls was higher than that of 325 glioma patients (for example, advanced degree in 26% of controls and 18% of cases) [80]. However, a French case-control study including 122 glioma patients (43 DLGG) found that an education level considered as “moderate” was significantly associated with a decreased risk of glioma (OR = 0.35, IC95% 0.16–0.77) compared to subjects with no education [82]. As such association was not found in subjects with a “high” education level, this result must be considered with caution. In the same vein, a recent cohort study found an increased risk of glioma in highly educated people (≥3 years university education) compared to those with primary education [83]. In this study, men and women with an intermediate or high non-manual occupation had a significantly increased risk of glioma. Regarding DLGGs specifically, a hospital-based case-control study in 135 low-grade gliomas (but including gangliogliomas) found no association with education [84]. Finally in a pooled analysis of seven case-control studies including 617 WHO grade II and III oligodendrogliomas and oligoastrocytomas and 1260 controls, 16.2% of cases compared with 17.6% of controls had a higher education level [85].
To date, there is no demonstrated association between professional activities and the risk of DLGG or, more generally, of glioma. A few professions have been inconsistently associated with the risk of brain tumors: health professions [86, 87], electricity workers [88], agriculture workers [89], industrial workers in petrochemical refineries [90], rubber industries [91], polyvinyl chloride industries [88], chemical industries [87] or nuclear plants; and various other professions such as teachers [92], architects [92, 93], butchers [93], salesmen and waiters [89], servicemen [89]. However these associations are inconstantly found and must be considered with great caution as they could be due to chance only since many of these studies conducted multiple comparisons. Moreover, due to the lack of “functional” classification of the activities, these studies evaluated the professional expositions rather than the neurocognitive functions involved in the profession.
34.2.3 The Cellular and Molecular Hypotheses
It appears increasingly clear that glial tumors emerge from particular initiating cells (cells of origin) transformed by the activation of oncogenic signaling pathways resulting from acquired molecular alterations. This oncogenesis will unfold in a cellular microenvironment, called stroma, itself shaped by loco-regional characteristics that can differ from one brain region to another. The emergence of a tumor in a given area of the brain is then a complex phenomenon in which cellular and molecular phenomena are closely intertwined, so that it is difficult to dissociate the cellular, molecular and functional hypotheses on the origin of gliomas.
Currently available data in the literature on these complex issues present common characteristics: they result from studies conducted in transgenic mice with restricted expression of transgenes in particular cell lineages, and they relate mainly to high-grade gliomas. Understanding of the molecular and cellular events involved in the genesis of low-grade gliomas for now lacks lifelike animal model.
34.2.3.1 Cellular Hypothesis
The oligodendrocyte precursor cells (OPC) and neural stem cells (NSC) have been identified as possible cells of origin of gliomas. OPC represent the major cycle-related population of the adult normal brain, dispersed throughout the gray and white matter. Considering former publications [94–96], OPC seemed particularly involved in the origin of low-grade gliomas. This finding is in good accordance with clinical observations reported by Vergani et al., with a systematic involvement of cortex in a series of 43 DLGG suggesting a centripetal tumor growth [97]. To go further, Galvao et al. showed that OPCs might transform into malignant glial cells by a two-step process. An initial step of malignant transformation through inactivation of TP53 and NF1 genes is followed by a reactivation involving mTOR pathway [98].
Other studies show that, in experimentally raised gliomas, there is interplay between the nature of the cell of origin and the triggered oncogenic signaling pathways. Each of these two elements involved in malignant transformation exerts its influence and the type of glioma thus obtained is the result of their combination.
To find out whether adult lineage-restricted progenitors of central nervous system cells are possible initiating cells of glioblastomas, Alcantara Llaguno et al. induced inactivation of Nf1, Pten and TP53 genes in neural progenitor cells (NPC) and OPC. They obtained two different types of glioblastomas. These tumors were different with regard to their molecular profile, their location in the brain and survival duration of mice developing these tumors. Each type was related to a different cell of origin i.e. NPC or OPC. This work demonstrated that the same oncogenic sequence could give rise to a different tumor type depending on the cell type in which it exerts its action. However experimental tumors thus obtained were histologically classified as glioblastomas, in both cases [99].
