Introduction
At no other time in the life of a patient is the physician confronted with as many diagnostic considerations in the interpretation of apparent disturbances of the erythrocyte as during the neonatal period. Neonatal erythrocytes are fundamentally different from those of older children and adults, varying in size, shape, globin composition, oxygen transport, membrane characteristics, cellular metabolism, and life span. Because of these differences, evaluation of a neonate with a suspected erythrocyte disorder mandates a thorough understanding of developmental erythropoiesis (reviewed in Chapter 1 ), appreciation of the differences between neonatal and adult red blood cells (RBCs), understanding of perinatal conditions influencing the fetus and newborn, and knowledge of inherited and acquired disorders of the erythrocyte that manifest in the neonate.
The Neonatal Erythrocyte
Erythrocyte Size
Early in embryogenesis, the size and volume of erythrocytes are significantly greater than that of neonatal and adult erythrocytes, with the mean corpuscular volume (MCV) ranging from 150 to 180 fl and cellular diameter ranging from 20 to 25 µ. Erythrocyte size and volume decrease throughout gestation to values of MCV between 108 to 118 fl and diameter between 8 to 10 µ at term ( Table 2-1 ). After birth, erythrocytes continue to decrease in size and volume, with values similar to those of adults by 1 year of age.
Gestational Age (wk) | Hematocrit (%) * | Hemoglobin (g/dL) | MCV (fl) | Reticulocytes (%) |
---|---|---|---|---|
18-20 † | 36 ± 3 | 11.5 ± 0.8 | 134 ± 9 | NR |
21-22 † | 38 ± 3 | 12.3 ± 0.9 | 130 ± 6 | NR |
22-23 † | 38 ± 1 | 12.4 ± 0.9 | 125 ± 1 | NR |
24-25 | 63 ± 4 | 19.4 ± 1.5 | 135 ± 0 | 6.0 ± 0.5 |
26-27 | 62 ± 8 | 19.0 ± 2.5 | 132 ± 14 | 9.6 ± 3.2 |
28-29 | 60 ± 7 | 19.3 ± 1.8 | 131 ± 14 | 7.5 ± 2.5 |
30-31 | 60 ± 8 | 19.1 ± 2.2 | 127 ± 13 | 5.8 ± 2.0 |
32-33 | 60 ± 8 | 18.5 ± 2.0 | 123 ± 16 | 5.0 ± 1.9 |
34-35 | 61 ± 7 | 19.6 ± 2.1 | 122 ± 10 | 3.9 ± 1.6 |
36-37 | 64 ± 7 | 19.2 ± 1.7 | 121 ± 12 | 4.2 ± 1.8 |
Term | 61 ± 7 | 19.3 ± 2.2 | 119 ± 9 | 3.2 ± 1.4 |
* Values reported as the mean ± standard deviation.
Erythrocyte Shape and Deformability
Marked variability exists in the shape of fetal and neonatal erythrocytes. Irregularly shaped erythrocytes are found in larger numbers on the peripheral blood smears of hematologically normal term neonates than on those of adults ( Fig. 2-1 ). Acanthocytes, target cells, and immature erythroid cells with various membrane projections are frequently seen. These variations have been attributed to poor or absent splenic function in neonates. Decreased splenic function is also associated with membrane surface “pits,” the site of formation of endocytic vacuoles, attributed to immaturity of the neonatal macrophage pitting process. Nearly half of erythrocytes from preterm infants and a quarter of erythrocytes from term infants have surface pits compared with approximately 2% of normal adult erythrocytes. Erythrocyte deformability is determined primarily by three factors: the surface/volume relationship of the cell, cytoplasmic viscosity, and intrinsic membrane rigidity. Cellular deformability influences several processes, including whole blood viscosity, which in turn affects peripheral vascular resistance and cardiac workload, flow in the peripheral circulation, and the life span of the erythrocyte. As erythrocytes age, neonatal cells lose more volume, have a higher mean corpuscular hemoglobin concentration, and become less deformable than adult RBCs, with decreased deformability leading to increased splenic sequestration. Together these properties lead to accelerated membrane loss and decreased erythrocyte life span.
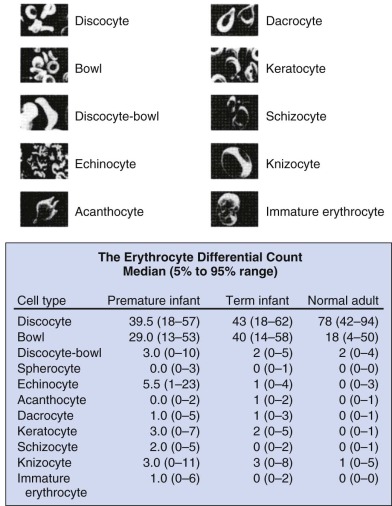
Membrane Differences
Fetal and neonatal erythrocyte membranes demonstrate additional characteristics that differ from those of adult cells ( Box 2-1 ). Fetal and neonatal erythrocytes contain more phospholipid and cholesterol per cell, resulting in a larger surface to volume ratio and rendering them slightly more osmotically resistant. This observation has practical clinical importance, because when osmotic fragility testing is used in a neonate to diagnose hereditary spherocytosis (HS), a normative neonatal osmotic fragility curve should be used rather than the standard adult curve. The surface charge of neonatal erythrocytes is more negative than that of adult cells because of a higher sialic acid content. This increased negative charge contributes to the decreased erythrocyte sedimentation rate observed in newborns. Neonatal erythrocytes contain increased amounts of total and membrane-associated myosin compared with adult cells. Membrane acetylcholinesterase is decreased. Permeability to monovalent cations is increased, and less sodium-potassium adenosine triphosphatase activity is required for monovalent cation removal.
Less osmotically fragile
Differences in surface antigen expression
A and B antigens diminished
Lewis system diminished
I antigen very diminished (or absent): i antigen present
Possible decreased structural stability as a result of increased levels of unbound 2,3-diphosphoglycerate
Membranes less deformable
Red cells of different shapes with a greater predominance of stomatocytes than adult cells
Increased variability of the smear with multiple morphologic changes
Red cell membrane pits (as a result of decreased splenic function)
The expression of various antigens and surface receptors vary between neonatal and adult erythrocytes. The major RBC antigens—Rh, MN, Kell, and Duffy—are well developed in early intrauterine life. Other membrane-associated antigens—Lutheran, ABO, I, and XgA—are incompletely developed at birth. Other antigens, such as the Lewis antigen, are absent at birth. Neonatal erythrocytes express higher levels of several membrane-associated proteins, such as the insulin and insulinlike growth factor receptors, while expressing fewer digoxin receptors than adult erythrocytes.
The i antigen is a commonly used marker of fetal erythropoiesis. Differences in the glycans attached to the external surface of band 3, the anion exchanger, account for this developmental marker. In fetal cells the chain is arranged in an unbranched, linear fashion (i antigen with i reactivity), whereas the chain is arranged in a branched manner in adult cells (I antigen with I reactivity). The i antigen predominates in fetal and neonatal cells, gradually decreasing in a reciprocal relationship with the I antigen, which gradually increases, until approximately 18 months of age. Adult erythrocytes express minimal to no i antigen.
Globin Composition and Oxygen Transport
The synthesis and composition of embryonic, fetal, and neonatal globins are described in Chapter 19 . Depending on gestational age, approximately 70% to 90% of the hemoglobin in the erythrocyte of the fetus and neonate is fetal hemoglobin (HbF). HbF is more soluble in strong phosphate buffers and is resistant to acid denaturation. This latter characteristic is the biochemical basis of the Kleihauer-Betke test (discussed later).
An important functional difference between HbF and adult hemoglobin (HbA) results from the ability of HbA, but not HbF, to interact with phosphorylated compounds, particularly adenosine triphosphate (ATP) and 2,3-diphosphoglycerate (2,3-DPG). The interaction of HbA with 2,3-DPG leads to a marked decrease in oxygen affinity. In contrast, HbF has no or minimal interaction with these organic phosphates, leading to a relatively higher oxygen affinity of fetal blood, with a leftward shift of the oxygen dissociation curve ( Table 2-2 ; Fig. 2-2 ) compared with adult blood. In utero, this characteristic is of significant benefit to the fetus because it facilitates placental oxygen exchange from maternal blood to fetal erythrocytes.
ARTERIAL OXYGEN SATURATION (%) † | ||||
---|---|---|---|---|
P 50 (mm Hg) | 95 | 90 | 85 | 80 |
20 ‡ | 10.0 | 13.0 | 20.0 | >25.0 |
23 | 7.3 | 9.0 | 11.0 | 15.5 |
25 | 6.2 | 7.3 | 8.8 | 11.0 |
27 § | 5.3 | 6.3 | 7.3 | 8.9 |
* Assumes a cardiac output of 250 mL/kg/min and an oxygen consumption of 6.5 mL/kg/min.
