FIGURE 1.1
The apoB48 lipoprotein particle family consists of chylomicrons and chylomicron remnants (CR). Chylomicrons are secreted by intestinal cells following a meal and transport the dietary lipids to target tissues within the body. Chylomicron particles are too large to penetrate the arterial wall with any facility and so accumulation of chylomicrons does not increase the risk of vascular disease. However, large numbers of intact chylomicron particles do increase the risk of pancreatitis. CR are chylomicron particles from which most of the triglyceride has been removed by adipose tissue and muscle but which still contain almost all the cholesterol. CR are normal particles present in small numbers in plasma, even in the postprandial period, because they are so rapidly removed by the liver.
In remnant lipoprotein disorder, the removal of the normal CR particles is markedly delayed. Accordingly, large numbers of CR particles accumulate in plasma – 10 to 20 fold more than normal – and with their prolonged half-life in plasma, these become enriched in cholesterol. Unquestionably, atherogenic risk is markedly increased in this disorder and rapid, accurate diagnosis is one of the major practical advantages of the apoB algorithm. Whether atherogenic risk is significantly increased when CR removal is only slightly delayed and the number of remnant particles is only slightly increased remains controversial. In these situations, there are, on average, 10 times more very low-density lipoprotein (VLDL) particles than CR particles and generally 9 times more low-density (LDL) particles than VLDL particles.
The family of the apoB100 lipoprotein particles consists of VLDL, intermediatedensity lipoprotein (IDL), LDL and Lp(a) particles. Each contains one molecule of apoB100. As will become obvious, apoB100 lipoprotein particles are much more important in atherogenesis than apoB48 lipoprotein particles because the number of apoB100 particles, almost always, is overwhelmingly greater than the number of apoB48 particles. For example, as just noted above, at the peak of the postprandial period, even in patients who are hypertriglyceridemic, with the prominent exception of those with remnant lipoprotein disorder, there are approximately 5 to 10 times more VLDL apoB100 particles than apoB48 particles. Accordingly, for convenience and in accord with usual practice, we will use apoB and apoB100 interchangeably since virtually all the apoB particles present in plasma, with the prominent but unusual exception of remnant lipoprotein disorder, are apoB100 lipoprotein particles.
1.1.2 Anatomy of the ApoB48 and ApoB100 Lipoprotein Particles
The major structural features of the apoB48 and the apoB100 lipoprotein particles are illustrated in Figure 1.1 . Each particle has a coat and a core. Most of the lipids within the particle – cholesterol ester and triglyceride – are present within the core and the differences in size of the particles are due to differences in the mass of core lipids. The relative proportion of the core lipids differs and these differences are also characteristic of the different apoB lipoprotein particles.
For the chylomicrons and CR particles, the coat consists of a phospholipid monolayer into which an apoB48 molecule is intercalated, whereas for VLDL, IDL and LDL particles, the coat consists of a phospholipid monolayer into which one apoB100 molecule is intercalated. ApoB48 and apoB100 wrap around the outer phospholipid monolayer of the lipoprotein particles in which they are found and by doing so provide a structural backbone for the particle. Because there is only one apoB48 molecule per particle, the number of chylomicrons and CR is equal to the plasma concentration of apoB48. Similarly, the sum of VLDL, IDL, LDL, and Lp(a) – an LDL particle to which a molecule of apo(a) has been attached – is equal to the plasma apoB100.
Because apoB100 and apoB48 are critical to the architectural structure of the particle, in contrast to the many other apolipoproteins that may be present, or to the core lipids, they are fixed constituents of the particle during its entire lifetime. Free cholesterol has a limited solubility in phospholipid membranes and that is where it is found in the coat of the lipoprotein particles. By contrast, cholesterol ester – that is, cholesterol to which a fatty acid has been joined – is virtually insoluble and immiscible in water and accordingly is found within the core of the particle. This also applies to triglyceride, which is also insoluble and immiscible in water.
Although apoB48 and apoB100 are the signature apolipoproteins, a series of other apolipoproteins including apoE, apoCI, apoCII, apoCIII, apoAI, apoAIV, apoAV are scattered on the surface of chylomicrons and VLDL. Some of these are present when the particle is secreted from its site of synthesis while others are transferred from other lipoprotein particles during their lifetimes in plasma. Many influence critical steps in the metabolism of the lipoprotein particles whereas the function(s) of others remains unknown. In contrast to apoB48 and apoB100, these apolipoproteins are not fixed constituents of the particles but attach and detach relatively freely during their lifetime.