On the other hand, in a recent study, Lindberg et al. showed that, depending on the oncogenic pathways activated in the OPCs, a tumor of oligodendroglial or astrocytic type would arise. Overexpression of PDGF-B produced tumors similar to human grade II and grade III oligodendrogliomas while activation of the K-RAS/AKT pathway led to grade III and IV astrocytic tumors. The main finding provided by this work is that the importance of the cells of origin seems inferior to genetic aberrations in determining tumor histopathology [100].
An additional level of complexity is added by the high specialization of the different brain areas. This underlies intrinsic variations, within a given cell type, related to the location, independently to the cell lineage which it belongs. Several studies, once again conducted in mice, showed the diversity linked to the location within a highly compartmentalized organ as the brain. Ko et al. reported distinct gene spatial expression patterns in neurons, astrocytes and oligodendrocytes when analyzed from different brain areas. Gene expression patterns showed strong mirror symmetry between the left and the right hemispheres. Astrocyte and oligodendrocyte-specific transcripts displayed spatially clustered expression patterns less numerous and with less precise boundaries than for neurons [101].
This regional molecular variability in different cell types also affects functionally. First, in normal adult mouse brain, transplanted OPCs differentiated more or less rapidly into myelinating oligodendrocytes depending on whether they were derived from the white-matter or the gray-matter [102]. Second, in an animal model of glioblastoma, the oncogenic induction gives tumors whose growth rate is different depending on the subtype of astrocytes in which oncogenic stimulation was performed. Astrocytes expressing the glutamate aspartate transporter (GLAST) gave rise to more slowly growing tumors than astrocytes expressing glial fibrillary acidic protein (GFAP) [103].
Beyond the nature of the cell of origin and of the oncogenic pathways, the tumor microenvironment, is also involved in tumor development, particularly in the case of DLGG experiencing slow growth. It is the third component in this already complex interactions game. Immune cells represent one of the major components of the tumor microenvironment. Indeed, the immune system is involved in immune tolerance allowing the tumor to settle and then to progress. The immune cells present within the tumor are microglia, resident macrophages of the central nervous system, but also macrophages from peripheral blood that infiltrate secondarily the tumor (tumor associated macrophages TAM). All together, microglia and blood macrophages represent 30–50 % of the infiltrating cells in gliomas. Accumulating evidence indicates that microglia and TAM promote glioma growth and invasion [104]. At this level too, the diversity encountered between different brain areas is manifested: it has been known for a long time that the distribution and morphology of microglial cells varies from region to region of the brain [105].
There is a link between the immune microenvironment of the tumor and function. Garafolo et al. used the xenograft of glioblastoma cell lines to show that the enriched environment influences tumor development in mice. Indeed, the enriched environment induced the increase of two factors in the tumor microenvironment. The first of them is IL15 that causes accumulation of natural killer (NK) cells in the tumor. NK cells have a direct anti-tumor activity. The second is the important mediator Brain-derived neurotrophic factor (BDNF) that reduced macrophage infiltration and induced a reduction in tumor size [79].
34.2.3.2 Molecular Hypothesis
Once the onset of the tumor is achieved, other molecular events are going to take over and allow tumor development. As a logical consequence of the influences of the original cell, both by its nature and its location, and of the activated oncogenic pathway, molecular profiles determined on resected tumors vary from one region to another.
During the past fifteen years, a number of anatomo-molecular studies showed significant correlations between the location of DLGG and the tumor molecular patterns. First, the IDH mutation, considered as an early key event in the genesis of DLGG, is found with a frequency that varies according to the tumor location. DLGG with IDH mutation are more frequently found in the anterior part of the brain, especially in the frontal lobe in several studies in limited series often mixing DLGG and high-grade gliomas [19–22]. In a recent work from our team (Darlix et al., unpublished data) in a homogeneous and consecutive series of 198 DLGG patients, we confirmed that frontal tumors are more frequently IDH mutant compared to temporo-insular tumors (87.1% vs 57.5%, p < 0.001). Second, 1p19q loss, another molecular hallmark of DLGG, has also a different distribution depending on the location. Similarly co-deleted 1p19q gliomas were found more significantly in the anterior part of the brain, especially in the frontal lobe [8, 14–16, 20, 21]. This result has also been confirmed in our recent work, with the presence of a 1p19q codeletion in 45.2% of frontal DLGG compared to 17.0% of temporo-insular DLGG (p = 0.003). However, the anatomo-molecular studies published so far have some limitations. Most importantly, their methodology for determining groups of tumors according to their locations often relied on a subjective assessment of lobar anatomy, while it is needed to use different approaches to deal with the question of multi-lobar tumors. In addition, the majority of these studies were conducted on series including gliomas of different grades [8, 20] or a small number of homogeneous tumors [17–19].