† The lower the arterial oxygen saturation, the less resting pulmonary function (e.g., bronchopulmonary dysplasia).
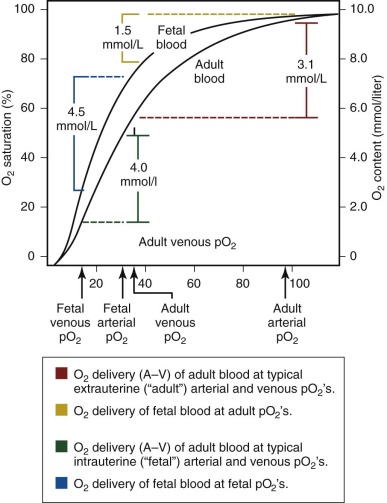
Cellular Metabolism
Fetal and neonatal erythrocytes have a number of metabolic differences compared with adult cells ( Box 2-2 ). Glucose and ATP production are increased, probably representing compensatory activity to maintain normal intracellular homeostasis by energy-dependent ion transport. Several glycolytic enzymes demonstrate increased activity, but this increase may reflect a larger percentage of younger RBCs in the neonatal circulation compared with the adult circulation rather than a characteristic specific to neonatal erythrocytes. Activities of other enzymes, including carbonic anhydrase and methemoglobin reductase, are reduced.
Carbohydrate Metabolism
Glucose consumption increased
Galactose more completely used as substrate under normal circumstances and for methemoglobin reduction *
* Appears to be a unique characteristic of the newborn’s erythrocytes and not merely a function of the presence of young red blood cells.
Decreased activity of sorbitol pathway *
Decreased triokinase activity *
Glycolytic Enzymes
Increased activity of hexokinase, phosphoglucose isomerase, * aldolase, glyceraldehyde-3-phosphate dehydrogenase, * phosphoglycerate kinase, * phosphoglycerate mutase, enolase, pyruvate kinase, lactate dehydrogenase, glucose-6-phosphate dehydrogenase, 6-phosphogluconic dehydrogenase, galactokinase, and galactose-1-phosphate uridyl-transferase
Decreased activity of phosphofructokinase *
Distribution of hexokinase isoenzymes differs from that of adults *
Nonglycolytic Enzymes
Increased activity of glutamic oxaloacetic transaminase and glutathione reductase
Decreased activity of NADP-dependent methemoglobin reductase catalase, * glutathione peroxidase, carbonic anhydrase, * adenylate kinase, * and glutathione synthetase *
Presence of alpha-glycerol-3-phosphate dehydrogenase
ATP and Phosphate Metabolism
Decreased phosphate uptake, * slower incorporation into ATP and 2,3-diphosphoglycerate *
Accelerated decline of 2,3-diphosphoglycerate during red blood cell incubation *
Increased ATP levels
Accelerated decline of ATP during storage or incubation
Storage Characteristics
Increased potassium efflux and greater degrees of hemolysis during short periods of storage
More rapid assumption of altered morphologic forms during brief incubation *
Membrane Characteristics
Decreased ouabain-sensitive ATPase *
Decreased potassium influx *
Decreased permeability to glycerol and thiourea *
Decreased membrane filterability *
Increased sphingomyelin, decreased lecithin content of stromal phospholipids
Decreased content of linoleic acid *
Increase in lipid phosphorus and cholesterol per cell
Greater affinity for glucose *
Other Characteristics
Increased methemoglobin content *
Increased affinity of hemoglobin for oxygen *
Glutathione instability *
Increased tendency for Heinz body formation in the presence of oxidant compounds *
ATP, Adenosine triphosphate; ATPase, adenosine triphosphatase; NADP, nicotinamide adenine dinucleotide phosphate.
One important characteristic of the neonatal erythrocyte is increased susceptibility to oxidant-induced damage, leading to glutathione instability, Heinz body formation, and methemoglobinemia. Alterations in the pentose phosphate pathway, decreased glutathione peroxidase, diminished oxidant capacity of neonatal plasma, variations in superoxide dismutase levels, and decreased membrane sulfhydryl groups have all been implicated in the susceptibility of the neonatal erythrocyte to oxidative stress. This increased susceptibility to oxidative injury may lead to hemolysis when severe hypoxia and acidosis is present in the fetus and newborn.
Life Span
The life span of the erythrocyte in a term neonate is between 60 and 90 days, with preterm infants demonstrating even shorter life spans, from 35 to 50 days. This shortened life span has been attributed to several properties specific to neonatal erythrocytes, including increased membrane deformability, more rapid loss of membrane surface area, increased susceptibility of membrane proteins and lipids to peroxidation, and a rapid decrease in intracellular enzyme activity and ATP content. When neonatal erythrocytes are transfused into adults, they exhibit a shortened life span that is attributed to the intrinsic differences between neonatal and adult erythrocytes. When adult erythrocytes are transfused into a neonate, they exhibit a normal life span.
Normal Hematologic Values
A number of factors influence hematologic values in the neonate, including many of the developmental changes previously listed. Interpretation of laboratory data in the neonate requires consideration of a number of factors, because a value that is normal for one infant may not be normal for another infant. The gestational and chronologic age of the infant, the infant’s sex, timing of cord clamping, and the site of blood sampling are important factors to be considered.
Hemoglobin and Hematocrit
Hemoglobin concentration rises gradually throughout gestation and peaks shortly after birth. The mean hemoglobin at 10 weeks’ gestation is 9 g/dL, increasing to 11 to 12 g/dL by approximately 23 weeks and to 13 to 14 g/dL by 30 weeks’ gestation (see Table 2-1 ). Hemoglobin concentration is relatively stable the last 6 to 8 weeks of gestation, with a mean concentration 16 to 17 g/dL at term. Hemoglobin may increase by 1 to 2 g/dL at birth as a result of placental transfusion. Decreased plasma volume leads to a peak in hemoglobin between 2 to 6 hours of life, with levels stabilizing by 8 to 12 hours of age.
Erythropoiesis decreases at birth, leading to a gradual decrease in hemoglobin concentration during the next several weeks of life ( Table 2-3 ). The nadir of hemoglobin concentration is reached at 8 weeks of life, with levels of approximately 11 g/dL in healthy term infants before erythropoiesis increases and the hemoglobin rises. This process is called physiologic anemia (discussed later).
Value | Cord Blood | Day 1 | Day 3 | Day 7 | Day 14 |
---|---|---|---|---|---|
Hemoglobin (g/dL) | 16.8 | 18.4 | 17.8 | 17.0 | 16.8 |
Hematocrit (%) | 53.0 | 58.0 | 55. | 54.0 | 52.0 |
Red cells (mm 3 ) | 5.25 | 5.8 | 5.6 | 5.2 | 5.1 |
MCV (fl) | 107 | 108 | 99 | 98.0 | 96.0 |
MCH (pg) | 34 | 35 | 33 | 32.5 | 31.5 |
MCHC (g/dL) | 31.7 | 32.5 | 33 | 33 | 33 |
Reticulocytes | 3-7 | 3-7 | 1-3 | 0-1 | 0-1 |
Nucleated RBCs (/mm 3 ) | 500 | 200 | 0-5 | 0 | 0 |
Platelets (1000’s mm 3 ) | 290 | 192 | 213 | 248 | 252 |
* During the first 2 weeks of life, a venous hemoglobin value below 13.0 g/dL or a capillary hemoglobin value below 14.5 g/dL should be regarded as anemic.
Cord Clamping and Placental Transfusion
The timing of cord clamping significantly influences hemoglobin values in the newborn (discussed later). Around the time of birth, placental blood is rapidly transferred to the infant. Approximately a quarter of this transfusion occurs within 15 seconds of birth and half occurs by the end of the first minute after birth. Delayed cord clamping (DCC) typically increases the infant’s blood volume by 30%, because the placental vessels contain between 75 to 125 mL of blood at birth. Holding the infant above the level of the placenta prevents placental transfusion and may even lead to neonatal transfusion into the placenta, resulting in neonatal anemia.
