Keywords
Oncolytic virus, cancer therapy, vaccinia virus, Lister strain vaccinia virus, immunotherapy
Introduction
Vaccinia virus (VV) has played a prominent role in one of the greatest achievements in medical history—the eradication of smallpox (caused by variola virus). This occurred in the late 1970s, having claimed the lives of half a billion people worldwide in the preceding three centuries . In 1776, Jenner famously inoculated a small boy with pus obtained from a pustule on the hand of a milkmaid . The active therapeutic responsible for the resulting immunity to smallpox was most likely cowpox virus, which is closely related to but taxonomically different from VV . The origin of VV remains obscure, but by the early 20th century it became the foremost smallpox vaccine .
Although variola is no longer present in any population, threats of bioterrorism from laboratory-preserved derivatives maintain the potential need for rapid widespread vaccination. Vaccine development thus still remains active. In addition, VV has found utility in other roles, including the immunotherapeutic prevention of other infectious diseases as well as in the treatment of cancer . With regard to the latter, the earliest studies (which mainly used replication-attenuated VV recombinants for fear of toxicity) were relatively disappointing in the clinic. Replication-competent VVs retain their ability to lyse tumor cells and spread through tumor tissue. Recent advances in DNA recombinant technology (enabling the rational manipulation of the viral backbone), coupled with the ever-increasing knowledge gains in the fields of molecular virology and cancer cell biology, have aided the development of safe and efficacious replication-competent oncolytic VVs. These are currently at the forefront of the most promising novel anticancer agents.
Different VV strains were used in different areas of the world for mass vaccination. The anticancer potential of the Lister strain (VVL), which was popular in Europe, is the main focus of this chapter.
Positive Biological Features of VV Favoring Use in Cancer Therapy
VV is a member of the poxvirus family, which can be broadly divided into those that infect vertebrates (chordopox) and those that infect insects (entomopox). VV belongs to the group Orthopoxvirae, a genus of the Chordopox subdivision. Structurally, its protein core surrounds a double-stranded linear sequence of DNA that approximates 192 kbp (see http://www-ncbi-nlm-nih-gov.easyaccess2.lib.cuhk.edu.hk/genbank and http://www.poxvirus.org ) with its free ends linked by hairpin loops. This contains approximately 200 largely nonoverlapping genes, some of which are duplicated due to their position in inverted terminal repeat sequences .
The capacity of VV strains to stably accommodate up to 25 kb of exogenous DNA with little hindrance to infectivity makes it an ideal vector for use in vaccination and gene therapy . VVs have several other inherent features that make them particularly suitable for development as oncolytic agents for cancer treatment.
First, VVs have an efficient life cycle, leading to the rapid destruction of infected cells and indeed tissue via local dissemination . Viral replication occurs in juxtanuclear “factories,” commencing 1 or 2 hr following infection. During this time, host cell nucleic acid synthesis completely shuts down as cellular resources are diverted toward viral production . The initial viral replication cycle is usually complete by 8 hr, at which time the first extracellular virions are shed. Infected cells are usually lysed approximately 48 hr postinfection. Up to 10,000 viral genome copies per cell may ultimately be produced, of which half will be packaged into infectious particles .
Second, VV strains have a broad tumor cell tropism. Unlike other oncolytic viruses, cellular entry is not dependent on specific surface receptors (which would thereby limit entry only into tumor cells that express these) but appears to involve a number of nonspecific membrane fusion pathways . During its life cycle, VVs may appear in four infectious forms that differ in the number of layers and composition of their encapsulating lipoprotein membrane(s) . Cellular entry of the two major forms, IMV (intracellular mature virion) and EEV (extracellular enveloped virion), is morphologically different . The single IMV envelope fuses with the cell membrane, releasing its naked core directly into the cytoplasm, whereas the double-layered EEV particle is first engulfed by endocytosis. Its outer membrane is subsequently disrupted by the low endosomal pH following which the inner membrane is able to fuse with the endosomal membrane (and release its nucleoprotein core into the cycytoplasm).
Third, the relative molecular independence of the life cycle of VVs in comparison to other oncolytic viruses such as adenovirus makes VVs more resistant to alterations in host cell biology . Uniquely, infectious vaccinia virions are prepacked with virally encoded proteins—notably a virus-specific RNA polymerase accompanied by enzymes responsible for further mRNA processing and various early transcription factors . Viral transcription is therefore able to commence almost immediately after cellular entry. This feature ensures minimal reliance on host cellular proteins for replication—a fact that makes it difficult for the host cell to effect antiviral defensive maneuvers based on alterations in host gene transcription.
Fourth, VVs are potentially genetically safer compared with other virus families because the previously mentioned self-sufficiency enables their life cycle to be entirely cytoplasmic, with practically no possibility of host chromosomal integration.
Fifth, VVs have the potential to be efficacious following systemic administration, a feature that would enable the targeting of metastatic disease . VVs have acquired various mechanisms to evade host defenses (discussed later), whilst their different morphological forms are antigenically distinct. Following infection, the relatively stable IMVs form the majority of virion particles in a host. They are brick shaped and measure 300×200×120 nm. IMVs may be released only upon cell lysis. However, upon release, an abundance of exposed viral proteins on their single lipoprotein envelope rapidly mobilize innate and adaptive host defense mechanisms. A cell-associated virion (CEV) resembles a particle just about to bud off from the host cell and affords a means of direct cell-to-cell dissemination. If it does bud off into the systemic circulation, it maintains its additional protective host cell lipoprotein bilayer, which enables the resulting particle (EEV) to be relatively antigenically quiet . This, coupled with virus-derived transmembrane proteins that antagonize aspects of innate (complement) and adaptive (neutralizing antibodies) systemic host defense, permits widespread dissemination . Current mass viral production techniques, which depend on co-culture in a standard cell line, produce mainly IMVs. Strategies to produce proportionately more EEV forms of VV are an active area of research .
