Condition
Fetal
Alloimmune
Congenital infection (e.g. CMV, toxoplasma, rubella, HIV)
Aneuploidy (e.g. trisomies 13, 18, 21)
Autoimmune (e.g. ITP, SLE)
Severe rhesus hemolytic disease
Inherited (e.g. Wiskott-Aldrich syndrome)
Early-onset neonatal (<72 h)
Chronic fetal hypoxia (e.g. pre-eclampsia, IUGR, diabetes)
Perinatal asphyxia
Perinatal infection (e.g. E. Coli, GBS, Hemophilus influenzae)
DIC
Alloimmune
Autoimmune (e.g. ITP, SLE)
Congenital infection (e.g. CMV, toxoplasma, rubella, HIV)
Thrombosis (e.g. aortic, renal vein)
Bone marrow replacement (e.g. congenital leukemia)
Kasabach-Merritt syndrome
Metabolic disease (e.g. propionic and methylmalonic acidemia)
Inherited (e.g. TAR, CAMT)
Late-onset neonatal (>72 h)
Late-onset sepsis
NEC
Congenital infection (e.g. CMV, toxoplasma, rubella, HIV)
Autoimmune
Kasabach-Merritt syndrome
Metabolic disease (e.g. propionic and methylmalonic acidemia)
Inherited (e.g. TAR, CAMT)
22.1.2 Clinical and Laboratory Features of Thrombocytopenia
22.1.2.1 Alloimmune
Neonatal alloimmune thrombocytopenia (NAIT) constitutes less than 5 % of cases of neonatal thrombocytopenia but is important to exclude due to its associated morbidity and mortality [13], and the requirement for specific platelet transfusion components matched for the alloantibodies. The diagnosis and management of this condition is dealt with in Chap. 16.
22.1.2.2 Congenital Infection
The toxoplasma, rubella, cytomegalovirus, herpes (TORCH) viral infections can cause thrombocytopenia although the exact mechanism is unclear. Parvovirus can also cause thrombocytopenia through suppression of bone marrow and megakaryocyte colony formation [14]. Other viruses known to cause thrombocytopenia include enterovirus, Coxsackie virus, adenovirus, Epstein-Barr virus, and dengue virus. HIV is known to infect megakaryocytes and suppress colony growth [15] and is so frequently associated with neonatal thrombocytopenia that it has been suggested as a screening tool [16].
22.1.2.3 Aneuploidies
Thrombocytopenia is common although rarely severe in the trisomies 18 (86 %), 13 (31 %), and 21 (6 %), as well as in Turner syndrome (31 %) [17]. The mechanism of thrombocytopenia in trisomies 13 and 18 is unknown although it may have its etiology in chronic fetal hypoxia. Thrombocytopenia in trisomy 21 is similar to that seen in growth-restricted infants: neutropenia, polycythemia and increased circulating normoblasts. Ten percent of infants with Down syndrome, however, develop a clonal preleukemic disorder showing increased myeloblasts and variable levels of thrombocytopenia, and 20–30 % of these babies will develop acute megakaryoblastic leukemia within the first 5 years of life [18]. It is thought that trisomy of chromosome 21 disturbs fetal hemopoiesis and predisposes to mutations in the GATA1 gene, a key megakaryocyte/erythroid transcription factor [19].
22.1.2.4 Autoimmune
Autoimmune conditions such as maternal idiopathic thrombocytopenic purpura, which may occur in association with systemic lupus erythematosus, can also cause thrombocytopenia in the fetus and neonate. This is dealt with in Chap. 15.
In addition to anemia, neutropenia and reticulocytosis, thrombocytopenia is common in severe rhesus hemolytic disease, especially after intrauterine or exchange transfusions; this is due to platelet-poor blood product and suppression of platelet production in favor of erythropoiesis.
22.1.2.5 Inherited Thrombocytopenias
These are caused by reduced platelet production due to abnormal hemopoietic stem or progenitor cell development. They may be associated with defective platelet function. Many of these disorders will be diagnosed by their associated congenital anomalies.