Site of Blood Sampling
Significant variation in hemoglobin levels may exist, depending on the site of blood sampling. Hemoglobin levels from capillary blood samples typically are higher than those obtained from indwelling venous or arterial catheters. Capillary samples may lead to overestimation of the hemoglobin, particularly when the samples are obtained from a poorly perfused extremity. The largest differences between capillary and venous hematocrits exist in very preterm infants, particularly those with acidosis, hypotension, and anemia. In infants younger than 30 weeks’ gestation, capillary hematocrit values are 20% higher than venous hematocrit values, compared with capillary values that are 12% higher than venous hematocrit levels at term. “Arterializing” a capillary sample by prewarming the extremity may improve the correlation between capillary and venous hematocrit levels. Finally, values of samples from similar sources, such as arterial, venous, and capillary samples, correlate independent of the site of sampling. For example, umbilical, radial, and femoral arterial hemoglobin levels show little variation from sample to sample.
Reticulocytes
Reticulocyte counts and the absolute reticulocyte count are elevated at birth, with values from 4% to 7% and 200,000 to 400,000/µL in term infants and 6% to 12% and 400,000 to 550,000/mL in preterm infants, respectively. In healthy infants, the reticulocyte count falls over the first few days of life to levels of 0 to 1% by day 4 of life (see Table 2-3 ). A reticulocyte count of 0 on day 1 of life indicates that there is erythrocyte under production (discussed later).
Erythrocyte Indices
Mean Corpuscular Volume
The average volume of circulating erythrocytes is estimated by the MCV. During pregnancy an inverse relationship exists between gestational age and MCV, with MCV values decreasing throughout gestation to 108 to 118 fl at term (see Table 2-1 ). When an MCV less than 95 fl is observed in a term neonate, α-thalassemia trait or iron deficiency should be considered.
Mean Corpuscular Hemoglobin
Similar to the MCV, the mean corpuscular hemoglobin is higher in preterm and term neonates, ranging from 33 to 41 pg/cell compared with 27 to 31 pg/cell in adults.
Mean Corpuscular Hemoglobin Concentration
The mean corpuscular hemoglobin concentration does not vary between neonates and adults. Although neonatal erythrocytes are larger and contain more hemoglobin, the hemoglobin in the erythrocyte is not more concentrated.
Erythrocyte Morphology
As previously noted, significant variations in neonatal erythrocyte morphology are commonly seen on peripheral blood smears, particularly in preterm infants, without intrinsic abnormalities of the erythrocyte. These variations include discocytes, bowls, echinocytes, and keratocytes (see Fig. 2-1 ).
Neonatal Anemia
Determining the cause of neonatal anemia can present a significant challenge. The differential diagnosis is extensive and includes not only many of the causes of anemia seen in older patients but also many causes unique to the fetus and newborn that are associated with pregnancy, labor, and delivery ( Fig. 2-3 ). Knowledge of these disorders and an understanding of the associated pathophysiology provide the appropriate context for the evaluation, diagnosis, and treatment of neonatal anemia. This chapter reviews anemia by etiology using the broad classifications of hemorrhage, hemolysis, and inadequate erythrocyte production.
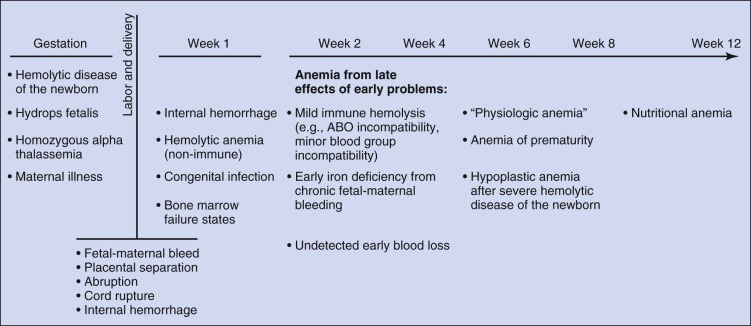
Hemorrhage in the Fetus and Newborn
Prenatal Hemorrhage
Fetal blood loss prior to birth is usually via the transplacental route, including fetomaternal hemorrhage (FMH), placental abruption, placenta previa, vasa previa, and twin-twin transfusion ( Box 2-3 ). Fetal blood loss, for instance due to intracranial hemorrhage in a thrombocytopenic infant with platelet isoimmunization or in a fetus after traumatic amniocentesis or cordocentesis, is less common.
Prenatal Hemorrhage
Transplacental Hemorrhage
Fetomaternal hemorrhage
Abruptio placentae
Placenta previa
Vasa previa
Velamentous insertion of umbilical cord
Twin-twin transfusion
Traumatic Fetal Hemorrhage
Maternal trauma
Amniocentesis
Cordocentesis
Placental Lesions
Chorioangioma
Hematoma
Hemangioma
Choriocarcinoma
Other Causes of Fetal Hemorrhage
Intracranial bleeding
Gastrointestinal bleeding
Intrapartum Hemorrhage
Placenta
Abnormalities of placentation previously listed
Surgical laceration, such as incision of the anterior placenta or cord at delivery
Umbilical Vessels
Rupture of normal cord
Rupture of abnormal cord
Aneurysm
Varix
Cyst
Funisitis with weakened vessels
Short cord
Cord entrapment, for example, by forceps
Tight nuchal cord
Occult cord prolapse
Intrapartum Neonatal Trauma
Cranial hemorrhage including subgaleal, subarachnoid, and intraventricular hemorrhage
Cephalohematoma
Splenic rupture
Adrenal hemorrhage
Liver laceration
Retroperitoneal hemorrhage
Postpartum Hemorrhage
Intracranial bleeding in the VLBW infant
Gastric or intestinal ulceration
Hemorrhage from vascular malformation
Coagulopathy
Iatrogenic causes
Phlebotomy
Tracheal mucosal tear during endotracheal intubation
Posterior pharyngeal tears during laryngoscopy or OGT placement
Vessel perforation during umbilical catheterization
Intercostal vessel laceration during thoracostomy tube placement
Gastric rupture from overdistention or OGT placement
Pulmonary hemorrhage during ventilation
Bladder mucosal tear during catheterization
Surgical wounds
OGT, Orogastric tube; VLBW, very low birth weight.
Fetomaternal Hemorrhage (FMH)
FMH is the passage of fetal blood into the maternal circulation before or during delivery. Antenatal FMH is associated with variable effects on the fetus and neonate, ranging from none to neurologic injury, hydrops fetalis, and in utero demise of the fetus or, in the neonate, congestive heart failure (CHF), hypovolemic shock, persistent pulmonary hypertension, neurologic injury, and death. The structure of the fetal-placental unit sets up a large pressure gradient from the umbilical artery to the intervillous space. The maternal intervillous space is separated from the pulsatile fetal circulation by a thin, two-cell membrane, with the constant possibility for rupture of the trophoblastic-endothelial juncture and contamination of the maternal intervillous space with fetal blood ( Fig. 2-4, A ). Given this very fragile mechanical relationship, it is surprising that significant FMH does not occur more frequently. Most spontaneous FMH occurs during the third trimester ( Fig. 2-4, B ), most commonly during labor and delivery, although hemorrhage has been reported as early as 3 to 4 months’ gestation ( Fig. 2-4, C ). Approximately 99% of deliveries are associated with FMH of less than 15 mL. However, 3 in 1000 deliveries are associated with FMH greater than or equal to 30 mL, and 1 in 2000 pregnancies is associated with an FMH greater than or equal to 100 mL.
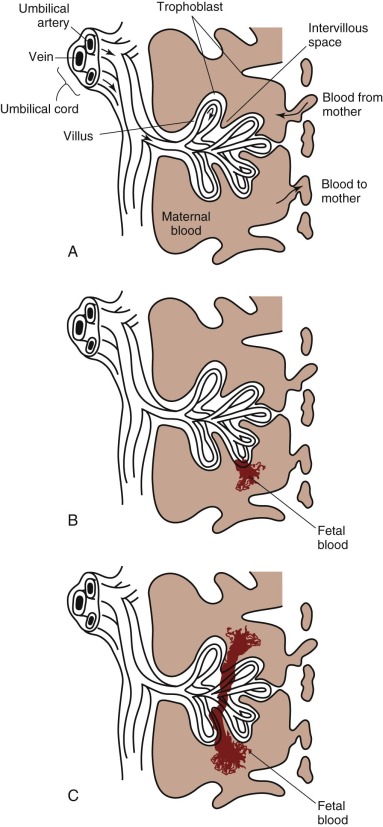
Fetal RBCs can be detected in the maternal circulation in a number of ways. The most frequently used test is microscopic demonstration of fetal RBCs in the maternal circulation with use of the Kleihauer-Betke staining procedure. With this method, HbA is denatured and eluted from smears of adult RBCs with an acid solution, leaving behind largely empty RBC membranes. Because HbF resists denaturation and elution, fetal cells stain darkly. Simple observation of the acid-treated maternal blood smear can provide a qualitative picture. The actual amount of fetal blood lost is estimated using the following formula: 2400 × the ratio of fetal to maternal cells = 1 mL of fetal blood. Using this equation, it has been shown that most deliveries result in less than 3 mL of fetal blood in the maternal circulation, and only 0.3% have a fetomaternal transfusion of 10 mL or more. However, the volume of fetal blood in the maternal circulation does not reflect the timing or acuity of the hemorrhage. Anti-HbF–based flow cytometry is an alternative method for detecting and quantifying cells that contain HbF in the maternal circulation. Conditions that elevate maternal HbF production (e.g., thalassemia minor, sickle cell disease, hereditary persistence of HbF, and pregnancy-induced maternal HbF production) confound these analyses. ABO blood group incompatibility can complicate interpretation of testing for FMH because removal of fetal cells from the maternal circulation by maternal anti-A or anti-B antibodies may lead to a false-negative result.