Sixth, the efficacy of oncolytic VV is not attenuated and may even be enhanced by cellular hypoxia. Advanced solid tumors often harbor areas of hypoxia secondary to outgrowth from and/or destruction of local vasculature. This contributes to the aggressive, treatment-resistant phenotype of several cancers . Hypoxia is detrimental to the efficacy of other types of oncolytic viruses . In contrast, we have demonstrated that hypoxia does not impair the replication or oncolysis mediated by VVL. Indeed, its oncolytic potency in some tumors may even be enhanced by the presence of hypoxia .
Finally, a wealth of historic clinical data, accrued mainly from its use as a vaccine, confirms the excellent safety profile of VV. Nevertheless, in the extremely unlikely event of unchecked viral replication, effective antiviral treatment options are currently available .
Cancer Selectivity
Several VVs have demonstrated tumor specificity and antitumor efficacy in preclinical models of malignancy . One advantage of using VV as an antitumor therapeutic is that certain molecular drivers of oncogenesis may also be conducive to viral replication. Many tumor cells contain mutations that inactivate common cellular defense mechanisms that act to counter both pathogens and tumorigenesis, such as defects in interferon (IFN) and apoptotic pathways . These mechanisms remain intact in normal cells, in which viral replication can therefore be inhibited. Tysome et al . compared replication of a VVL recombinant in cultured normal human keratinocytes (NHEK) with that in its malignant counterpart (SCC25). There was minimal viral replication in the former, despite the fact that it was infected with five times the dose of virus used in SCC25 cell culture.
Oncogenic mutations that result in excess stimulation of the cell cycle may also promote viral replication, mainly due to the provision of a large nucleotide pool. For example, the epidermal growth factor receptor (EGFR) pathway is overactive in many, if not all, solid malignancies . Interestingly, VV may actively drive this pathway as part of its infectious cycle: VV DNA encodes a secreted EGF homolog, vaccinia growth factor (VGF), which binds to EGFRs on neighboring cells, stimulating their replication and effectively priming them for imminent virus invasion .
Tumors usually harbor very rich but disorganized and “leaky” blood supplies . Large gaps between endothelial cells readily allow the large VV particles to leave the circulation at these junctures . Vascular permeability may be increased by elevations in temperature. Chang et al. demonstrated a 100-fold increase in uptake of systemically delivered VV by tumor following localized heat treatment. Furthermore, some VV strains have tropism for ovaries , which has been partially attributed to their relatively permeable blood supply; however, recent data obtained by our lab questions this as the sole mechanism (Wang et al. , unpublished data).
Another potential mechanism for the inherent tumor selectivity of VV may relate to the overexpression of vascular endothelial growth factor (VEGF) in tumor microenvironments. VEGF is a major effector of angioneogenesis . We have recently demonstrated enhanced VVL uptake by various solid tumor cell models in the presence of VEGF. This appears to be effected by stimulation of the Akt signaling pathway, which ultimately leads to enhanced viral endocytosis .
Investigators have attempted to improve on the natural tumor selectivity of VVs by genetic manipulation. Traditionally, strategies have relied on disrupting genes that are essential for the virus to replicate in normal cells but that are redundant for replication in cancer cells. The most popular of these has been the disruption in the gene encoding the vaccinia thymidine kinase enzyme (VTK). VTK is essential for the synthesis of deoxyribonucleotides particularly in cells with low nucleotide pools, as is the case in most normal, well-differentiated mammalian cells. Tumor cells, however, usually have an abundance of nucleotides. VTK gene-deleted recombinant VVs have demonstrated enhanced tumor selectivity in a number of in vivo tumor models . For example, disruption of the VTK gene in the neurovirulent laboratory-derived Western Reserve (WR) VV strain reduced viral colonization and replication in the mouse central nervous system following intravenous administration, without affecting tumor tropism . Tumor specificity was enhanced by a further deletion of the VGF gene . This WR double-deleted mutant (WRDD) was more tumor specific and less virulent than either the single VTK-deleted or its wild-type counterparts.
The VVL derivative from Moscow (LIVP) used by our group and others has a premature stop codon in the locus that encodes VTK (J2R) . Interestingly, near complete deletion of the J2R locus, in our case by replacing a significant section of the coding sequence with marker transgenes encoding luciferase and lacZ enzymes, further enhanced the tumor selectivity of LIVP (Hughes et al ., unpublished data).
Other candidate genes that could be disrupted include those involved in combatting host cell defense mechanisms. Numerous tumor types have defective apoptotic pathways and would therefore allow viruses harboring mutations in anti-apoptotic genes to selectively replicate in them. Specific candidates include the anti-apoptotic serpin proteins SP1 and SP2 ; F1L, which inhibits the release of cytochrome c; and N1L, a Bcl-2 homolog with additional putative functions . Although deliberate disruption of these and other genes should theoretically enhance tumor selectivity, viral replication and oncolytic potency often deteriorate in proportion to the extent of genetic manipulation . Thus, a VV in which both SP1 and SP2 coding sequences were disrupted was severely attenuated compared to viruses harboring deletions in either sequence alone . In our experience, a disruption in the J2R locus of LIVP is all that is required to strike a good balance between tumor selectivity and antitumor efficacy. For other strains, however, particularly the more virulent ones such as WR, additional genetic disruption may be required prior to potential clinical use.