Bernard-Soulier Syndrome
This syndrome can lead to fetal or early neonatal thrombocytopenia although severe bleeding is not often seen in neonates. It is characterized by mild thrombocytopenia with giant megakaryocytes. It has an autosomal recessive pattern of inheritance and leads to defects in the glycoprotein 1b-IX-V complex [20, 21], which results in defective platelet adhesion (see Chap. 13). Neonates can develop alloantibodies following platelet transfusions, so transfusions should be restricted to cases of severe hemorrhage.
Wiskott-Aldrich Syndrome and X-Linked Thrombocytopenia
These syndromes form part of a spectrum of disorders caused by a mutation in the WAS protein of the X chromosome [22] which leads to disorders of platelet function and survival. X-linked thrombocytopenia can present in the neonatal period with a mild thrombocytopenia. These conditions are characterized by microthrombocytopenia, eczema, immunodeficiency, and recurrent bacterial and viral infections [23].
22.1.3 Timing of Onset of Thrombocytopenia
22.1.3.1 Early-Onset Thrombocytopenia
Chronic Fetal Hypoxia
Placental insufficiency is associated with early-onset thrombocytopenia in preterm neonates. Impaired delivery of oxygen and essential nutrients is implicated in its development. Platelets are initially produced in the fetal liver, with production transferred to the bone marrow in the third trimester [11]. In hypoxia, blood flow to the liver falls. Previous studies have also demonstrated a reduction in circulating megakaryocyte progenitors, suggesting decreased platelet production as a cause [11, 12, 14]. Typically, thrombocytopenia is mild to moderate and self-limiting, resolving in the majority of cases before 10 days of age [12]. Additional hematological abnormalities which can help to confirm growth restriction and hypoxia as causative factors include transient neutropenia, increased circulating nucleated red cells, and evidence of hyposplenism (spherocytes, target cells, and Howell-Jolly bodies) [12, 24].
Perinatal Asphyxia
Thrombocytopenia can occur after perinatal asphyxia as a result of bone marrow suppression and reduced platelet production. Asphyxia-related liver dysfunction also causes decreased production of coagulation factors. Increased consumption of platelets often follows severe acidosis, hypoxia, and endothelial damage and disseminated intravascular coagulation (DIC).
Perinatal Infection
Group B streptococcus (GBS) and gram-negative enteric organisms (predominantly Escherichia coli) account for 70 % of early-onset sepsis. Non-typeable Haemophilus influenzae sepsis has been increasingly identified in neonates, particularly those that are premature. Other gram-negative enteric bacilli such as Klebsiella sp. and gram-positive organisms such as Listeria monocytogenes, enterococci, group D streptococci, α-hemolytic streptococci, and staphylococci have also been implicated. The thrombocytopenia in sepsis is most likely due to increased platelet destruction; platelet levels drop quickly after onset of symptoms of infection [25, 26]. The mean duration of thrombocytopenia is 5–6 days [25, 26]. Platelet transfusions given to septic infants have a shortened life span [26].
Thrombotic Disorders
Bone Marrow Infiltration
Congenital leukemia, osteopetrosis, and histiocytoses can also cause thrombocytopenia secondary to bone marrow infiltration.
Kasabach-Merritt Syndrome
Thrombocytopenia in this condition is associated with sequestration of platelets on the endothelium of hemangiomas, either cutaneous (80 %) or visceral [30]. Thrombocytopenia may be associated with DIC and microangiopathic anemia. Treatment options include corticosteroids, antifibrinolytic agents (e-aminocaproic acid and tranexamic acid), interferon alpha-2a, cytotoxic chemotherapy, intralesional steroids, embolization, and laser therapy.
Metabolic Disease
The organic acidemias, propionic, methylmalonic, and isovaleryl academia, can also present with neonatal thrombocytopenia and neutropenia, mimicking neonatal sepsis [31]. The clinical finding is of a healthy newborn at birth that rapidly becomes ill after the first day of life. Urine organic acid analysis is diagnostic.
Lysosomal storage diseases such as Gaucher’s disease can also present with thrombocytopenia [32]. These disorders are characterized by mutations in one of a series of enzymes involved in the degradation of glycosphingolipids. Patients can present with hepatosplenomegaly, cardiomyopathy, renal dysfunction, anemia, thrombocytopenia, and neurological degeneration. Thrombocytopenia associated with splenomegaly may also be secondary to infiltrative metabolic disorders such as the mucolipidoses and mucopolysaccharidoses.