Abruptio Placentae
Placental abruption—that is, separation of the placenta from the uterine wall—occurs in approximately 1% of pregnancies. Abruption is more common in pregnancies complicated by chronic or pregnancy-induced hypertension. The classic triad of placental abruption is vaginal bleeding, uterine contractions, and an irritable, tender uterus. If bleeding occurs in a retroplacental location, recognition and treatment may be delayed. Fetal hypoxemia may develop as a result of decreased placenta surface area and may be worsened by fetal hemorrhage accompanying the maternal bleeding. If fetal hemorrhage is significant, severe anemia, hypovolemia, and heart failure may rapidly develop, leading to in utero fetal demise. Placental abruption is typically an acute event. However, fetal blood loss may occur gradually when the area of placental separation is small with a low-grade, chronic hemorrhage. At birth, these neonates exhibit anemia with compensation of varying degree, marked reticulocytosis, and prominent normoblastosis. In severe cases, iron deficiency may occur.
Placenta Previa
Placenta previa—that is, implantation of the placenta in a low-lying position in advance of the fetal presenting part, usually overlying part or all of the cervical os—occurs in approximately 1 in 250 births. The classic presentation is acute onset of painless vaginal bleeding, with a peak incidence around 34 weeks’ gestation. When placenta previa persists to the third trimester, a cesarean section is indicated. Fetal blood loss of varying amounts occurs in approximately 50% of cases. Signs of fetal anemia include intrauterine growth retardation, abnormal biophysical testing, and tachycardia. Vaginal bleeding may be intermittent and recurrent or massive and acute. In the former situation, the neonate presents with a partially or completely compensated anemia and marked reticulocytosis. In the latter situation, the neonate may present with acute hypovolemic shock with risk of death. Up to 10% of neonates born after the occurrence of placenta previa exhibit significant anemia, making it the most common placental anomaly that causes neonatal anemia.
Vasa Previa
Vasa previa occurs when fetal vessels exiting the placental end of the umbilical cord travel across the fetal membranes and then return to the fetal placental surface. If the fetal vessels are near the cervical os, they may tear when membranes rupture or during labor, when progressive cervical dilatation occurs. In both situations, laceration of the fetal vessels may lead to rapid exsanguination and death of the fetus. Acute onset of vaginal bleeding (usually at the initiation of labor or during the progression of labor) with acute fetal tachycardia is a classic presentation of vasa previa. Prompt recognition of this condition, delivery, and resuscitation of the anemic infant are indicated to prevent peripartum death.
Velamentous Insertion of the Cord
In cases of velamentous insertion of the cord, which occurs in approximately 1% of pregnancies, the umbilical vessels are unprotected and may tear spontaneously or during labor. Rarely the unprotected umbilical vessel crosses the cervical os, combining a velamentous insertion with vasa previa, with a high potential for tearing when the membranes rupture. Velamentous insertion is more common in twins and with low-lying placentas. The presentation of painless vaginal bleeding followed by signs of fetal distress is similar to vasa previa. The incidence of fetal loss is 1% to 2%.
Twin-Twin Transfusion and Related Disorders
Monochorionic twin pregnancies are at high risk for an adverse outcome because of vascular anastomoses that create shared fetal circulations. This situation may lead to complications, including twin-twin transfusion syndrome (TTTS), the twin anemia polycythemia sequence, the twin reversed arterial perfusion sequence, and monoamniotic twinning. TTTS complicates 8% to 15% of twin gestations with monochorionic diamniotic placentation, with the unbalanced transfer of blood from one fetus to the other via vascular anastomoses in the placenta. Classically, the donor twin is small and anemic and the recipient is large and plethoric. Fetal demise may occur from exsanguination of the donor or circulatory overload in the recipient. This condition may be dynamic with varying consequences to the twin fetuses, because the vascular anastomoses may vary in both size and number, as well as proceed in opposite directions. Antenatal factors that may predict outcome include the presence of hydrops fetalis and gestational age. Perinatal therapies have included serial amnioreduction, fetoscopic laser occlusion of communicating placental anastomoses, maternal digoxin therapy, and selective feticide.
At birth, both infants may be critically ill. The anemic donor twin may experience CHF and respiratory failure, whereas the plethoric recipient twin may require treatment for polycythemia hyperviscosity syndrome (PHS) and its complications, including thrombosis, hyperbilirubinemia, and, rarely, disseminated intravascular coagulation (DIC). In the classic presentation of TTTS in which the donor twin has been chronically hemorrhaging into the recipient, the donor anemic twin has marked reticulocytosis and normoblastosis, whereas the plethoric, polycythemic infant weighs greater than or equal to 20% more with a hemoglobin greater than or equal to 5 g/dL higher than its sibling. Acute transfusions, which typically occur closer to the time of delivery, do not lead to significant differences in neonatal weights, and there is an equal chance of either twin being the donor. Despite advances in pregnancy management, mortality in fetuses with TTTS remains high. The risk of long-term neurodevelopmental morbidity in survivors is significant.
Traumatic Fetal Hemorrhage
Fetal bleeding has been described after multiple trauma and after blunt abdominal trauma to the pregnant mother. It has also been described after traumatic amniocentesis or cordocentesis and after external cephalic version.
Placental Lesions
The marked vascularity and unique double circulation of the placenta predisposes it to numerous vascular abnormalities, some of which may lead to fetoplacental hemorrhage and/or FMH. Chorioangioma is the most common vascular anomaly of the placenta associated with neonatal anemia from fetoplacental hemorrhage and/or FMH. Chorioangiomas may number from one to many. In severe cases, they may lead to severe in utero hemorrhage and fetal demise. Other placental lesions associated with neonatal anemia are hemangioma, hematoma, and intraplacental choriocarcinoma.
Other Causes of Fetal Hemorrhage
In utero hemorrhage into fetal organs is uncommon, with intracranial and intraabdominal hemorrhage predominating. Intracranial hemorrhage typically occurs when the pregnancy is complicated by thrombocytopenia (autoimmune or alloimmune), severe fetal hypoxemia, or maternal coumadin use. Intraabdominal bleeding may occur after in utero volvulus, leading to ischemic bowel necrosis with venous hemorrhage.
Intrapartum Hemorrhage
Hemorrhage due to events around the time of delivery is classified into cases involving the placenta and umbilical cord and cases related to neonatal trauma (see Box 2-3 ).
Placenta
Labor may exaggerate or worsen abnormalities of placentation (such as vasa previa, abruptio placentae, and velamentous insertion of the cord during labor) and lead to significant hemorrhage.
Laceration of the Placenta.
An inadvertent placental incision during a cesarean section can lead to severe hemorrhage. The risk of this complication is highest when the placenta is anteriorly placed and is lacerated during an emergency cesarean section or in cases in which placentation is unknown. Lower uterine incisions have also been associated with increased risk of placental injury.
Umbilical Vessels
One in 10,000 deliveries is associated with umbilical cord bleeding or rupture, which is associated with the risk of anemia, hypovolemic shock, and death. Cord rupture is most common in precipitous deliveries. Typically, traction on a shortened or abnormal umbilical cord leads to cord rupture, usually on the fetal side. Abnormalities such as cysts, vascular malformations (including aneurysms, hemangiomas, or varices), funisitis, or a velamentous insertion can weaken the cord and predispose it to rupture. Entrapment of the cord accompanied by direct pressure, for example, by forceps, can also lead to traumatic cord laceration.
Neonates born with a tight nuchal cord can lose up to 20% of their blood volume into the placenta, leading to anemia and hypovolemic shock. In cases of a tight nuchal cord, placental blood is blocked from flowing into the fetus by constriction of the thin-walled umbilical vein, while blood flow out of the fetus into the placenta via the muscular umbilical arteries continues.