The molecular regional differences yet observed in DLGG would have consequences on the outcome of these tumors because prognosis value has been assigned to some of them, especially IDH mutation and 1p19q loss. Therefore, the tumor location could be in itself a prognostic factor.
34.3 The Environmental Theory
Beyond their preferential locations within the brain, DLGG also seem to present with a heterogeneous distribution at the geographical level [5]. Indeed, even though the epidemiology of DLGG is poorly known, a few studies have previously suggested such an heterogeneity in the geographical distribution of gliomas, at the worldwide or European level, with a trend towards higher incidence rates in highly-developed countries (http://globocan.iarc.fr/) [106–109]. However these studies have major limits. First, they included gliomas of all grades and even different histological subtypes of primary central nervous system tumors (PCNST). Second, they compared the incidence rates at an international or intercontinental level, resulting in an obvious bias due to differences among continents and European countries in terms of case registrations and access to healthcare. In a study from our team based on data from the French Brain Tumor DataBase [110–112], we analysed the geographical distribution of a homogeneous series of 4790 newly-diagnosed and histologically confirmed diffuse WHO grade II and III gliomas (2099 grade II, 2484 grade III, 38 NOS “not otherwise specified” astrocytomas, 25 NOS oligoastrocytomes and 144 NOS gliomas) in metropolitan France, over a four-year period (2006–2009) [5]. The overall crude rate was 19.4/106. We found that the geographical distribution by region was heterogeneous, with higher incidence rates in Northeast and central parts of France, for all included tumors as well as for grade II and grade III gliomas analysed separately. Since France has a unique healthcare system, access to healthcare is likely to be similar in all regions, especially as the distribution of neurosurgical centers is roughly homogeneous in the metropolitan French territory, with one or more neurosurgical center(s) (depending on the number of inhabitants) in each region (and no region without a neurosurgical center). Therefore, the demonstrated uneven geographical distribution of DLGG raises the question of the role of environmental risk(s) factor(s) (environmental theory).
A possible hypothesis for the uneven DLGG geographical distribution is the impact of environmental factors on DLGG risk. To date, however, there is no demonstrated environmental risk factor of DLGGs. Interestingly, a geographical heterogeneity in the incidence rates was highlighted for other cancers. In France, the incidence rates for all cancers are higher in the North and Northeast regions (data from the National Cancer Institute: http://www.e-cancer.fr/publications/69-epidemiologie/574-la-situation-du-cancer-en-france-en-2011). Especially, the distribution of colorectal cancer (CRC) was comparable to that of WHO grade II and III gliomas in our study, with similar higher incidence rates in the Northeast part of France. We can thus hypothesize that these two diseases may share some risk factors. Some diets were validated as risk factors in CRC with red and processed meat, alcohol, obesity or abdominal fat being strongly associated with a higher risk of CRC, while the “Mediterranean diet” prevents its occurrence [113, 114]. A working hypothesis could thus be that diet factors, and in particular processed meat, may have an impact on the risk of developing WHO grade II and III gliomas. N-nitroso compounds, mostly found in processed meat, are considered as carcinogenic [115]. Regarding gliomas, an experimental model in rat showed an increased occurrence of glioma after intravenous injections of N-nitroso compounds [116]. In France, the consumption of red and processed meat is higher in the Northeast regions (CREDOC, research center for the study and the observation of living conditions, http://www.credoc.fr/pdf/4p/101.pdf). In epidemiological studies, processed meat has been inconsistently associated with glioma risk [117–121]. Two cohort studies and a large international case-control study found no association between processed meat consumption and the risk of glioma [117–119]. In the case-control study, astrocytomas, oligodendrogliomas and glioblastomas were analyzed both together and separately. Two recent meta-analyses found similar results, with a modest increase of the glioma risk associated with consumption of processed meat in case-control studies but not in cohort studies [120, 121]. So far, no epidemiological study has investigated the association between processed meat and WHO grade II and III glioma risk specifically. Other diet factors have been