Inherited Disorders
Inherited disorders typically presenting in the early to late neonatal period with variable severity of onset include Bernard-Soulier syndrome, X-linked thrombocytopenia, thrombocytopenia-absent radius syndrome, amegakaryocytic thrombocytopenia with radioulnar synostosis, and congenital amegakaryocytic thrombocytopenia.
Fanconi anemia is characterized by short stature, skeletal anomalies, increased incidence of solid tumors and leukemias, and aplastic anemia. It is rare to present with thrombocytopenia in the neonatal period although it should be considered in the presence of dysmorphic features and unexplained thrombocytopenia. The diepoxybutane test is almost always diagnostic of this disorder [33].
Thrombocytopenia-Absent Radius (TAR) Syndrome
Thrombocytopenia-absent radius (TAR) syndrome is a rare autosomal recessive condition in which thrombocytopenia is associated with bilateral radial aplasia, although thumbs may be present and normal. It can also be associated with lower limb abnormalities (47 %), renal anomalies (27 %) and cardiac defects (15 %) [34]. Episodes of thrombocytopenia begin in the neonatal period, and around 50 % of affected infants are symptomatic (showing evidence of bleeding) in the first week of life, with a further 40 % becoming symptomatic by the age of 4 months [35]. The platelet count improves spontaneously after the first year of life. The therapy of TAR syndrome is supportive with platelet transfusions for hemorrhage, red cell transfusions for anemia, and avoidance of stressors, such as cow’s milk, which may induce thrombocytopenia.
Amegakaryocytic thrombocytopenia with radioulnar synostosis (ATRUS) is caused by mutations in the HOXA11 gene and is characterized by severe thrombocytopenia from birth, radioulnar synostosis, clinodactyly, and shallow acetabula [36].
Congenital amegakaryocytic thrombocytopenia (CAMT) is an autosomal recessive condition that almost always presents early in the neonatal period with severe thrombocytopenia; 50 % of affected infants will have evidence of bleeding [37]. Although there is isolated thrombocytopenia in the newborn period, 50 % will go on to develop aplastic anemia in childhood. Stem cell transplantation is curative in cases of severe disease [38].
22.1.3.2 Late-Onset Thrombocytopenia
Thrombocytopenia occurring after 72 h of age is due to sepsis or NEC in more than 80 % of cases [14].
Late-Onset Sepsis
The vast majority of late-onset infections are caused by gram-positive organisms, with coagulase-negative staphylococci accounting for almost half of infections [39]. The rate of infection is inversely related to birthweight and gestational age. Candida species are increasingly important causes of late-onset sepsis, occurring in 12 % of very low birthweight infants [40]. Thrombocytopenia occurs in 79–100 % of these infants [41, 42]. In late-onset sepsis, thrombocytopenia is often more severe (<30 × 109/L) and of rapid onset, and can take several weeks to resolve [8].
Necrotizing Enterocolitis
This is the most common neonatal gastrointestinal illness, affecting predominantly preterm infants (90 % of cases occur in premature infants). Surgery is required in 20–60 %, and there is a mortality rate of 20–28 % [42]. In one study, 90 % of infants with NEC had thrombocytopenia (<150 × 109/L), with 55 % of these infants having platelet counts below 50 × 109/L [43]. Within the group with severe thrombocytopenia, 55 % had severe bleeding complications. Severe thrombocytopenia had an 89 % positive predictive value for intestinal gangrene in one study [44]. The most likely reason for low platelets is increased platelet destruction although there may be suppression of platelet production by pro-inflammatory cytokines.
22.1.4 Treatment of Neonatal Thrombocytopenia
Neonatal thrombocytopenia and bleeding are common and important clinical problems for preterm neonates. The main specific treatment for thrombocytopenia in the neonate is platelet transfusion, but intravenous immunoglobulin (IVIg) may be used for maternal ITP-associated thrombocytopenia. Current national guidance recommends platelet transfusion thresholds of 20–30 × 109/L and 50 × 109/L for neonates depending on the clinical situation [45], but policies and protocols for neonatal platelet transfusion therapy vary widely among clinicians and institutions [46, 47]. This variation reflects the generally broad nature of recommendations in national guidelines, which themselves are based largely on consensus rather than evidence, and often extrapolated from data on adults [48, 49].