Much remains to be learned about the function of these apolipoproteins. ApoCIII, in particular, seems to be an important determinant of VLDL metabolism and there is considerable evidence linking increased apoCIII to increased cardiovascular risk.7,8It remains uncertain, however, whether this association is independent of any effect of apoCIII on VLDL or LDL particle number. Even if it were not, as is not unlikely, that would not diminish the significance of apoCIII as a potential therapeutic tool to manipulate VLDL or LDL particle number.
The major components of apoB48 and apoB100 particles are summarized in Table 1 .9
Table 1.1
Major Components of ApoB48 and ApoB100 Particles
Chylomicron | VLDL | IDL | LDL | |
---|---|---|---|---|
Density (g/ml) | ~ 0.93 | 0.93-1.006 | 1.006-1.019 | 1.019-1.063 |
Diameter (nm) | 75-1200 | 30-80 | 25-35 | 18-25 |
Surface components | ||||
Total Apolipoprotein content | 1-2% | 8% | 19% | 22% |
ApoB48 | + | – | – | – |
ApoB100 | – | + | + | + |
ApoCI | + | + | + | – |
ApoCII | + | + | + | – |
ApoCIII | + | + | + | – |
ApoE | + | + | + | – |
ApoAI | + | + | – | – |
ApoAIV | + | + | – | – |
ApoAV | + | + | – | – |
Surface Lipids | ||||
Cholesterol | 1-3% | 7% | 9% | 8% |
Phospholipids | 7-9% | 18% | 19% | 22% |
Core Lipids | ||||
Triglycerides | 85-90% | 55% | 23% | 6% |
Cholesterol Esters | 1 – 3% | 12% | 29% | 42% |
1.1.3 The ApoB48 Lipoprotein Particles: Chylomicrons and Chylomicron Remnants
Physiological Role
The triglyceride and cholesterol within the food we eat are absorbed by the microvillar cells of the intestine, within which they are assembled into nascent chylomicron particles, which are secreted into the lymph and then into plasma, and within which they are delivered via the circulation to selected sites within our bodies. The amount of each of these major lipids that we ingest per day varies and is determined by the wealth of the society in which we live, our individual wealth within that society, and the dietary culture of our society. The mass of triglycerides always far exceeds the mass of cholesterol. On average, in affluent Western cultures, we ingest about 100 g of triglycerides versus 500 mg or less cholesterol per day. Triglycerides provide us with fatty acids and fatty acids are one of our principal sources of energy (Figure 1.2 ).
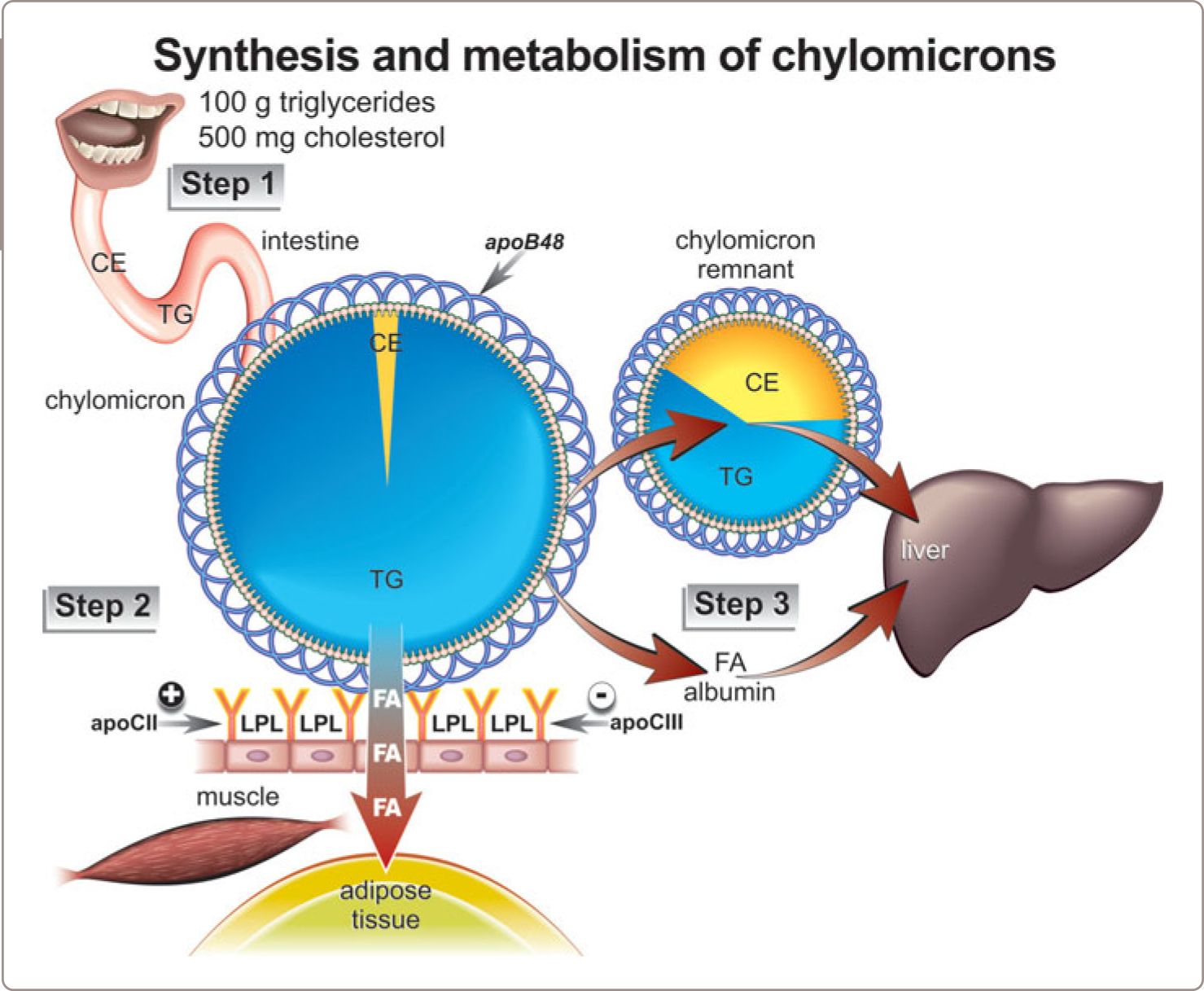
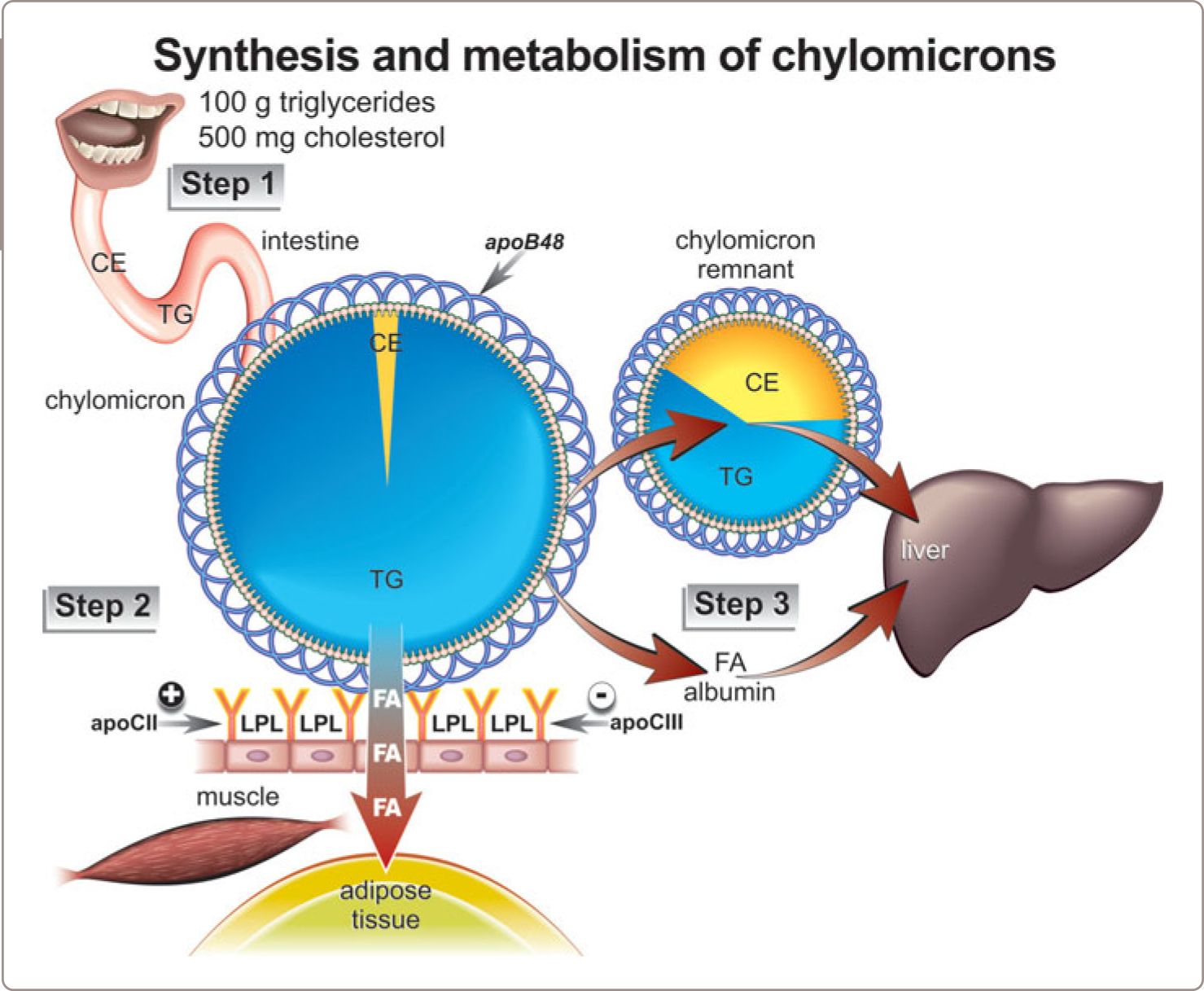
FIGURE 1.2