Rather than disrupt the coding sequence of a gene in the viral backbone, an alternative strategy to enhance tumor selectivity is one that targets relevant post-transcriptional gene products. Hikichi et al . illustrated this principle for the first time in VV using an attenuated VVL in which the B5R gene (essential for viral trafficking and dissemination) was restored with an additional 3′ sequence that was complementary to the microRNA, Let-7a. Let-7a is commonly downregulated in tumor cells, whereas normal cells contain it in abundance. Translation of the recombinant B5R mRNA (and consequent virus production) therefore only took place in tumor cells.
Unique Characteristics of the Lister Strain VV
Different strains of VV were used in different areas of the world in the days of mass vaccination. The New York City Board of Health (NYCBOH) strain and its derivative, Wyeth, were popular in the United States, whereas Copenhagen (CPN) and Lister found prominence in Europe. Historical records trace the origin of the Lister strain to Elstree, UK (1961), where the original master stock was prepared from calf lymph and distributed to centers in France, Moscow, Tokyo, and Atlanta. Early animal studies with VVL suggested a superior safety profile, particularly with regard to neurological sequelae . During the 1970s, in Japan, an attenuated Lister strain mutant LC16m8, selected for its extremely low neurovirulence, was used to safely vaccinate more than 50,000 infants against smallpox .
During approximately the past decade, the WR strain of VV, a virulent laboratory-derived NYCBOH derivative with potent tumor lytic ability, has been the most extensively studied and genetically manipulated oncolytic VV. It is currently thought to be the most superior oncolytic strain, although this assumption was made on the basis of its replication and oncolysis in only two human cancer cell lines in the only published comparative study to date . In that study, the WR strain was selected to be rationally engineered to be more tumor selective, with preservation of its oncolytic potency (WRDD). Despite this, concerns remain regarding its safety, and it obviously lacks the advantage of previous clinical usage. Furthermore, we recently discovered that VVL15 ( Table 16.1 ) actually replicated in and lysed tumor cells significantly better than the WRDD in 11 of 14 human cancer lines tested. However, WRDD did perform better in similar in vitro analyses with murine cancer lines (Hughes et al ., unpublished data). Despite the latter, VVL15 surprisingly demonstrated significantly better antitumor potency (compared to WRDD) in several immune-competent murine cancer models (Hughes et al. , unpublished data). Given the potency of WRDD against murine cancer lines in vitro , the latter effect must be mediated by indirect mechanisms—for example, through modulation of host immunity or vascular compromise. We are currently investigating putative underlying mechanisms.
Virus Name | Disrupted Genes | Transgene Expression | References |
---|---|---|---|
GLV-1h68 | J2R (TK), F14.5L, A56R (hemagglutinin) | Renilla luciferase-GFP fusion protein (RLUC-GFP), β-galactosidase, β-glucoronidase | |
GLV-1h99 | J2R (TK), F14.5L, A56R (hemagglutinin) | Human norepinephrine transporter (into F14.5 instead of RLUC-GFP), β-galactosidase, β-glucoronidase | |
GLV-1h153 | J2R (TK), F14.5L, A56R (hemagglutinin) | Human sodium iodide transporter (into A56R instead of β-glucoronidase) | |
GLV-1h107, 1h108, 1h109 | J2R (TK), F14.5L, A56R (hemagglutinin) | VEGF single-chain antibody (GLAF-1) under different promoters, RLUC-GFP fusion protein | |
VVL-15 | J2R (TK) | Luciferase, E. coli lacZ | |
VV-TK-53 | J2R (TK) | P53, E. coli lacZ | |
VVhEA | F14.5L | Fused human endostatin and angiostatin | |
VVlacZ | F14.5L | E. coli lacZ | |
VVRG | F14.5L | RLUC-GFP | |
VV-2-12 | J2R (TK), N region | mIL-2, mIL-12, E. coli lacZ, luciferase | |
VV-mIL12 | J2R (TK) | mIL-12, E. coli lacZ | |
VV-mIL2 | J2R (TK) | mIL-2, E. coli lacZ |
Many of the open reading frames in VV are involved in viral genome transcription, replication, structure, and assembly. These are located centrally in the viral genome and are highly conserved among all orthopoxviruses. Peripherally located genes are responsible for modulation of host antiviral defense and are strain specific. The function and indeed expression status of many of these have yet to be delineated, but genes encoding proteins that interfere with IFN pathways seem to be overrepresented, indicating their significance in host defense. Although there is only an 8% difference between the genomes of Lister and other strains , phenotypic and functional differences between strains must be a consequence of these differences.
Garcel et al. sequenced a clonal isolate of a VVL substrain . The vast majority of the 201 putative VVL open reading frames (192) had more than 98% sequence homology to those previously identified in other strains, including CPN and WR strains. L172 (A53R) encodes a tumor necrosis factor (TNF) receptor homolog found in VVL but absent in WR. An extra interleukin (IL)-18 binding protein gene (L013) is again present in VVL but missing in the WR strain.