Platelet transfusions are frequently used in modern neonatal clinical practice as prophylaxis in thrombocytopenic neonates [49]. Previous studies estimate that 25 % of neonates and 50 % of extremely low birthweight neonates whose platelet counts fall below 150 × 109/L receive one or more transfusions [50, 51]. In the only randomized controlled trial to assess a threshold level for the effectiveness of neonatal prophylactic platelet transfusions, moderate thrombocytopenia (50–150 × 109/L) was not detrimental to short-term neonatal outcome. However, neonates with severe thrombocytopenia (platelet count less than 50 × 109/L) were excluded from the study because of their perceived high risk of hemorrhage [52]. Benefits of transfusion need to be weighed against possible risks.
22.1.5 Conclusions
The most common cause of early thrombocytopenia in neonates is chronic hypoxia. NAIT accounts for less than 5 % of all cases of neonatal thrombocytopenia. Sepsis and NEC account for more than 80 % of the late-onset thrombocytopenia. Inherited causes of thrombocytopenia are rare. Infants with severe thrombocytopenia and evidence of bleeding should receive platelets, but the role of prophylactic platelets in the neonate with asymptomatic thrombocytopenia is unclear.
Key Learning Points
The underlying cause of thrombocytopenia is predicted by the timing of onset.
Chronic fetal hypoxia is the most common cause of early-onset thrombocytopenia and is mild and self-limiting.
Sepsis and/or NEC is the most common cause of late-onset thrombocytopenia.
Bleeding risk is greater in NAIT than in sepsis/NEC and greater in sepsis/NEC than in chronic fetal hypoxia.
The role of prophylactic platelet transfusions and the optimal platelet count thresholds are not known.
22.2 Neonatal Thromboembolism
22.2.1 Introduction
Neonatal thromboembolism is a rare but increasingly reported condition in the neonatal unit that can cause significant neonatal mortality and morbidity. Ninety percent of thromboembolism is associated with thrombosis of arterial or venous catheters and central venous lines, but stroke is also prevalent, and the incidence of perinatal stroke ranks second only to the strokes of the elderly population (1 in 2,300 to 1 in 5,000 births) [53].
22.2.2 Etiology of Neonatal Thromboembolism
Idiopathic thromboembolism accounts for less than 1 % of cases of newborn thromboembolism as compared to 40 % in the adult population [54]. Usually at least one risk factor can be identified, either maternal or fetal, including maternal diabetes or SLE with antiphospholipid syndrome, pre-eclampsia, IUGR, maternal autoimmune conditions, maternal thrombophilia, placental vasculopathy, infection, hypovolemia, dehydration, hypoxia, acidosis, and neonatal prothrombotic disorders [55–62].
The prothrombotic disorders that have been linked with neonatal thromboembolism include deficiencies in protein C, protein S, antithrombin, and plasminogen [63–66]. Homozygous deficiencies of these factors usually present with severe clinical symptoms and require urgent management. However, diagnosing heterozygous states can be difficult in the neonate because physiological values are lower than in older children and adults [67]. Diagnosis can also be complicated by the coexistence of acquired disorders – present in over 80 % of newborns with systemic thromboembolism [68]. Of note, homozygous plasminogen deficiency is associated with ligneous conjunctivitis, and in some cases with occlusive hydrocephalus [66], the pathogenesis of which is unclear. Both homozygous and heterozygous plasminogen deficiency do not appear to be associated with spontaneous thrombotic events, although repeated thrombotic occlusion of implanted catheters has been described [66, 69, 70]. It has been suggested that patients with heterozygous type I plasminogen deficiency who develop thrombosis may have additional risk factors such as deficiency or protein C or S or antithrombin deficiencies [70].
The clinical presentation of homozygous protein C deficiency in the neonatal period is purpura fulminans, cerebral stroke, and/or large-vessel thrombosis. The diagnosis is confirmed in the presence of these clinical features with an undetectable protein C level and both parents testing as heterozygotes for protein C deficiency. Heterozygous protein C deficiency does not normally present with thrombosis in early infancy [71], but cohort studies have indicated an increased prevalence of protein C heterozygosity in neonates with thromboembolism and additional risk factors [72]. Babies with homozygous protein S deficiency also present with purpura fulminans, but like protein C deficiency, the heterozygous state is only uncommonly associated with thromboembolic phenomena in infancy or childhood [73]. Homozygous antithrombin (AT) deficiency, where levels are less than 10 % of normal, is rare but can be associated with severe thromboembolic events, venous or arterial [74]. Heterozygous AT deficiency is associated with diverse but less severe thrombotic events.