studied, but none has been associated with DLGG or glioma risk yet. Another interesting observation is the relative similarity between the geographical distribution of WHO grade II and III gliomas [5] and that of multiple sclerosis [122, 123]. As WHO grade II and III gliomas have the same pattern of geographical distribution than multiple sclerosis, these two diseases may share some risk factors. Indeed, there is strong evidence that environmental factors influence the distribution of multiple sclerosis [124]. In particular, sunlight exposure (and, consequently, outdoor activities) is significantly associated with multiple sclerosis prevalence (sunny areas being associated with low prevalence). This association probably involves vitamin D, as a low prevalence of multiple sclerosis has been reported in countries with a poor amount of sunlight but a high consumption of vitamin D (oily fish) [124, 125]. We could thus hypothesize that sunlight and vitamin D intake influence the risk of WHO grade II and III gliomas. Based on the map of the sunlight provided by the Joint Research center of the European Commission (available at: http://re.jrc.ec.europa.eu/pvgis/cmaps/eu_cmsaf_opt/G_opt_FR.png), it seems that regions with low sunlight correlate with those with higher incidence rates of WHO grade II and III gliomas in our study, supporting the hypothesis of an association between sunlight and WHO grade II and III gliomas risk. This hypothesis is also supported by a study that found a reverse association between UVB irradiance and brain tumor risk [126].
The uneven geographical distribution of DLGGs in France [5] also raises the question of the role of genetic risks factors that could be involved in some geographical regions (genetic susceptibility, the genetic predisposition theory).
34.4 The Genetic Predisposition Theory
Genetic susceptibility variants could also impact on the risk of DLGG development and have been widely studied in the past years to better understand the origins of this entity. The DLGG molecular epidemiology is extensively reviewed in a previous chapter of the present book (“Molecular Epidemiology of DLGG”); we will only briefly summarize the current knowledge on the subject. Interestingly, about 5% of glioma patients have a familial glioma history [127]. While these aggregations could be in relation with the environment (subjects in a family share environmental exposures), they suggest the existence of a genetic susceptibility. Among susceptibility factors, monogenic genetic syndromes account for 1% of familial cases of glioma [128–130]. Beyond these monogenic syndromes, some non-syndromic genetic factors of susceptibility seem to influence the risk of glioma as well [131].
A familial history of brain tumor is a risk factor of glioma in non-syndromic families [85, 131–133], including for DLGG [131, 132] and oligodendroglial tumors [85]. A pooled analysis of data from two case-control studies found an increased risk of melanomas, sarcomas and PCNST (including DLGG) in first-degree relatives of glioma patients, with no increase in the global cancer risk [131]. In the study published by McCarthy et al., the risk of developing a diffuse WHO grade II and III oligodendroglioma was increased in patients with a familial history of PCNST (odd-ratio = 1.8 CI 95% 1.1–3.1) [85]. In a Swedish study, the standardized incidence was 3.65/105 (CI 95% 2.31–5.47) for first-degree relatives of DLGG patients, and 7.00/105 (CI 95% 3.35–12.87) for siblings, which is significantly higher than the reported incidence in the general population [132]. Various studies have tried to characterize these familial gliomas on a genetic point of view. First, comparative genomic hybridization techniques were used and have suggested that familial gliomas are relatively close to “eneral” gliomas, with only few inconsistently-reported specific alterations [134]. Other studies used linkage analyses to identify candidate genes/genetic variants in familial gliomas. The GLIOGENE project (“Genetic Epidemiology of Glioma International Consortium”) was set up in 2006 and includes glioma families treated in 14 institutions and five countries [127]. It allowed the identification of a region of interest located on chromosome 17q [135, 136]. Further analyses of this specific region (17q sequencing) pointed out several candidate genes (Myo19, KIF18B, SPAG9 in particular) [137]. However, none of these genes was found in common for all the described glioma families. Another region of interest located on chromosome 15q23 was reported in several studies [138, 139]. These linkage studies, however, face major difficulties, in particular the fact that, due to the poor survival of many glioma patients, only few families comprise more than two cases alive simultaneously.