Intrapartum Trauma
Neonates may experience varying degrees of anemia as a result of internal bleeding associated with intrapartum trauma. These internal hemorrhages may or may not be obvious at the time of delivery. In severe cases, signs of hypovolemic shock are seen shortly after birth. In other cases the infant may present with sudden pallor, tachycardia, and hypotension later on the first or second day of life. The site of internal bleeding may be discerned by the intrapartum history and other individual characteristics. Marked hyperbilirubinemia may occur.
Cranial Hemorrhage.
Cranial hemorrhage is common in neonates because the head is typically the presenting part. Trauma to the scalp, the skull, or intracranial structures may result from traction applied manually—for example, by forceps or vacuum-assist devices. Cranial hemorrhage is associated with varying degrees of blood loss.
Subgaleal Hemorrhage.
Bleeding into the subgaleal space usually occurs after a difficult, instrument-assisted delivery. Trauma and/or traction tears of the emissary or “bridging” veins, which are connections between the dural sinuses and the scalp veins, leads to accumulation of blood in the potential space between the galea aponeurotica and the periosteum of the skull. The subgaleal space extends from the base of the skull forward to the orbital ridge and laterally to the temporal fascia and is thus able to accommodate significant amounts of blood. Prompt recognition of subgaleal hemorrhage, with the finding of ballotable fluid in dependent regions of the head, is critical, and close monitoring should be provided. The degree of bleeding may lead to hypovolemic shock, and exsanguination into a subgaleal hemorrhage with neonatal death has been reported. The occurrence of an extensive subgaleal hemorrhage should prompt evaluation for intracranial hemorrhage and neonatal coagulopathy.
Cephalohematoma.
In cases of cephalohematoma, the hemorrhage is confined by the periosteum and limited to the area overlying a single bone of the skull. Giant cephalohematomas may result in neonatal anemia and hyperbilirubinemia.
Intracranial Hemorrhage.
Intracranial bleeding is most frequently found after a difficult delivery, in preterm infants, and in infants with disorders of coagulation, such as platelet isoimmunization or hemophilia. Traumatic delivery leading to subdural, subarachnoid, and/or epidural hemorrhage may lead to anemia, even in term infants without coagulopathy. Preterm infants are at high risk for intraventricular hemorrhage, which may approach 10% to 15% of the total blood volume. Although signs of acute blood loss may be present, anemia is rarely the only associated clinical finding.
Abdominal Hemorrhage.
Traumatic intraabdominal hemorrhage is typically observed after a difficult delivery with prolonged traction and/or compression of the abdomen, particularly during breech extraction. Patients may present with shock, abdominal distention, and discoloration of the abdominal wall or scrotum anytime from shortly after birth to up to 10 to 14 days of life. The diagnosis is suspected on the basis of the intrapartum history and clinical course, with confirmation by abdominal imaging—usually ultrasound. Some persons advocate repeat imaging of the abdomen after 12 to 24 hours to examine whether equilibration has occurred.
Adrenal Hemorrhage.
Hemorrhage of the adrenal gland is uncommon in the neonatal period. The adrenal gland is normally enlarged in the neonate, which, combined with its vascularity, predisposes it to birth trauma and hemorrhage. Adrenal hemorrhage may also occur in neonates with coagulopathy or infection or after acute hypoxia or asphyxia. Adrenal hemorrhage has been associated with renal dysfunction, intestinal obstruction, and hyperbilirubinemia. Bilateral adrenal hemorrhage, independent of the cause, can predispose the patient to adrenal collapse and an Addisonian crisis.
Hepatic Hemorrhage.
Liver laceration, which occurs more frequently than recognized, is present in 1.2% to 5.6% of fetal and neonatal autopsies. Liver laceration may present immediately after birth or the infant may appear well for the first 12 to 24 hours of life because the hemorrhage is initially confined within the surrounding capsule. When the hepatic capsule ruptures, hemoperitoneum, abdominal distention and discoloration, and hypovolemic shock rapidly ensue. In some cases, a palpable mass in the right upper quadrant is present. Aggressive resuscitation and prompt surgical intervention have been lifesaving in affected neonates, but mortality is high.
Splenic Hemorrhage.
Splenic rupture may occur after traumatic delivery of a normal infant or after delivery of an infant with marked splenomegaly. Splenic enlargement may be due to a congenital infection or extramedullary hematopoiesis—for example, as a result of erythroblastosis fetalis. Rupture may occur at delivery or later. It has been reported to occur during exchange transfusion. Symptoms of splenic rupture are similar to those of hepatic laceration.
Postpartum Hemorrhage
Neonatal Coagulopathy
Neonatal anemia may result from marked bleeding due to abnormalities in hemostasis, particularly at intracranial and gastrointestinal sites, or after trauma, such as circumcision. Disorders most commonly associated with significant hemorrhage in the neonate include congenital factor deficiencies, particularly hemophilia, and acquired and inherited platelet abnormalities. Neonatal hemorrhage with secondary anemia has occurred in infants with vitamin K deficiency as a result of maternal anticonvulsant therapy or in exclusively breast-fed infants who were not treated with vitamin K at birth. Neonatal anemia has also been reported in infants with Wiskott-Aldrich syndrome and thrombocytopenia–absent radius syndrome.
Iatrogenic Causes
Phlebotomy-associated blood loss leading to anemia may occur in hospitalized neonates, particularly premature infants. It is not surprising that these critically ill premature infants require the most phlebotomy procedures for diagnostic testing or that they experience the most additional blood wastage (phlebotomy overdraw) during this testing. Numerous noninvasive monitoring techniques and microscale laboratory analyses have been developed to avoid these phlebotomy-associated losses. Other iatrogenic causes of hemorrhage and anemia include vascular or tissue damage during diagnostic or therapeutic procedures, accidental disconnection of vascular catheters, and postoperatively associated with operative site or wound.
Other Causes of Hemorrhage
Gastric and intestinal ulceration with hematemesis or hematochezia may cause significant anemia in the neonate. The Apt test permits differentiation of neonatal blood from swallowed maternal blood. Hemorrhage into large tumors such as sacrococcygeal teratomas and bleeding from vascular malformations such as hemangiomas, hemangioendotheliomas, and arteriovenous malformations as causes of neonatal anemia have been described.
Clinical Manifestations of Fetal and Neonatal Hemorrhage
Neonates are less tolerant of acute hypovolemia than are adults. Whereas an adult can tolerate a 10% loss of blood volume, a loss of this degree in the neonate results in significant peripheral and circulatory effects. Loss of greater than 20% of blood volume in the neonate leads to circulatory collapse, hypovolemic shock, and a high mortality rate. Symptomatology in neonatal hemorrhage is variable and is affected by the timing and amount of blood loss and the degree of resultant hypovolemia, if any ( Table 2-4 ).
Findings | Acute Blood Loss | Chronic Blood Loss |
---|---|---|
Clinical | Acute distress; pallor; shallow, rapid, and often irregular respirations; weak or absent peripheral pulses; low or absent blood pressure; no hepatosplenomegaly | Marked pallor disproportionate to distress; occasionally signs of CHF are present; pulses are usually normal; blood pressure is usually normal; hepatomegaly |
Venous pressure | Low | Normal or elevated |
Hemoglobin | May be normal initially, then drops quickly in first 24 hours | Low at birth |
RBC morphology | Normochromic and macrocytic | Hypochromic and microcytic, anisocytosis |
Serum iron | Normal at birth | Low at birth |
Course | Prompt treatment of anemia and shock required to prevent death | Generally uneventful |
Treatment | IV fluids, blood, and iron therapy later | Iron therapy, occasionally pRBCs |
Hemorrhage prior to delivery may present as anemia without signs of hypovolemia. If adequate time has elapsed from the time of hemorrhage until delivery, reticulocytosis may be present, which may be confused with a hemolytic process. However, jaundice is not prominent, blood smear morphology is not suggestive of a hemolytic process, and the Coombs test is negative, all providing additional evidence for prenatal hemorrhage rather than hemolysis.
If the in utero blood loss is chronic, the neonate may be asymptomatic or present at birth with findings of CHF, including pulmonary edema, hepatomegaly, and peripheral edema, because over time, the fetus compensates for blood loss by increasing total blood volume. If the amount of in utero blood loss has been significant but has occurred over a protracted period, the neonate may be normovolemic but pale at birth, with hypochromic, microcytic erythrocytes on a blood smear indicating iron deficiency.
Neonates with significant acute blood loss at the time of delivery are pale, tachypneic, tachycardic, and have decreased pulses and hypotension. Pallor may be striking. Differentiation from other causes of pallor in the neonate is critical because immediate resuscitation with volume replacement is indicated to prevent circulatory collapse and death ( Table 2-5 ). When the neonate is in shock, a blood transfusion is indicated. In cases of acute hemorrhage, hemoglobin levels are a poor guide to diagnosis and therapy because hemodilution has not yet occurred and hemoglobin levels may not have fallen dramatically enough to immediately indicate that crystalloid volume replacement without transfusion is adequate.