The presence of Factor V Leiden or the prothrombin G20210A mutation have also been linked to neonatal thromboembolism [61, 62, 75, 76]. Approximately 5 % of Caucasians are heterozygous for the factor V Leiden mutation (R506Q) at the cleavage site for activated protein C (APC), which decreases the rate of inactivation of factor V, leading to a reduced anticoagulant effect of APC (APC resistance [77]); and 2 % for the prothrombin G20210A mutation, that is associated with increased prothrombin levels possibly due to increased mRNA stability [78]. A homozygous polymorphism in the C677T methylene tetrahydrofolate reductase (MTHFR) gene has been linked to an increased thrombotic risk in infants with perinatal arterial stroke [79]. Homozygosity for this polymorphism, in the presence of folate deficiency, may predispose to hyperhomocystinaemia, with the phenotypic expression silenced by folic acid supplementation. An elevated homocysteine level has also been associated with an increased thrombotic risk [80], but the contribution of these risk factors to neonatal thrombosis is unclear.
22.2.3 Clinical Presentation
22.2.3.1 Thrombosis Following Umbilical Catheterization
Studies have identified central venous lines as an important risk factor for neonatal thrombosis [81]. Clinical studies have shown an incidence of 13 % [82] and autopsy studies a 20–65 % incidence of venous thromboembolism in infants with an umbilical venous catheter (UVC) in situ [83, 84]. UVCs have been associated particularly with portal venous thrombosis. Major venous thrombosis due to umbilical artery catheters (UAC) occurs in 1–3 % of all infants [85]. High position of the UAC (UAC tip between T6–T10) may be associated with fewer complications compared to low position (UAC tip L3–L5) [86].
Many factors contribute to catheter-related thrombosis, including vessel caliber, blood flow, and catheter type. Use of a heparin infusion [87] or heparin-bonded lines [88] prolongs the duration of catheter patency but has not been shown to alter the rate of catheter-related thrombus. In order to reduce the incidence of catheter-related thrombosis, catheter use should be restricted to those situations in which it is truly necessary.
The majority of babies with UAC-related thrombosis remain asymptomatic, but some babies can show evidence of loss of patency of the catheter, limb ischemia, and NEC secondary to mesenteric artery occlusion, or hypertension secondary to renal artery occlusion. Contrast angiography is the gold standard diagnostic method but rarely available in neonates. Ultrasound may not pick up all cases of arterial obstruction [89]. Long-term morbidity can include hypertension, impaired renal function and paraplegia.
Clinical features of venous catheter-related thromboembolism include loss of central venous line patency, venous congestion, and/or discoloration of the limbs. Superior vena caval obstruction leads to facial and venous congestion of the upper chest. Pulmonary thromboembolism presents with respiratory compromise. Long-term complications of thromboembolism include prominent collateral circulation, catheter-related sepsis, chylothorax, portal hypertension, and post-thrombotic syndrome.
22.2.3.2 Renal Vein Thrombosis
Renal vein thrombosis is the most common non-catheter-related thromboembolism in neonates and is responsible for around 10 % of all thromboembolism. It is commonest in the first week of life, and almost 80 % of all renal vein thromboses occur in the first month of life [90, 91]. Reduced renal blood flow, increased blood viscosity, hyperosmolarity, hypercoagulability due to perinatal asphyxia, polycythemia, dehydration, septicemia, and maternal diabetes have been suggested as possible pathophysiological mechanisms. Newborns usually present with hematuria, proteinuria and a flank mass. Bilateral renal vein thrombosis is present in 24 % of newborns [92]. Ultrasound is the diagnostic method of choice. There are no recent studies on long-term morbidity of this condition.