Synthesis and Secretion of ApoB48 Lipoprotein Particles
An overview of the synthesis and metabolism of chylomicrons is presented in Figure 1.2 . Chylomicron particles are very large particles, 75-1200 nm in diameter, and consist principally of triglycerides (90%) with lesser amounts of phospholipids (approximately 9%), cholesterol (1-3%) and only small amounts of protein (1-2%). Each contains one molecule of apoB48, which stays with the particle during its lifetime. Within the lumen of the small intestine, the dietary triglycerides are hydrolyzed by pancreatic lipases. The fatty acids that are released by pancreatic lipase from the dietary triglyceride as well as the cholesterol that was ingested enter the enterocytes of the small intestine and are reformed into triglycerides. Most of the cholesterol that has been absorbed or synthesized within the enterocytes is esterified and the triglycerides and CE are integrated into the core of a nascent chylomicron particle around the surface of which an apoB48 molecule is wrapped.
Step 1: Chylomicrons are secreted by the enterocytes into the intestinal lymphatics and then enter the systemic circulation via the thoracic duct
Within the lymphatics and the systemic circulation, a series of apolipoproteins are added to their surface. These additional apolipoproteins modify the downstream metabolic fate of the chylomicron particle. During fasting, the smallest chylomicron particles continue to be secreted, but at low rates, and these contribute to the small amount of apoB48 that can be detected in fasting plasma.
Step 2: Interaction of Chylomicrons with Adipose Tissue and Skeletal Muscle
Breakdown or hydrolysis of the triglyceride within a chylomicron particle can only occur after it binds to multiple contiguous molecules of lipoprotein lipase (LPL) which, in turn, are bound to the endothelial surface of the capillary endothelium of adipose tissue, and skeletal and cardiac muscle (Figure 1.2 ). Normally, about 70% of the triglycerides within the core of the chylomicron particle are hydrolyzed by LPL, after the LPL has been activated by apoCII. ApoCII is an apolipoprotein which is present on the surface of the chylomicron particle and which is essential for normal activity of LPL whereas apoCIII, which is also present on the phospholipid monolayer coat of the particle, appears to inhibit LPL activity.