A genomic comparison between Lister, CPN, and WR strains conspicuously revealed a short sequence encoding completely different proteins in the Lister genome . Part of this sequence (L196) bears resemblance to that encoding a transmembrane Bax inhibitor motif containing protein found in various mammalian species and therefore implicates a historic recombination event with mammalian DNA . L196 encodes an apoptosis inhibitor . Another unique gene in this region that is missing CPN and WR strains encodes a TNF binding protein (L195) . Furthermore, its neighboring gene, L194 encodes a truncated version of a type 1 IFN binding protein that is intact in the WR strain. The alternative sequence in the corresponding section of CPN and WR genomes includes an open reading frame encoding an IFN-α/β binding protein (B19R/WR200). There is also a different anti-apoptotic gene, WR195, which encodes the serpin protein SPI-2 (an IL-1β converting enzyme inhibitor leading to the inhibition of FAS-mediated apoptosis).
VVL therefore codes for at least two extra TNF binding proteins (L172 and L195) in comparison to WR and CPN strains and could thus be better armed against the host TNF response. On the other hand, it lacks at least one IFN binding protein gene (WR200). There are also qualitative differences in genes encoding anti-apoptotic proteins.
Interestingly, the gene encoding the semaphorin-like protein L156 (A39R), which appears to stimulate monocytes and enhance production of proinflammatory cytokines , is frame-shift mutated in the WR strain. That this plays a role in the immunogenicity of VV is dramatically demonstrated by the induction of systemic inflammation and pulmonary edema in mice administered a WR recombinant expressing this transgene.
During the past fifteen years, the most commonly reconstructed Lister variant for the purposes of antitumor therapy has been the Moscow derivative, LIVP. The WR VV is particularly neurovirulent, reflecting its origin from multiple passages through the murine brain . Although systemic inoculation with a VTK gene-deleted WR virus reduced viral recovery from off-target organs, titers in these organs were still significantly higher in comparison to that with LIVP . In one study, there was no viral recovery from the brain or ovaries of nude mice following an intravenous dose of 1×10 7 plaque-forming units (PFU) of VVL . Other organs also had a higher affinity for WR VV compared to VVL. Pulmonary titers were consistently higher at any given time-point following intranasal inoculation of similar doses of viruses . Indeed, as little as 5×10 5 PFU of intranasally inoculated WR caused severe signs of toxicity (including significant weight loss) and 50% death in immune-competent BALB/c mice . Similar toxicity was only observed with VVL if administered in doses 100-fold higher .
Although difficult to generalize, the reduced persistence and virulence of VVL in immune-competent animal hosts implies the stimulation of a more potent “primary” antiviral immune response in comparison to WR VV. The implications of this enhanced immunogenicity with regard to antitumor efficacy have yet to be delineated. Further characterization of the expression and functional aspects of individual VV genes will enable the rational engineering of more selective, safer, and potentially more effective recombinants. Table 16.1 outlines the range of VVL constructs that we and other groups have used as putative anticancer therapeutics. Details of these will follow in the remainder of this chapter.
Lister Strain Derivatives with Demonstrated Antitumor Efficacy
In general, VV and other oncolytic viruses mediate their antitumor effects via direct and indirect mechanisms. These may be broadly classified as a consequence of (1) direct viral oncolysis, (2) local vascular destruction, and/or (3) focusing of the effector host immune defenses (both innate and adaptive) into the tumor microenvironment. All of these mechanisms may be augmented by incorporation of relevant therapeutic transgenes into the viral backbone.
As with other strains, VVL derivatives have demonstrated tropism for a variety of different tumor types both in cell culture and in animal models. The latter have predominantly been xenograft models of human malignant solid tumors, including breast , liver , prostate , bladder , squamous cell , pancreatic , thyroid (anaplastic) , and mesothelioma .
Ascierto et al . investigated whether molecular signatures within a particular tumor cell could predict permissivity of infection by the Lister strain recombinant GLV-1h68 ( Table 16.1 ) . They screened the NCI 60 panel of human cancer cell lines , which covers a broad spectrum of human malignant cancers. Three genes were found to be consistently upregulated in permissive versus nonpermissive lines: GDF15, a member of the TGF superfamily that regulates inflammatory and apoptotic pathways; CD9, a transmembrane protein that regulates cell growth, activation, and mobility; and integrin β 5 , which plays a role in cell movement and adhesion. In contrast, far more genes were conspicuously downregulated and could be grouped into those involved in NF-κB, TGF-β, or IFN-α/β signaling and/or the activation of the RNA polymerase complex. In other words, the most permissive cell lines to GLV-1h68 had predampened host cell antiviral defense mechanisms and host gene transcription.
Chen et al . demonstrated a positive correlation between the replication efficiency of VVL derivatives in tumor cell culture and antitumor efficacy in the corresponding xenograft model. Although the latter largely reflected higher titers of replicating virus and thus oncolysis, they also postulated a significant contribution from the innate immune system. Indeed, expression profile analysis revealed upregulation of characteristic “innate” cytokines, chemokines, and growth factors in tumor tissue associated with a cellular infiltrate consisting of dendritic cells, monocytes, and neutrophils . Thus, even in these immune deficient models, mechanisms additional to viral oncolysis contributed to antitumor efficacy. It is these latter mechanisms that will require maximizing as viral oncolysis is likely to be curtailed in immune competent models and human patients.