22.2.3.3 Perinatal Stroke
Perinatal ischemic stroke is defined as “a group of heterogeneous conditions in which there is a focal disruption of cerebral blood flow secondary to arterial or venous embolization between 20 weeks of fetal life and the 28th day of postnatal life, confirmed by neuroimaging or neuropathologic studies” [93]. The incidence of ischemic perinatal stroke is 0.25–1 infants per 1,000 live births and accounts for 30 % of all children with hemiplegic cerebral palsy born at term or late preterm [53, 55, 94].
Early neonatal stroke typically presents in the first 3 days and can occur as a result of placental embolism, infection, birth trauma, or hypoxic ischemic encephalopathy (HIE). Late neonatal stroke occurs between 4 and 28 days of age and can be secondary to infection, cardiac disease, or venous thrombosis with paradoxical embolism. Neonates with stroke may present with focal or generalized neonatal seizures, or with non-specific features such as apnea, poor feeding, irritability, abnormalities in tone, or cyanosis. The differential diagnosis includes kernicterus, bacterial and viral encephalitis, mitochondrial disorders, tumors, or hypoglycemia.
Several classifications of neonatal stroke have been suggested, based either on distribution of these events [95] or on the proposed underlying etiological mechanism [96]. In the neonatal period, two common subtypes of stroke are arterial ischemic stroke (AIS) and cerebral sinovenous thrombosis. In AIS it is thought that emboli originating in the placenta pass through the foramen ovale and occlude the intracerebral vessel. Neonatal AIS is characterized by documented complete or incomplete occlusion of an intracerebral vessel causing a focal lesion in the brain, or a documented lesion that can be explained only by occlusion of a particular vessel [97]. Studies have shown that middle cerebral artery infarction accounted for between 50 and 83 % of focal events [95].
Sinovenous thrombosis accounted for 45 % of all neonatal stroke cases registered in the Canadian registry, with an incidence of 41 per 100,000 births [53]. Risk factors such as maternal diabetes, hypertension, infection, asphyxia, complicated delivery, and congenital cardiac disorders have been commonly identified [98].
Additional forms of vaso-occlusive ischemic events unique to the perinatal period are periventricular venous infarction and presumed fetal stroke detected in later infancy. Less common types of neonatal stroke are multifocal infarctions, subcortical infarctions, and infarctions caused by meningitis, thrombophilia, and arteriopathy. Maternal cocaine abuse has also been associated with cortical infarction in up to 17 % of exposed infants [99].
Neuroimaging remains the mainstay in the diagnosis of stroke. Cranial ultrasound may miss the cerebral ischemic lesions, in particular more anterior or posterior lesions [100, 101]. Appropriately timed conventional T2-weighted magnetic resonance imaging (MRI), diffusion-weighted imaging, and magnetic resonance angiography remain the principal methods of diagnosing neonatal stroke [102, 103]. Infants diagnosed with stroke should be investigated for thrombophilia.
Outcome data vary but, overall, neurological deficits or epilepsy occur in two thirds of survivors of acute ischemic stroke, with sensori-motor deficit being the most common. Lesions involving the motor cortex, basal ganglia, and internal capsule together are more likely to be associated with motor disability than lesions limited to the cortex or basal ganglia alone [104]. Seizure disorders have been shown to occur in 15 % of long-term survivors of AIS [82]. However, fewer than 5 % of neonates with AIS have recurrent systemic or cerebral thrombosis [53]. A follow-up study of survivors of cerebral sino-venous thrombosis showed that, in contrast to AIS, 77 % of survivors had no neurological sequelae [105].
22.2.4 Treatment of Neonatal Thrombosis
Treatment options for neonatal thrombosis include supportive care, anticoagulation and thrombolysis. In the past, clinicians have been reluctant to use anticoagulant therapy in neonates due to the possible risks of bleeding. The German registry of neonatal thrombosis reported rates of major hemorrhage of 2 % for heparin anticoagulation and 15 % for thrombolysis [90]. The American College of Chest Physicians (ACCP) guidelines suggest that where possible, pediatric hematologists with experience in thromboembolism manage pediatric patients with thromboembolism; and when this is not possible, a combination of a neonatologist/pediatrician and adult hematologist supported by consultation with an experienced pediatric haematologist [106].