Most of the fatty acids which are released from the chylomicron particle enter the nearby adipocytes or myocytes and are rapidly reformed into triglycerides. However, a significant number bind to albumin and are swept away to enter the systemic circulation. The fraction that goes one way is inversely proportional to the fraction that goes the other. If most of the fatty acids enter the adipocytes – that is, if most are trapped by the adipocyte – few will immediately enter plasma and, accordingly, few will reach the liver. However, if the portion that enters adipocytes is reduced, more will enter plasma and more will reach the liver. This matters for our cardiovascular health because fatty acid flux to the liver is a major determinant of hepatic VLDL secretion which, in turn, is a major determinant of apoB particle number in plasma and therefore of atherogenic risk. Thus, impaired fatty acid trapping by adipose tissue is one of the major physiological causes of elevated apoB or HyperapoB.10
By contrast, little or none of the cholesterol ester core of the chylomicron particle is hydrolyzed or sequestered in adipose tissue and therefore remains with the rest of the chylomicron particle. Because so much of the core triglyceride has been broken down, the chylomicron particle is now much smaller and relatively enriched in cholesterol. Moreover, as the particle gets so much smaller, much of the surface phospholipids, which are now in excess, and many of the surface apolipoproteins – but not, of course, apoB48 itself – are detached from the chylomicron particles. The phospholipids of the external monolayer along with the free cholesterol within it become incorporated into high-density lipoprotein (HDL) particles. There is, therefore, a direct relation between the effectiveness with which chylomicrons are metabolized peripherally and the rate at which HDL is synthesized.
Step 3: Uptake of CR by the Liver
These changes in the structure and volume of the chylomicron particle due to LPL are profound and rapid. The result is a CR, a much smaller particle, relatively enriched in cholesterol, which is released from the endothelial surface usually with a number of molecules of LPL still attached. Within only a few minutes, these CR, which are normal products of the metabolism of the chylomicron particles, are removed from the circulation by attaching to the cell membrane of the hepatocyte and then being internalized (Figure 1.2 ).