VVL as a Gene Therapeutic Platform
The unique properties of VV outlined previously make it particularly appealing for use as a putative anticancer gene therapeutic vector. This has traditionally entailed the delivery of replication-impaired viruses engineered to express and thus restore dysfunctional tumor suppressor genes in cancer cells. A VVL recombinant in which a TP53 transgene replaced the VTK gene locus was rendered replication inactive by mild psoralen and UV treatment. It successfully infected tumor cells in culture and induced cell death mediated by apoptosis in cell lines with dysfunctional TP53 . However, in vivo , it was only effective following ex vivo infection of tumor cells prior to their subcutaneous implantation. Its replication-competent counterpart, however, demonstrated superior tumor growth inhibition compared to the control virus in a preestablished xenograft model—an effect that was further enhanced by combination treatment with radiotherapy . Similarly, in a syngeneic orthotopic model of bladder cancer, instillation of the same replication-competent VVL-TP53 recombinant decreased tumor incidence and improved survival compared to the control virus .
Vectors that are replication competent and/or at least produce a secreted therapeutic product (which may effect a “bystander” action against neighboring uninfected cells) are best suited to cancer gene therapy. However, even with a potent replicating virus such as VV, several barriers in the tumor microenvironment may prevent spread and transduction of all tumor cells, which in themselves will be genetically heterogeneous . The latter in particular means that restoring the function of a single gene is unlikely to succeed in curbing tumor growth.
Enhancing VVL-Mediated Compromise of the Local Tumor Vasculature
Tumor angiogenesis is one of the hallmarks of malignancy . All cells must be within 200 μm of a blood vessel (the limit of diffusion of oxygen) for survival. Solid tumors thus need to be highly vascularized, and this is generally mediated by an imbalance between endogenous pro- and anti-angiogenic factors favoring the former . Soluble growth factors such as VEGF and fibroblast growth factor-β are key molecules in this regard that are overexpressed by many tumor cells. However, the resulting neovasculature within a tumor is often poorly organized, with patchy areas of collapse and leakiness. As previously described, this leakiness may contribute to the tumor selectivity of the relatively large vaccinia virions. Furthermore, VV may directly interfere with the local tumor vasculature. Kirn et al. demonstrated that a WR recombinant infected and destroyed tumor-associated vascular endothelial cells . We have also witnessed this phenomenon with a VTK gene-deleted VVL derivative (VVL15). In addition, as described previously, the infectivity of VVL15 for tumor cells appears to be enhanced by the presence of VEGF (Hiley et al. , unpublished data). In contrast, GLV-1h68 appears not to infect intratumoral endothelial cells . Instead, it was associated with an enhancement of vasodilation and permeability of the peritumoral vasculature .
Tysome et al. demonstrated that LIVP armed with a gene encoding a fusion protein comprising two potent endogenous angiogenesis inhibitors, endostatin and agiostatin (VVhEA), selectively inhibited tumor angiogenesis in a xenograft pancreatic cancer model . This was associated with enhanced antitumor regression and animal survival, an affect that was reproduced in a similar model of head and neck cancer . Similarly, Frentzen et al. created a recombinant Lister strain virus based on the GLV-1h68 platform in which a single-chain antibody against both human and murine VEGF (GLAF-1) replaced the VTK gene locus (GLV-1h108) . This also demonstrated enhanced antitumor efficacy in xenograft models of human lung and prostate cancer; again, efficacy was associated with a significant reduction in microvessel density. Interestingly, in this study, the backbone virus GLV-1h68, when combined with the licensed monoclonal anti-VEGF-A inhibitor Avastin, demonstrated a regression pattern similar to treatment with GLV-1h108 alone. Stabilization of the haphazard tumor vasculature by independent angiogenesis inhibition may therefore aid oncolytic viral delivery. VV may in turn be mutually beneficial to drug delivery by decompressing and “normalizing” preexisting intratumoral vessels .
Enhancing the Immunotherapeutic Properties of VVL
A perceived disadvantage for oncolytic virotherapy with VV and other viruses is their ability to effectively stimulate both innate and adaptive host antiviral immune defense mechanisms. In an immune-competent host, the virus may be expected to be cleared before it can effect complete tumor eradication and a multidose regime would not be possible. This would certainly be problematic for any regime that relied solely on viral oncolysis for antitumor efficacy. However, we and other investigators consider that it is the immunogenicity of oncolytic viruses that may ultimately prove to be the key to their success. If VV could cross-prime an antitumor immune response, then infection and lysis of every single tumor cell would no longer be necessary.
The most favorable adaptive response for the successful eradication of intracellular pathogens such as viruses is one orchestrated by Th1 CD4 + T cells and effected predominantly by cytotoxic CD8 + T cells . If tumor cells were infected with a virus in a host already immunized to it, then the rapid expansion, mobilization, and infiltration of virus-specific memory CD8 + T cells into the tumor should inadvertently supplement viral oncolysis. Hu et al. demonstrated that intratumoral injection of adeno- or vaccinia virus in hosts preimmunized against these led to enhanced tumor shrinkage and greater infiltration of lymphocytes into the tumor compared to naive hosts . We have also observed a similar phenomenon with VVL15. Unfortunately, this strategy relies on the infection of every tumor cell population, which for the treatment of widespread metastases necessitates systemic delivery—a feat that may be thwarted by several barriers, particularly in preimmunized hosts .
In addition to redirecting an antiviral effector immune response, viral oncolysis could provide the backdrop to directly prime host tumor-specific immunity. However, tumor antigens resemble self, and tumor cells often downregulate antigen-presenting pathways (e.g., the MHC class I complex) . In addition, outside of the tumor cell, the surrounding microenvironment is often actively immunosuppressed by various mechanisms .