Feature | Asphyxia | Acute Blood Loss | Chronic Blood Loss | Hemolytic Disease |
---|---|---|---|---|
Respirations | Retractions, cyanosis | Rapid without effort | Little distress unless congestive heart failure | Rapid if congestive heart failure |
Arterial blood pressure | May be elevated or decreased | May be elevated or decreased | Usually normal | Usually normal |
Central venous pressure | Normal or elevated | Low | Normal or elevated | Usually normal |
Hepatosplenomegaly | Organs may be pushed down by lungs | None | Hepatomegaly if congestive heart failure | Hepatosplenomegaly |
Jaundice | Perhaps | If loss of blood into tissues (e.g., scalp) | Usually not | Yes |
Hemoglobin | Normal | Normal initially, drops after 24 h | Low at birth | Low at birth |
Red blood cell morphology | Normal | Normal/macrocytic | May be hypochromic microcytic | May have hemolytic morphology |
Other | Responds to O 2 intrauterine | Weak pulses | — | May have positive direct Coombs test or positive family history |
Supplemental iron should be provided to all infants with significant blood loss, acute or chronic, to replace lost stores.
Hemolysis in the Fetus and Newborn
Hemolytic processes are common causes of anemia in the neonate ( Box 2-4 ). As with adults, it is useful to classify hemolytic disorders as extrinsic or intrinsic. Specific hemolytic disorders are discussed in detail in other chapters. Elements specific to the fetus and newborn are discussed in this section.
Extrinsic Hemolysis
Isoimmunization
Rh sensitization
ABO
Others, such as Duffy, Kell, and Lewis
Maternal autoimmune disorders
Maternal medication use
Microangiopathic Anemias
DIC
Sepsis
Congenital infection—TORCH, malaria
Vascular—Related Causes
Kasabach-Merritt syndrome
Renal artery
Large vessel thrombosis
Severe aortic coarctation
Arteriovenous malformation
Oxidant Exposure
Other
Galactosemia
Prolonged or recurrent acidosis
Intrinsic Hemolysis
Enzymopathies
Hexose monophosphate shunt abnormality, such as G6PD
Embden-Meyerhof defect (glycolysis), such as pyruvate kinase
Other
Red Blood Cell Membrane Defects
Hereditary spherocytosis
Hereditary elliptocytosis and related disorders
Hereditary stomatocytosis
Hemoglobinopathies
α-Thalassemia syndromes
β-Globin cluster deletions
Unstable hemoglobins
DIC, Disseminated intravascular coagulation; G6PD, glucose-6-phosphate dehydrogenase; TORCH, toxoplasmosis, rubella, cytomegalovirus, and herpes virus infections.
Extrinsic Hemolysis
Blood Group Incompatibility
Immune-mediated hemolytic anemia due to blood group incompatibility is the most common cause of hemolytic anemia in the newborn period. Details of fetal and neonatal immune hemolytic disease are provided in Chapter 3 .
Other Causes of Extrinsic Hemolysis
Hemolytic anemia is present in some neonates born to mothers with autoimmune disease, particularly systemic lupus erythematosus and rheumatoid arthritis. Neonatal lupus syndrome is associated with the transplacental passage of maternal autoantibodies, including Ro/SS-A, Ro/SS-B, and La, as well as other poorly characterized contributing factors. Clinical findings may include cutaneous lesions, congenital heart block, thrombocytopenia, anemia, neutropenia, hepatobiliary disease, and pericarditis. With the exception of heart block, which is permanent, clinical manifestations, including anemia, are self-limited.
Maternal autoimmune hemolytic anemia has led to neonatal anemia of varying degree with a positive direct antiglobulin test.
Cases of maternal medication administration (including penicillin) reported to cause hemolytic anemia in the newborn have been described.
Angiopathic Anemias
Disseminated Intravascular Coagulation.
Disseminated intravascular coagulation (DIC), which is commonly associated with anemia in neonates, occurs most frequently in association with systemic infection. DIC may complicate neonatal sepsis, particularly sepsis due to group B streptococcus, Escherichia coli , or Pseudomonas aeruginosa . Congenital infections due to cytomegalovirus, toxoplasmosis, syphilis, rubella, herpes simplex, and human immunodeficiency virus (HIV) have been associated with neonatal hemolytic anemia, with or without DIC. Such cases may present a diagnostic dilemma when other causes of hemolysis are not found. Differentiating these common disorders clinically can be an aid to diagnosis ( Table 2-6 ). Neonatal enteroviral infection has been complicated by DIC and anemia. Although typically associated with hypoplastic anemia, parvovirus B19 infection may present with hemolysis as well (discussed later).
Finding | Congenital Syphilis | Toxoplasmosis (Generalized Form) | Cytomegalic Inclusion Disease | Rubella Syndrome | Erythroblastosis Fetalis | Nonimmune Hemolytic Anemia |
---|---|---|---|---|---|---|
Anemia | ++++ | +++ | ++ | ++ | +++ | ++ |
Jaundice | +++ | +++ | +++ | + | +++ | ++ |
Thrombocytopenia | ++ | + | +++ | +++ | + | 0 |
Hepatomegaly | ++++ | +++ | ++++ | ++ | ++ | + |
Splenomegaly | ++++ | ++++ | ++++ | ++ | ++ | + |
Purpura | ++ | + | +++ | +++ | + | 0 |
Skin rash | + | + | 0 | + | 0 | 0 |
Chorioretinitis | + | +++ | + | + | 0 | 0 |
Intracranial calcifications | 0 | + | +++ | 7 | 0 | 0 |
Generalized edema | ++ | + | + | 7 | + | 0 |
Additional findings | Mucocutaneous lesions; periostitis; snuffles; positive serology | Convulsions; microcephaly; hydrocephaly; positive dye test; lymphadenopathy | Pneumonia; cytomegalic inclusion cells in urine | Cataract; glaucoma; heart defects; deafness; microcephaly; hydrocephaly; bone lesion; rubella virus recoverable | Positive Coombs test; evidence of blood group incompatibility between mother and child | Morphology usually helpful |
Neonates with necrotizing enterocolitis and infection with Clostridium welchii or Clostridium perfringens have experienced the acute development of severe, rapidly progressive intravascular hemolysis. This condition may lead to hemolysis of the entire RBC mass with acute renal failure, hypovolemic shock, and death. These clostridial organisms produce several hemolytic toxins, one of which cleaves sialic acids from erythrocyte membrane glycoproteins, thus forming and exposing the Thomsen-Friedenreich cryptoantigen (or T antigen). Anti-T antibodies are present in almost all adult plasma, and thus transfusion of adult blood to a neonate lacking T antibodies can lead to life-threatening hemolysis.
Vascular-Related Causes, Including Kasabach-Merritt Syndrome.
Neonatal hemolytic anemia with microangiopathic changes has been associated with arteriovenous malformations, cavernous hemangiomas, renal artery stenosis, severe coarctation of the aorta, and large vessel thrombi. Kasabach-Merritt syndrome, a local consumptive coagulopathy with microangiopathic anemia, thrombocytopenia, and hypofibrinogenemia in association with significant vascular malformations, typically presents in the neonatal period. The coagulopathy and anemia, which may be persistent and severe, improve with regression or removal of the malformation.
Oxidant Exposure
Oxidant hemolysis in the newborn is not rare, and it is not always associated with an inherited metabolic abnormality of the erythrocyte (see Chapters 17 and 18 ) or with congenital Heinz body anemia (see Chapter 19 ). An acute hemolytic event occurs, presumably related to the oxidant sensitivity of neonatal red cells. Infants present with acute onset anemia and hyperbilirubinemia with “bite” and “blister” cells, spherocytes, and irregularly shaped RBCs on a peripheral blood smear. Typically no obvious source for the presumed oxidant stress is identified and the episode resolves in a few days. Transfusion is rarely required. Infants with ongoing hemolysis warrant further diagnostic evaluation (see Chapters 18 and 19 ).
Other Causes of Hemolysis
Other causes of neonatal hemolysis include galactosemia, prolonged or recurrent metabolic acidosis, lysosomal storage disease, and some amino acidopathies. Although typically associated with a hypoplastic anemia and thrombocytopenia, hemolysis may be found in neonates with osteopetrosis. Maternal ingestion of valproic acid has caused hemolysis and thrombocytopenia in a breastfed infant.