The process of binding and internalization of the CR is complex and involves interaction with hepatic lipase, LPL, apoE, and heparin sulfate proteoglycans in addition to receptors, such as the LDL receptor and the LDL-receptor related protein (LRP).11 Because apoB48 does not contain the binding region to the LDL receptor that is within the full-length apoB100, the LDL pathway cannot be directly involved in removal of CR.
1.1.4 The ApoB100 Lipoproteins Particles: VLDL, IDL, LDL
In this segment, we will follow a VLDL particle from its formation in the liver, through its interaction with LPL and conversion to an IDL particle, and finally to an LDL particle (Figure 1.3 ). VLDL particles are triglyceride-rich and have a half-life of 3-4 hours whereas LDL particles are cholesterol-rich and have a half-life of 3-4 days. This marked difference in particle survival time explains why there are almost always 9 times more LDL particles than VLDL particles (Figure 1.4 ). Thus, to repeat, there are two reasons that LDL is a far more important determinant of atherogenic risk than VLDL. First, there are many more LDL particles than VLDL particles and second, LDL particles are smaller and therefore are able to enter the arterial wall more easily than VLDL particles.
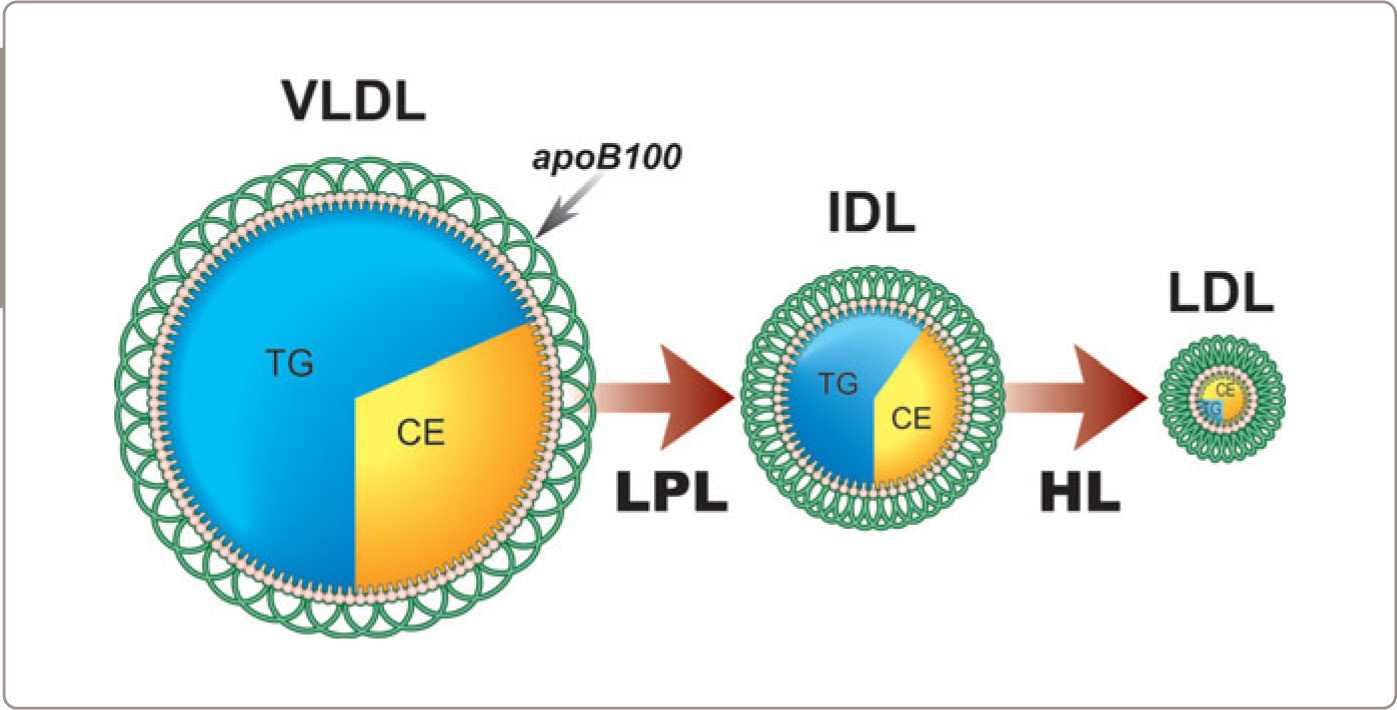
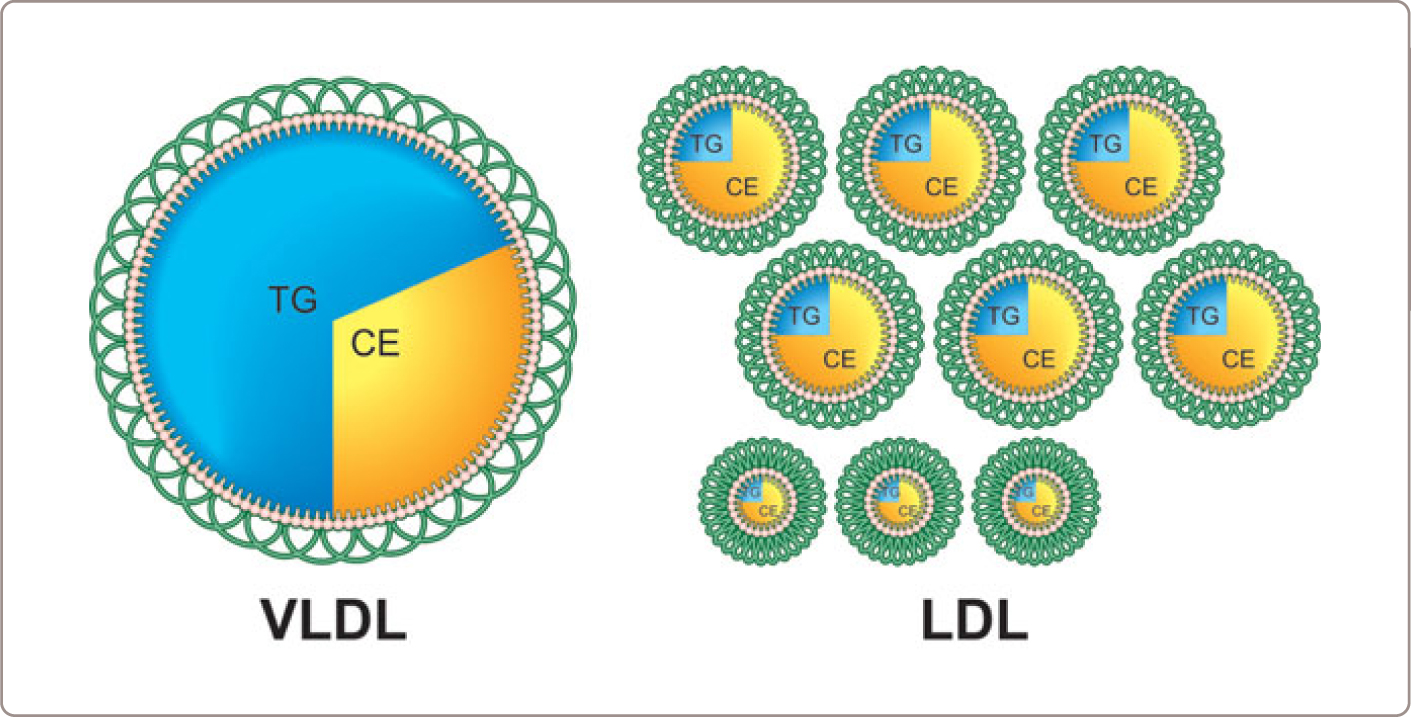
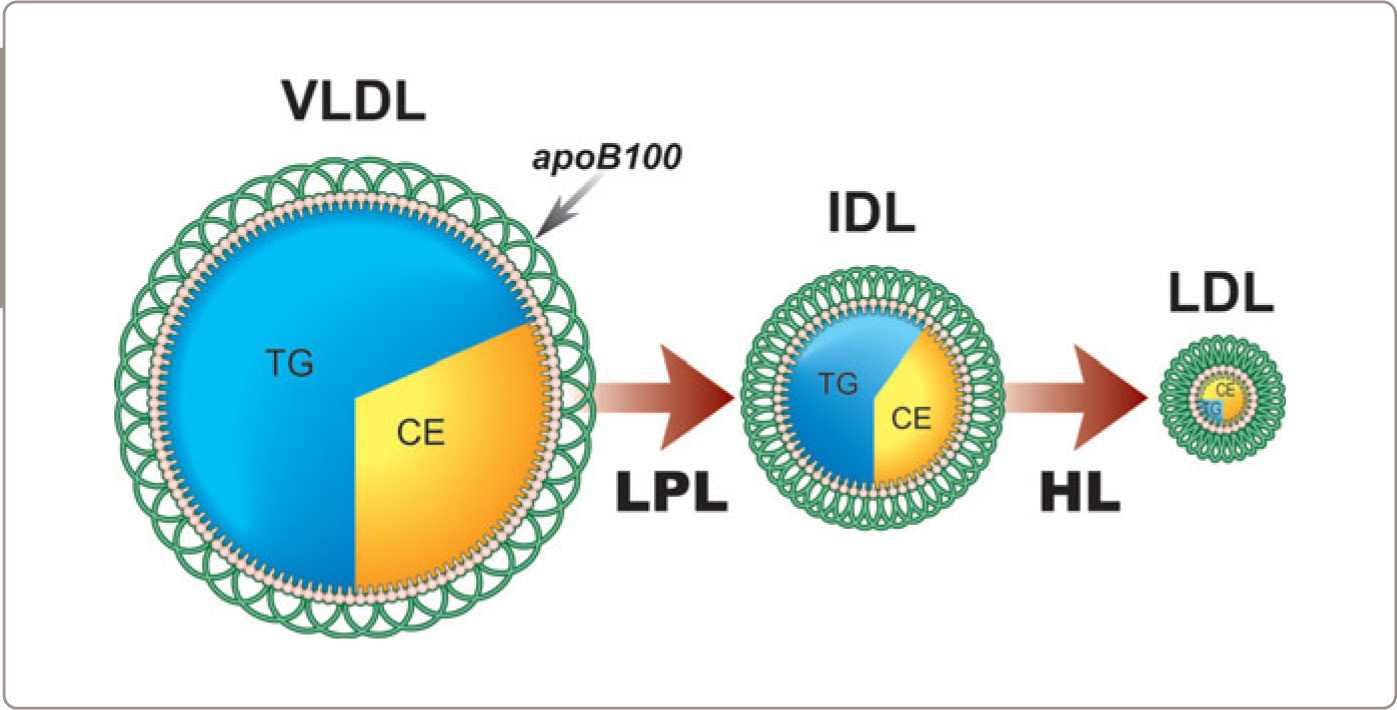
FIGURE 1.3