Matzinger formulated the concept that the prime role of the immune system is to respond to cellular and tissue distress as opposed to non-self and that the presence of certain “danger signals” may even reverse tolerance to self-antigens . Viral oncolysis releases potentially antigenic tumor-specific peptides from the relative safe haven of an intact tumor cell. These will be scavenged by professional antigen-presenting cells (APCs). Damaged cells will also release “danger” signals such as DNA, RNA, uric acid, and heat shock proteins . Both cellular- and pathogen (viral) -related “danger” signals stimulate APCs to optimally process and present their scavenged antigens to the adaptive immune system .
Components of the innate immune system may also play an effector role in the virotherapy of cancer. As previously described, the VVL recombinant GLV-1h68 selectively colonizes a range of human xenografts in nude mice following intravenous administration. In these models, gene expression profiling has consistently revealed a positive correlation between tumor eradication and host expression signatures representative of “innate defense” activation . These include IFN-stimulated genes (e.g., STAT 1 and IRF 7); certain chemokines and their receptors (e.g., IP10); and cytokines that include IL-1, IL-6, IL-15, and IL-18. A peritumoral infiltrate of mononuclear cells was also a feature of virally treated tumors. In contrast, other studies question the antitumor contribution of the innate immune system, which instead appeared to limit viral replication and oncolysis. For example, cyclophosphamide depletion of leukocytes prior to the administration of GLV-1h68 into a human breast tumor xenograft model actually enhanced tumor eradication . We have also demonstrated enhanced uptake of intravenously administered VVL15 by established tumors in both nude and immune-competent mice following selective, temporary monocyte depletion (Ferguson, unpublished data).
The role of the host immune system in VV-mediated antitumor clearance is thus complex and is likely to vary with viral strain, tumor type, and host. For example, Wang et al . demonstrated equivalent antitumor responses following intratumoral administration of a VV WR recombinant into two different syngeneic subcutaneous murine lung cancer models . Viral recovery from one of the tumors (TC-1) was high. In this model, viral oncolysis played the dominant antitumor role because alteration of the immune competence of the host made little difference to antitumor efficacy. In contrast, viral recovery from the other model (LKRM2) was poor; here, depletion of CD8 + T cells completely reversed tumor eradication.
VVL-Mediated Enhancement of Antitumor Immunity
A virus that strongly elicits a specific CD8 + T cell response would be expected to provide the best chance to cross-prime a favorable antitumor CD8 + T cell response. Highly replication-competent viruses promote better CD8 + T cell responses than their attenuated and nonreplicative counterparts . In a comparison study that included Lister and NYCBOH derivatives, wild-type WR virus demonstrated the strongest level of replication in animal models and also elicited the strongest CD8 + T cell responses following both intraperitoneal and intranasal infection . This phenomenon was attributed to the engagement of the costimulatory receptors, OX40 and CD27 (both TNF receptor family members), on T cells, which was only observed with the more virulent strains and recombinants of VV.
Replication potency of viruses in the context of tumor therapy, however, must be weighed against toxicity, particularly in view of the likely need to use relatively high doses in patients with advanced cancer. The WR strain persists significantly longer in off-target organs such as lungs and ovaries and afflicts considerable toxicity in both immune-competent and particularly nude animal hosts . In contrast, as mentioned previously, the Lister strain is cleared more than two times faster and is 100-fold less toxic following intranasal administration.
In an attempt to enhance their ability to cross-prime antitumor immunity, VV and other OVs have been armed with transgenes coding for various combinations of tumor antigens and/or immunomodulatory molecules. Regarding the former, vaccinating against a single tumor antigen is unlikely to be successful, especially because of the vast genetic heterogeneity (thus antigenic heterogeneity) exhibited within a particular tumor. The ideal aim would therefore be to vaccinate against multiple tumor antigens, tailored to the individual. This could be achieved by viral oncolysis (which should release an array of patient-specific tumor antigens); possibly boosted by concurrent immunomodulatory transgene expression. A wide variety of VV recombinants incorporating various different transgenes have been used as putative anticancer therapeutics, including those coding for T cell costimulatory ligands—for example, B7.1 , CD40L , and cytokines such as IFNs , granulocyte–macrophage colony-stimulating factor (GM-CSF) , IL-2 , and IL-12 . In the main, the goal of this therapeutic strategy is to nurture a Th1, cytotoxic antitumor effector response .
Chen et al . engineered a group of recombinant VVLs armed with transgenes encoding IL-2, IL-12, or both . Recombinants expressed high levels of cytokines in vitro and in vivo in a subcutaneous xenograft rat glioma (C6) model. Antitumor efficacy was significantly better with all constructs in comparison to the unarmed control virus following low-dose intratumoral injection. Because nude mice were utilized, this surplus effect was likely to have been mediated by tumor-focused activation of innate immunity, evidenced by localized increases in IFN-γ and natural killer cells in all experimental arms.
Strategies to enhance the immunogenicity of the virus are expected to enhance viral clearance in immune-competent hosts. This indeed has been observed in vivo with VVs armed with some of the aforementioned immunomodulatory genes . Furthermore, even with an antitumor vaccination protocol (mediated by viral vectors), secondary “booster” doses of virus are likely to be necessary. However, this situation necessitates the delivery of only a small quantity of virus into residual tumor tissue to effect killing of some, but not all, tumor cells (releasing the tumor antigens required for boosting putative tumor-specific memory T cells). Given in relatively high doses, VV colonization, replication, and transgene expression in solid tumor has been demonstrated following systemic administration in both animal models and patients, even in the face of preexisting neutralizing antibodies .