More recently, other genetic risk factors for glioma were studied, in particular genetic polymorphisms [140]. These studies found associations between a number of single nucleotide polymorphisms (SNPs) and the risk of the glioma [141–143]. To date, eight SNPs located in seven genes have been associated with an increased risk of glioma in genome-wide association studies (GWAS) [142]: rare genetic variant in the TP53 gene (glioblastomas and other gliomas) [144–146]; genetic variants in the EGFR gene (gliomas) [147, 148] ; in the RTEL1 gene (“regulator of telomere elongation helicase”) (gliomas) [149–151]; in the TERT gene (“telomerase reverse transcriptase”) (oligodendrogliomas and astrocytomas, whatever the grade or the IDH status) [149, 151] ; in the 8q24 locus/CCD26 gene (gliomas, in particular oligodendrogliomas and IDH-mutated tumors, and including DLGG) [152–155] ; in the PHLDB1 gene (IDH-mutated gliomas, whatever the tumor grade and histological subtype) [151, 155, 156] ; and in the CDKN2B gene [149, 150, 157]. These SNPs identified by GWAS studies have been further confirmed in a large case-control study [157], as well as in a meta-analysis [158]. Genetic variants of TERT, RTEL1, EGFR and TP53 were found associated with an increased risk of glioma, whatever the tumor grade or histological subtype [143]. On the contrary, genetic variants of PHLDB1 and CCDC26 (±CDKN2B): conflicting results [155] were shown to be associated with an increased risk limited to specific grades and/or histological subtypes of gliomas. Candidate-gene studies have also identified a number of candidate genes involved in several biological pathways: folate metabolism [159], DNA-repair pathways (XRCC ou “X-ray repair cross complementing group”, [160] apoptosis signaling pathway (i.e., CASP8 [161]. However, none of these SNPs has been confirmed in the large case-control study published in 2013 [157].
34.5 The Clinical Implications
Such spatiotemporal, functional, and biological considerations may have important implications with regard to the therapeutic strategy and management of DLGG patients. First, the dynamic interactions between DLGG and the brain may vary depending on the eloquence of the areas involved by the tumor. Indeed, it was shown that slow-growing DLGG might induce cerebral plasticity, explaining why most patients showed no or only mild neurological deficit despite voluminous gliomas, even in the so-called “critical” regions [162, 163]. Nonetheless, a recent atlas of DLGG resectability demonstrated that some cerebral areas had low compensatory capabilities [164], constituting a “minimal common brain” among patients [165]. As a consequence, the extent of surgical resection (and thus the median survival [166]) is correlated with the location of the tumor (with regard to the cortex as well as the white matter pathways, therefore the distance from the subventricular zone), that is, with a better tumor removal in “non-eloquent” than in “eloquent” areas and in “compensable” rather “non compensable” structures [9, 167–169]. In addition, a different genetic pattern in temporo-insular DLGG, with a less frequent IDH mutation and 1p19q codeletion compared with frontal DLGG, could also account for the poorer prognosis of these tumors. Indeed, DLGG prognosis was shown to vary according to the tumor location, frontal DLGG being associated with a better intrinsic prognosis compared with temporal or insular tumors whatever the extent of resection [6]. As a consequence, it might be pertinent to modulate the treatment strategies according to these two subgroups of patients, and discuss a more aggressive strategy for temporo-insular DLGG with, for example, earlier chemotherapy regimens after surgical resection.