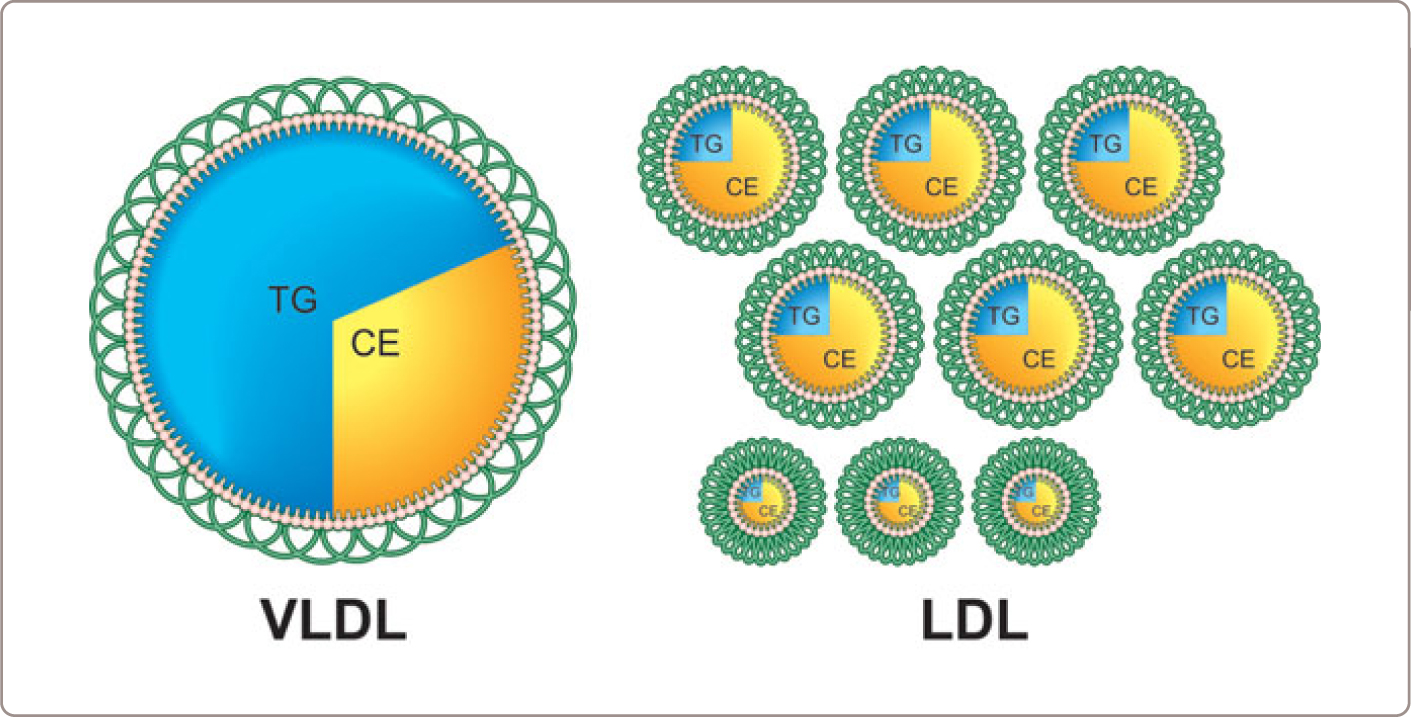
FIGURE 1.4
Step 1: Synthesis and Secretion of VLDL Particles
Much is known about how VLDL particles are constructed within the liver but, at the same time, much remains unknown.1-5,12-15 In particular, we do not know how the major lipids, triglycerides and cholesterol ester are moved from the sites at which they are made and stored within the hepatocyte to the site(s) at which apoB100 lipoprotein particles are assembled. Moreover, no one knows exactly what regulates the rate at which VLDL particles are secreted by the hepatocyte versus what determines just how much core lipid each contains. A general overview of VLDL metabolism is provided in Figure 1.5 .