A problem with using the same virus to provide a secondary immune boost against tumor antigens is that viral antigens are likely to be significantly more antigenic. As a consequence, a secondary immune response against viral antigens may swamp that against weaker tumor antigens. An alternative strategy that holds much promise is to use an antigenically different oncolytic virus for the secondary boost. Release and presentation of tumor antigens following administration of the second virus (to which the host is naive) should stimulate a secondary antitumor immune response that dominates the primary response to the new virus . Bridle et al. used such a heterologous prime-boost strategy to treat an aggressive intracranial syngeneic B16-F10 murine melanoma model . They demonstrated that intramuscular administration of a recombinant adenovirus armed with a transgene encoding a melanoma antigen (DCT), followed later by an intravenous dose of recombinant vesicular stomatitis virus (VSV) armed with the same gene, led to a significant increase in median survival compared to therapy with either virus alone or indeed with the same combination but with viruses lacking the DCT transgene. The heterologous regime was associated with a significantly enhanced percentage of anti-DCT CD8 + T cells. Importantly, there was a corresponding reduction in the anti-VSV CD8 + T cell response compared to that of a naive mouse infected with the VSV recombinant. In a similar manner, Zhang et al. used sequentially presented Semliki Forest and vaccinia viruses to enhance antitumor efficacy against a disseminated ovarian cancer model . Again, the best results were observed when viruses were armed with a transgene encoding a common tumor antigen.
We recently demonstrated complete eradication of a syngeneic subcutaneous pancreatic tumor model in a hamster host using a heterologous prime boost regime with a recombinant adenovirus followed by a VVL recombinant. Unlike in the previously mentioned studies, these were not armed with a tumor antigen-encoding transgene . The effect was abrogated with use of virus alone or if virus administration was reversed. Importantly, this strategy generated antitumor immune memory, evidenced by no tumor regrowth following subsequent subcutaneous inoculation of the same tumor cells. T cell subset depletion experiments confirmed the critical role of the adaptive immune system. Our study illustrated that a heterologous prime-boost regime tailored to whole tumor cells (as opposed to a single tumor antigen) is indeed possible in the face of two antigenically different but strongly oncolytic viruses administered in a favorable sequence.
Combination with Cytotoxic Agents to Enhance the Antitumor Efficacy of VVs
A population of tumor cells is usually genetically heterogeneous and will often harbor cells resistant to a single treatment modality. Although VV as an antitumor therapeutic operates through multiple mechanisms , it is unlikely that every single tumor cell will be infected and lysed. We have discussed how harnessing host immunity may aid in this regard. Combination with other cytotoxic treatment modalities may also be more likely to destroy residual tumor. In any case, novel oncolytic viruses will likely be introduced into clinical trials alongside established therapies.
Cisplatin and gemcitibine are commonly used for the treatment of advanced pancreatic cancer. Cisplatin binds to and cross-links double-stranded DNA. Gemcitibine is a nucleoside analog that terminates DNA strand extension and thus replication. Both work by ultimately triggering apoptosis. These have been used in a pancreatic xenograft model in conjunction with GLV-1h68 . Both demonstrated synergistic antitumor efficacy, although in the gemcitabine combination study, the final tumor size in the “virus only” group eventually “caught up” with that of the combination group. Purported mechanisms for this synergy include alterations in nucleotide pools, DNA repair pathways, and apoptosis. As noted previously, changes in the local tumor vasculature associated with viral infection may have improved delivery of drug into the tumor microenvironment. Although synergism was demonstrated in this particular study, because chemotherapeutics often target nucleotide pools and DNA replication, they may have an inadvertent effect on the viral life cycle.
Tumor-selective oncolytic viruses armed with transgenes may be used to locally deliver high concentrations of their translated product into the tumor microenvironment. They have been used to deliver enzymes that mediate the conversion of a relatively safe prodrug into a highly potent cytotoxic one that, being restricted to the tumor, should produce minimum systemic toxicity . One advantage of doing this is that not all cells need to be infected because the active toxic drug may spill over onto neighboring uninfected tumor cells, causing a ”bystander effect.” Replication-competent VVs armed with the transgene for the cytosine deaminase (CD) enzyme, which converts 5-fluorocytosine (5-FC) into the active metabolite 5-fluorouracil (5-FU), have demonstrated efficacy in vivo . Seubert et al . employed a different prodrug/enzyme system in conjunction with the GLV-1h68 recombinant . GLV-1h68 contains a lacZ transgene that encodes β-galactosidase. The prodrug in this case was derived from a seco analog of the highly cytotoxic natural antibiotic duocarmycin and activatable by β-galactosidase. Systemic addition of prodrug enhanced the antitumor effect of GLV-1h68 in a xenograft model of human breast cancer. Viral replication was not affected by either inactive or active metabolites, in contrast to a study in which a VV CD/5-FC system was used .
In a similar manner to combination treatment with chemotherapeutics, several preclinical studies have demonstrated mutual antitumor synergy afforded by combination of oncolytic virotherapy with radiation . Most such studies, however, were conducted with adeno- and herpes viruses. Timiryasova et al. demonstrated that a p53-ve glioma xenograft was more sensitive to a replication-competent VVL armed with a TP53 transgene in conjunction with radiotherapy compared to either treatment alone . As predicted, this effect was mediated by a superior level of apoptosis .