Intrinsic Hemolysis
Disorders of Erythrocyte Metabolism
Congenital nonspherocytic hemolytic anemia includes disorders not attributed to immune-mediated disturbances, defects of hemoglobin or the erythrocyte membrane, or other disorders. The disorders in this heterogeneous group are caused by a variety of metabolic abnormalities of the erythrocyte, including enzymopathies of glucose, glutathione, and nucleotide metabolism (see Chapters 17 and 18 ). Significant clinical, laboratory, and genetic heterogeneity exists within individual disorders. Hemolysis develops as a result of enzyme or antioxidant deficiency or impaired enzyme function.
Disorders of the Hexose Monophosphate Shunt and Associated Pathways
Disorders of the hexose monophosphate shunt or the glutathione metabolic pathways impair the ability of the erythrocyte to adequately respond to oxidant stress. In the normal erythrocyte, reduced glutathione detoxifies intracellular oxidants. In glucose-6-phosphate dehydrogenase (G6PD) deficiency, the inability to generate reduced nicotinamide adenine dinucleotide phosphate leads to inadequate levels of reduced glutathione, leaving oxidants free to damage critical erythrocyte proteins. Oxidation of the sulfhydryl groups of hemoglobin produces functionless methemoglobin and intracellular precipitates of hemoglobin called Heinz bodies.
Three clinical syndromes have been described in patients who are deficient in G6PD: neonatal jaundice (NNJ), congenital nonspherocytic hemolytic anemia, and acute hemolysis after an oxidative stress. All three have been observed in the neonatal period. NNJ, which is rarely present at birth, has a peak onset between the second and third day of life. The severity of hyperbilirubinemia is variable, but it may be severe, resulting in kernicterus or even death. Hyperbilirubinemia is usually adequately treated with phototherapy, but occasionally exchange transfusion is necessary. Anemia is rarely severe in neonates with NNJ, and when it is observed, it should prompt investigation for additional abnormalities. The cause of NNJ in neonates with G6PD deficiency is unknown. Contributions from decreased hepatic excretion of bilirubin, low-grade hemolysis, or manifestation of an additional genetic disorder have been suggested. The incidence of NNJ is increased in infants who are deficient in G6PD, with a polymorphism of the uridine diphosphoglucuronosyl transferase gene ( UGT1A ) associated with Gilbert syndrome (see Chapter 4 ).
Hemolytic anemia, even with nonimmune hydrops fetalis, has occurred in the G6PD-deficient fetus or neonate after maternal ingestion of an oxidant agent such as a sulfa drug, ascorbic acid, or fava beans. Hemolysis has occurred in breastfed, G6PD-deficient infants after maternal ingestion of fava beans. These cases have led to the term favism by proxy .
Significant hemolysis after transfusion or exchange transfusion with G6PD-deficient erythrocytes in neonates has been described. In areas endemic for G6PD deficiency, it has been recommended that blood donors be screened for G6PD deficiency prior to neonatal transfusion.
The diagnosis and treatment of G6PD deficiency is discussed in Chapter 18 .
Disorders of the Embden-Meyerhof Pathway
Defects of the Embden-Meyerhof pathway are inherited in an autosomal-recessive fashion, and typically hemolysis is only seen in homozygotes or compound heterozygotes. An exception is phosphoglycerate kinase, an X-linked disorder in which hemolysis is only seen in affected males.
Pyruvate kinase deficiency, which is the most common abnormality of the Embden-Meyerhof pathway, usually presents in the neonatal period or early childhood with anemia and jaundice (see Chapter 17 ). Severe cases have presented with anemia in utero or in the first days of life. Nonimmune hydrops fetalis has been described. Neonatal hyperbilirubinemia is common in persons deficient in pyruvate kinase, and phototherapy or exchange transfusion may be required. Kernicterus has been reported.
Other abnormalities of the Embden-Meyerhof pathway may present in the neonatal period. Glucose phosphate isomerase deficiency is the third most common hemolytic enzymopathy and is frequently manifested in infancy. Marked fetal and neonatal anemia, nonimmune hydrops, hyperbilirubinemia, and perinatal death have been described. Patients with hexokinase deficiency have experienced neonatal anemia and hyperbilirubinemia. Similarly, NNJ and anemia have been associated with 2,3-biphosphoglycerate mutase deficiency and phosphoglycerate kinase deficiency.
Disorders of Glutathione Metabolism
Deficiency of γ-glutamylcysteine synthetase and glutathione synthetase, enzymes involved in erythrocyte glutathione synthesis, has been associated with anemia in the neonatal period.
Disorders of the Erythrocyte Membrane
Disorders of the erythrocyte membrane (see Chapter 16 ) make up an important group of hemolytic anemias that may present in the fetal or neonatal period. HS, hemolytic hereditary elliptocytosis (HE), and hereditary pyropoikilocytosis are the membrane disorders most likely to present in the fetus and newborn.
Hereditary Spherocytosis.
HS frequently presents in the neonatal period with anemia and jaundice. Other findings include pallor, jaundice, tachypnea, and poor feeding. Anemia is present in more than half of patients and may require transfusion. Neonates with HS typically experience more significant hemolysis than do adults. Alterations in the neonatal intraerythrocytic environment, microcirculatory effects, and decreased membrane stability due to destabilization of the membrane junctional complex by elevated levels of 2,3-DPG all may worsen hemolysis. In addition, the shorter life span of the neonatal erythrocyte and the sluggish response of the marrow to erythropoietic stress in the first year of life contribute to the anemia. A longitudinal study of 23 infants with HS from birth to 1 year demonstrated an inadequate reticulocyte response and the need for transfusion in the first few months of life that gradually improved. Severe HS cases with in utero anemia and nonimmune hydrops fetalis have been described.
Hyperbilirubinemia, which is present in more than half of neonates with HS, occurs in the first few days of life. Levels of bilirubin may rise rapidly. Kernicterus has been described, and exchange transfusion may be required. Similar to G6PD deficiency and other inherited anemias, co-inheritance of the Gilbert syndrome UGT1A gene polymorphism may accentuate hyperbilirubinemia in neonates with HS.
Hereditary Elliptocytosis, Hereditary Pyropoikilocytosis, and Related Disorders.
HE rarely presents in the neonatal period. The exceptions are hemolytic HE and hereditary pyropoikilocytosis in which hemolysis, anemia, and jaundice may be severe, requiring treatment with phototherapy and blood transfusion. Elliptocytosis, pyknocytosis, and fragmented cells may be prominent on a peripheral blood smear. In most infants with hemolytic HE and hereditary pyropoikilocytosis, hemolysis wanes during the first year of life and typical HE proceeds to develop in the child with minimal to no anemia. Many of these patients have parents with typical HE and thus are homozygotes or compound heterozygotes for defects inherited from each of the parents. Southeast Asian ovalocytosis, a common HE syndrome in Southeast Asia and the South Pacific, usually presents with neonatal anemia and hyperbilirubinemia.
Hereditary Stomatocytosis Syndromes
The hereditary stomatocytosis (HSt) syndromes are a heterogeneous group of disorders characterized by variable numbers of stomatocytes on a peripheral blood smear and abnormal permeability of erythrocytes to sodium and potassium, with resultant modification of intracellular water content. HSt has presented in the neonatal period with severe hemolysis, anemia, hyperbilirubinemia, and hydrops fetalis. The dehydrated variant of HSt, hereditary xerocytosis, may present in utero with nonimmune hydrops fetalis unrelated to the degree of fetal anemia. Hereditary xerocytosis has been linked to mutations in the mammalian mechanosensory transduction protein PIEZO1.
Hemoglobin Disorders
Significant variation exists in the fetal and neonatal manifestations of α- and β-thalassemia because of developmental differences in globin chain synthesis. α-Globin synthesis is found in the yolk sac by 3 weeks’ gestation. By 9 weeks’ gestation, α-globin is the main α-like globin in the fetus, with only low levels of the major embryonic α-like embryonic hemoglobin, hemoglobin Portland (ζ 2 γ 2 ). In contrast, the switch from fetal to adult β-like globin chains, γ to β-globin, is not complete until 6 to 12 months of life. The primary hemoglobin in fetal and neonatal life is fetal hemoglobin, HbF (α 2 γ 2 ). Thus abnormalities of α-globin, such as homozygous α-thalassemia, lead to severe anemia in utero, whereas abnormalities of β-globin, such as homozygous β-thalassemia, are typically ameliorated in the first few months of life by sustained production of HbF. (See Chapter 21 for a detailed discussion of the thalassemias.)