Possible anticoagulants for use in neonates include unfractionated heparin (UFH), low molecular weight heparin (LMWH). However, target therapeutic ranges are based on adult rather than neonatal ranges. Neonates often require high doses of UFH to maintain the target therapeutic range; this is because of physiologically decreased levels of antithrombin, increased volume of distribution, rapid heparin clearance, and non-specific protein binding in plasma [107, 108]. Advantages of LMWH in this population relates mostly to its subcutaneous administration and reduced requirement for monitoring compared with UFH, particularly given that venous access is often limited in infants. There is limited dosing data regarding LMWH use in neonates. Small pharmacokinetic studies of enoxaparin and dalteparin in pediatric patients demonstrate wide ranges of dose requirements, with neonates requiring the highest doses [109–111]. The majority of neonates reach a therapeutic anti-Xa activity level using 1.625 mg/kg/dose. Anti-Xa activity levels effectively monitor LMWH therapy in neonates [112].
Thrombolytic therapy should be considered for neonates with acute thrombosis, such as aortic, atrial or peripheral arterial thrombosis, that is life-threatening or that could potentially lead to loss of a limb, that presents within 2 weeks of symptomatic onset. Thrombolytic agents can be administered systemically or locally. Systemic thrombolysis avoids the requirement for interventional radiologic procedures (often challenging in small children), a requirement for anesthesia, and the delay to therapy potentially encumbered during the organization of local invasive thrombolysis. Higher-dose recombinant tissue plasminogen activator (rt-PA, alteplase) (0.1–0.5 mg/kg/h) in short courses of 6–48 h are generally chosen for arterial clots and can also be used for venous thrombi. Low-dose (0.03–0.06 mg/kg/h) longer duration systemic infusions of t-PA for 12–96 h have been shown to be effective for lysis of venous thrombi [112–114]. Coagulation screening tests including PT, APTT, fibrinogen, plasminogen and D-dimer or FDP levels, should be monitored during therapy to ensure hemostatic levels of platelets and fibrinogen and to determine baseline fibrinolytic potential (plasminogen concentration) and activation (D-dimer levels). The therapeutic action of t-PA requires plasminogen and therefore its effect may be improved by the administration of fresh frozen plasma (FFP) 10 mL/kg daily for plasminogen concentrations less than 50 %. Infusions of thrombolytic agents should be discontinued as soon as clot lysis has been achieved, as there is no potential for further improvement and bleeding complications increase with increasing dose and duration of thrombolytic therapy. Therefore repeated imaging should be undertaken. In addition, cranial ultrasound imaging is recommended prior to initiation of therapy and daily thereafter [114].
Supportive care is usually provided to infants with renal vein thrombosis, and anticoagulant and thrombolytic therapy are controversial in the treatment of this condition. Unilateral renal vein thrombosis with thrombus extending into the inferior vena cava may warrant treatment with LMWH. In bilateral renal vein thrombosis, thrombolysis should be considered [115].
In neonates with clinical presentations of homozygous protein C deficiency, the ACCP recommends administration of either 10–20 mL/kg of FFP every 12 h or protein C concentrate, when available, at 20–60 units/kg until the clinical lesions resolve. For neonates with homozygous protein C deficiency, after initial stabilization, the ACCP recommends long-term treatment with a vitamin K antagonist (VKA) such as warfarin, LMWH, protein C replacement, or liver transplantation [106].
There are no trials supporting the efficacy or safety of anticoagulant therapy for treatment of stroke in the neonate, and currently there are no recommendations for anticoagulation or aspirin for this condition. For neonates with a first arterial ischemic stroke (AIS), in the absence of a documented, ongoing cardioembolic source, the ACCP suggests supportive care over anticoagulation or aspirin therapy. For AIS secondary to cardioembolic causes, the ACCP suggests anticoagulant therapy with LMWH or VKA for at least 3 months. For AIS secondary to cardioembolic causes in children with demonstrated right-to-left shunts (e.g. patent foramen ovale, PFO), the ACCP suggests surgical closure of the shunt [106].