VVL has also been armed with transgenes coding for receptors or transporters for radionuclides, which may therefore be localized to the tumor microenvironment . Indeed, such a strategy may even render traditionally radioresistant tumors more responsive. Hence, Gholami et al. used a GLV-1h68 VVL backbone armed with the human sodium iodide symporter to effect uptake of radioiodide tracer by human anaplastic thyroid cancer (ATC) cells . ATC tumors are usually radioiodide resistant due to lack of the sodium iodide symporter on their cell membranes .
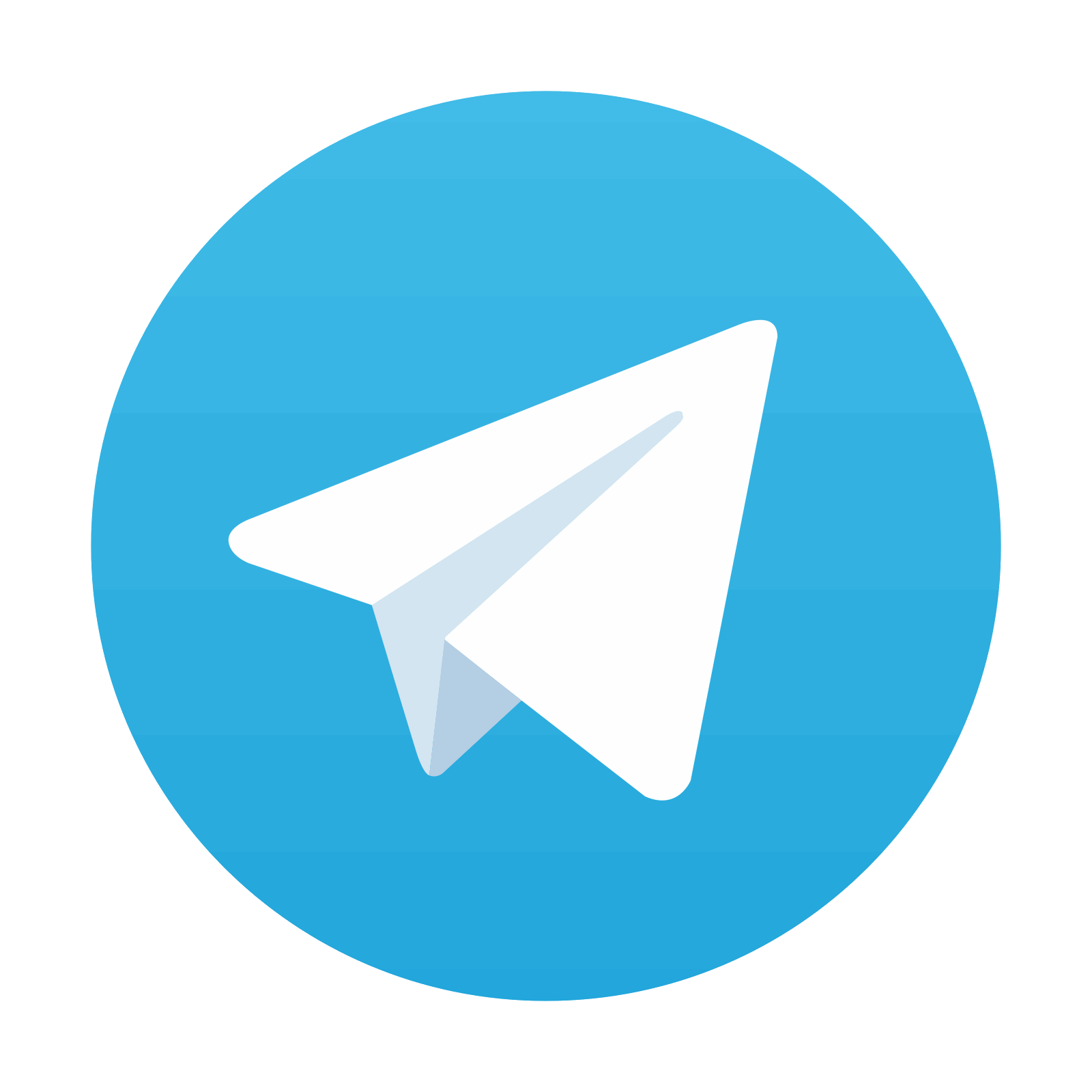
Stay updated, free articles. Join our Telegram channel

Full access? Get Clinical Tree
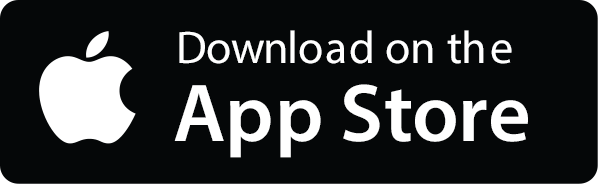
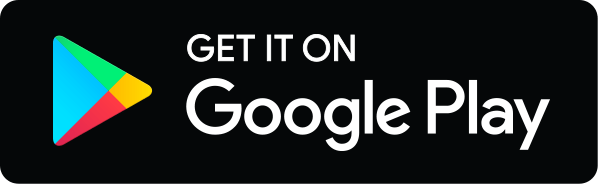