α-Globin Defects
Clinical severity of the α-thalassemia syndromes is related to the numbers of functional α-globin genes present. Deletion of a single α-globin gene results in a clinically silent asymptomatic carrier state. Deletion of two α-globin genes results in α-thalassemia trait with marked microcytosis, hypochromia, and mild anemia. Deletion of three α-globin genes results in hemoglobin H (Hb H) disease (see the section on Hb H disease in this chapter) with varying degrees of anemia, ranging from mild to severe. Deletion of all four α-globin genes results in homozygous α-thalassemia, which usually leads to death of the affected fetus in utero from severe hemolytic anemia, CHF, and nonimmune hydrops fetalis.
Homozygous α-Thalassemia.
In homozygous α-thalassemia, the major hemoglobin present is a tetramer of unpaired non-α chains, γ 4 , or hemoglobin Bart’s. Survival of the homozygous fetus to late gestation depends on the presence and amount of embryonic hemoglobin Portland. In the rare case where the child is born alive, death from cardiopulmonary collapse usually occurs shortly after birth. Advances in prenatal genetic diagnosis, interventional obstetric practice, and neonatal critical care have altered the clinical course of patients with homozygous α-thalassemia. Genetic screening of at-risk populations, particularly Southeast Asians, has allowed identification of couples at risk for conceiving an affected fetus. In more than a dozen cases of homozygous α-thalassemia, careful pregnancy surveillance, in utero transfusion therapy, and postnatal transfusion therapy with iron chelation have led to prolonged survival. Concerns about congenital malformations and potential long-term neurologic effects of prolonged intrauterine hypoxia exist. Similar to some patients with β-thalassemia, bone marrow transplantation may provide a potential cure for these patients.
Hb H Disease.
Infants with Hb H disease present with hypochromic, hemolytic anemia with microcytosis and RBC fragmentation. Neonatal hyperbilirubinemia may be severe. Hydrops fetalis has been described in cases of nondeletional Hb H disease. Hemoglobin electrophoresis demonstrates hemoglobin H (β 4 ) and hemoglobin Bart’s ( γ 4 ). The differential diagnosis of neonatal microcytosis, with or without anemia, includes Hb H disease, acute-on-chronic fetomaternal hemorrhage with iron deficiency, or early presentation of α-thalassemia trait. Family history and ethnic origin (such as Southeast Asian), pregnancy history, maternal Kleihauer-Betke testing, and neonatal hemoglobin electrophoresis will clarify the diagnosis.
β-Globin Defects
As previously noted, structural variants of β-globin, including β-thalassemia and sickle cell disease, typically do not present with neonatal anemia. However, the incidence of neonatal hyperbilirubinemia in sickle cell disease is increased. In one rare case of sudden infant death in an infant with sickle cell disease, widespread neonatal vasoocclusion was observed.
γδβ and εγδβ Thalassemia.
Large deletions of the β-globin gene cluster may lead to the phenotype of γδβ and εγδβthalassemia. These patients frequently present as neonates with a hypochromic hemolytic anemia with prominent normoblastosis. Over time, the anemia improves and the erythrocyte morphology evolves to that similar to β-thalassemia trait.
Unstable Hemoglobins
Unstable hemoglobins (see Chapter 19 ) due to abnormalities in the α-globin or the β-globin chain have been reported to cause NNJ and hemolytic anemia. Precipitated globin chain fragments form Heinz bodies, thus the name congenital Heinz body hemolytic anemia . Two interesting representative hemoglobin variants that present with hemolytic anemia in the neonate due to congenital Heinz body hemolytic anemia are hemoglobin Hasharon and hemoglobin F Poole. Hemoglobin Hasharon is due to a mutation in the α-globin chain such that, when mutant α-globin chains are paired with γ-globin, an unstable hemoglobin is produced. When mutant α-globin chains are paired with β-globin, it is no longer unstable, presumably because of the tenfold higher affinity between α and β chains than between α and γ chains. Hemoglobin F Poole is an unstable hemoglobin due to mutation in the γ-globin chain. As might be expected, Hemoglobin Hasharon, hemoglobin F Poole, and other hemoglobin variants influencing HbF structure and function produce hemolytic anemia in the neonatal period that resolves as the fetal-to-adult hemoglobin switch occurs.
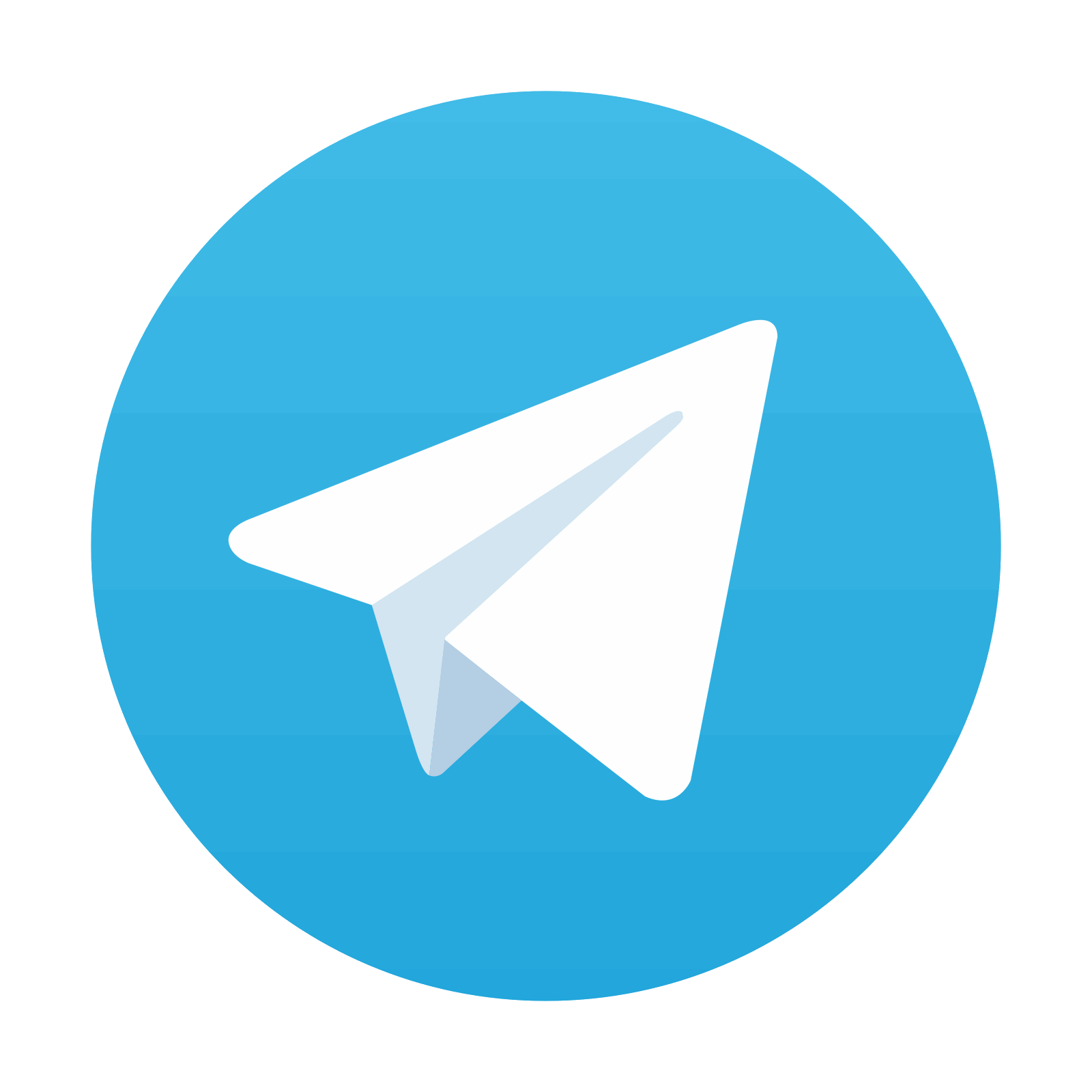
Stay updated, free articles. Join our Telegram channel

Full access? Get Clinical Tree
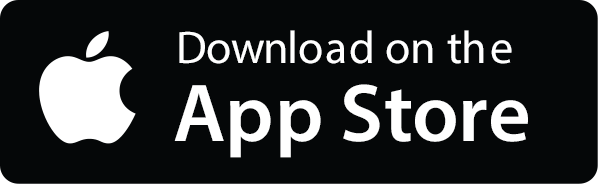
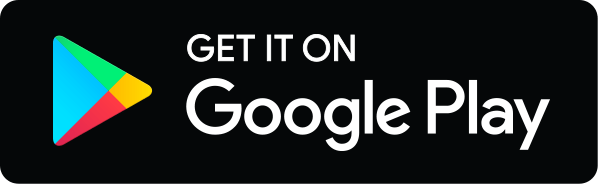