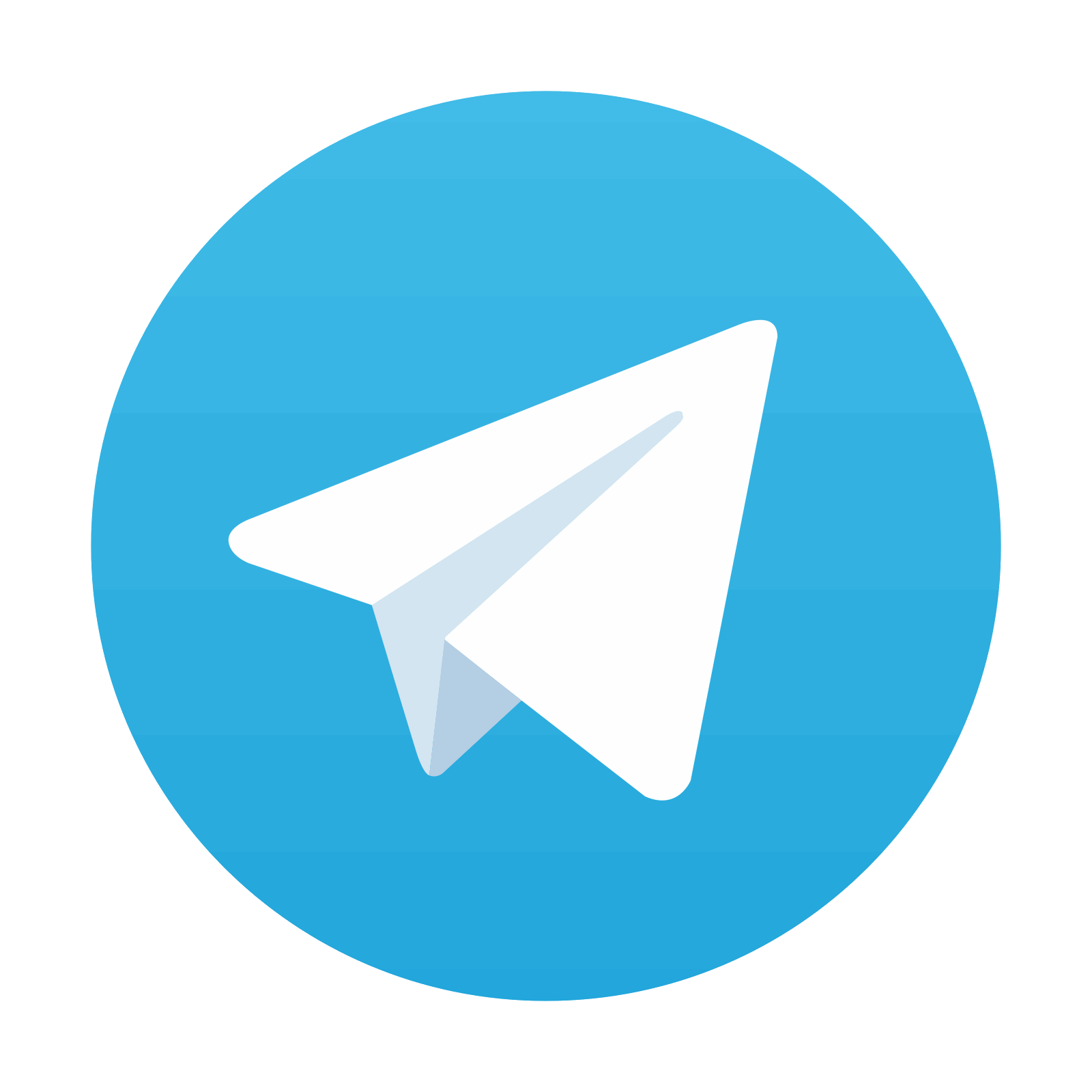
Stay updated, free articles. Join our Telegram channel

Full access? Get Clinical Tree
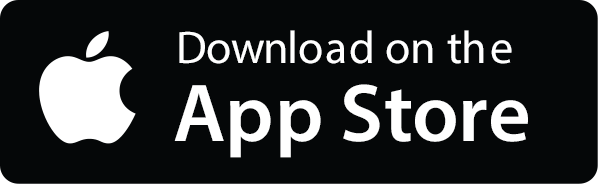
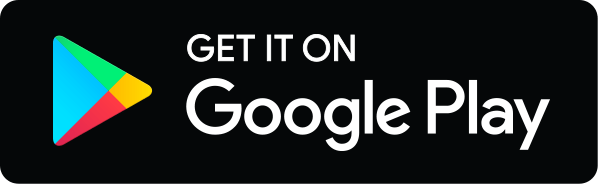