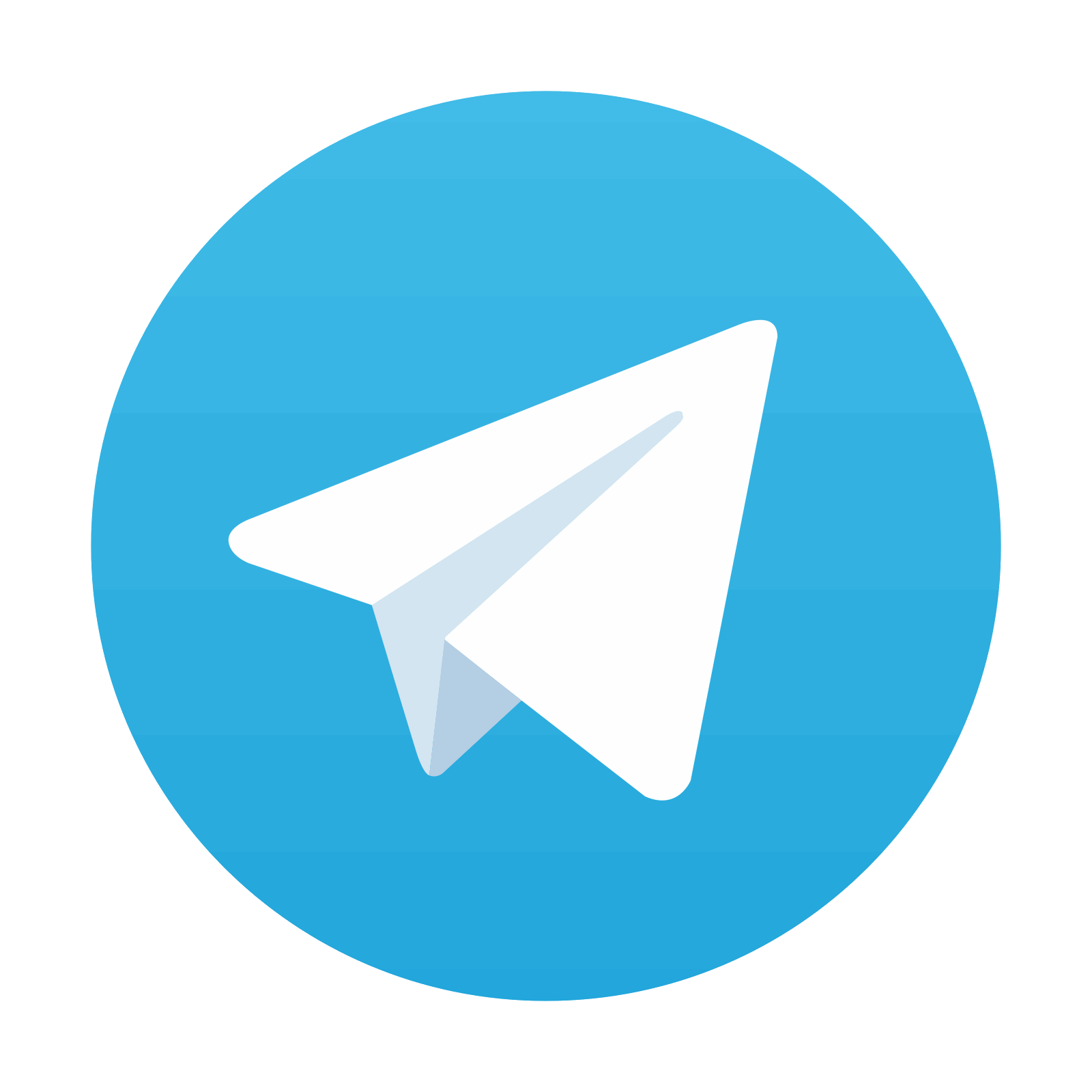
Stay updated, free articles. Join our Telegram channel

Full access? Get Clinical Tree
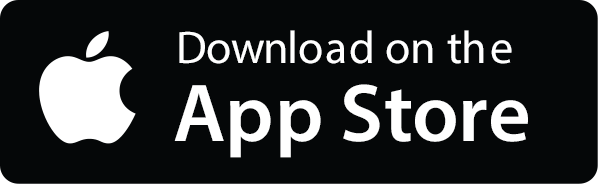
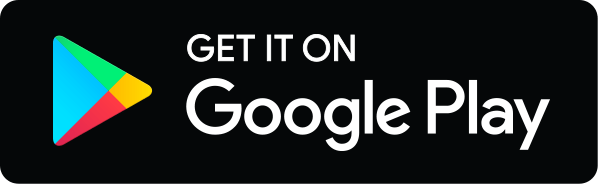