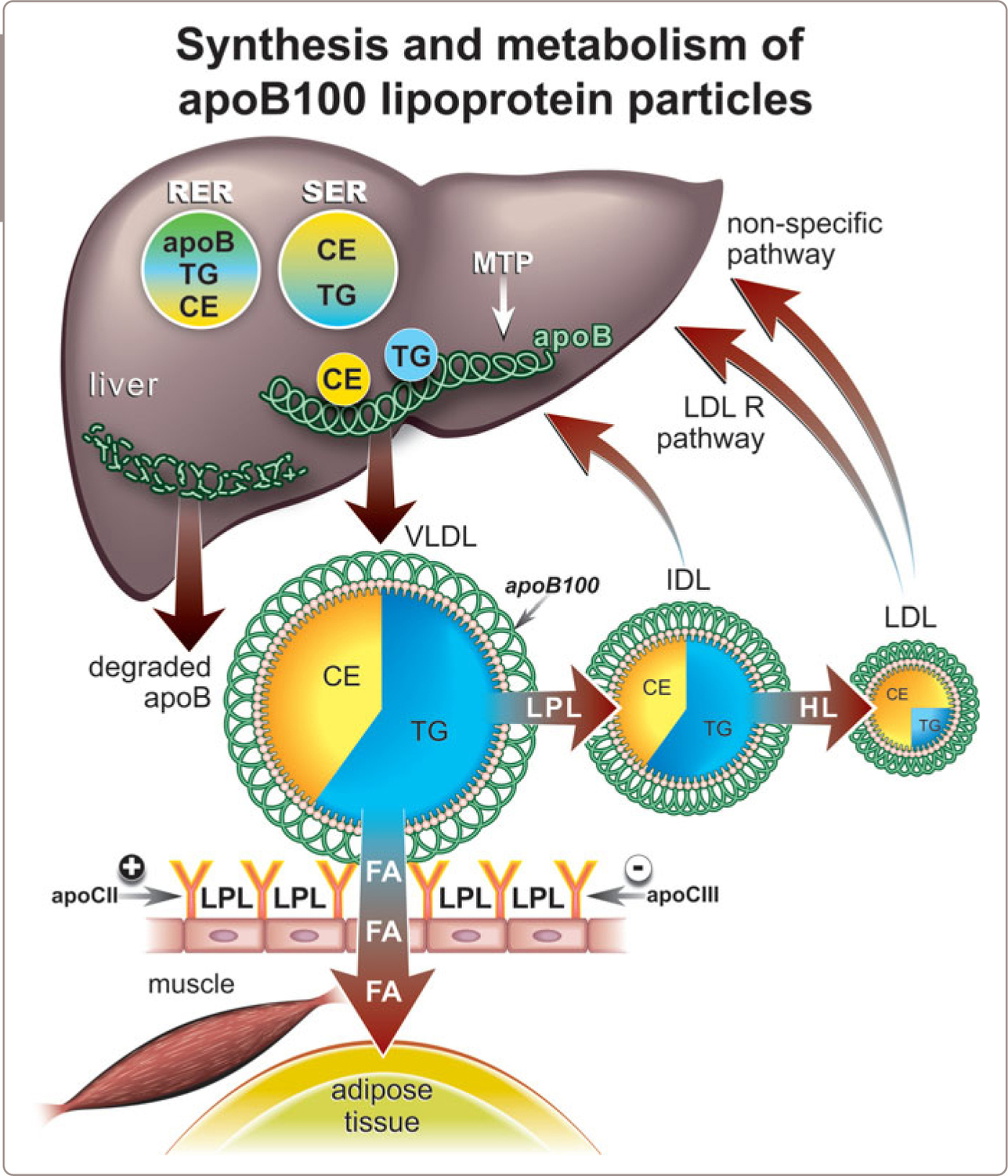
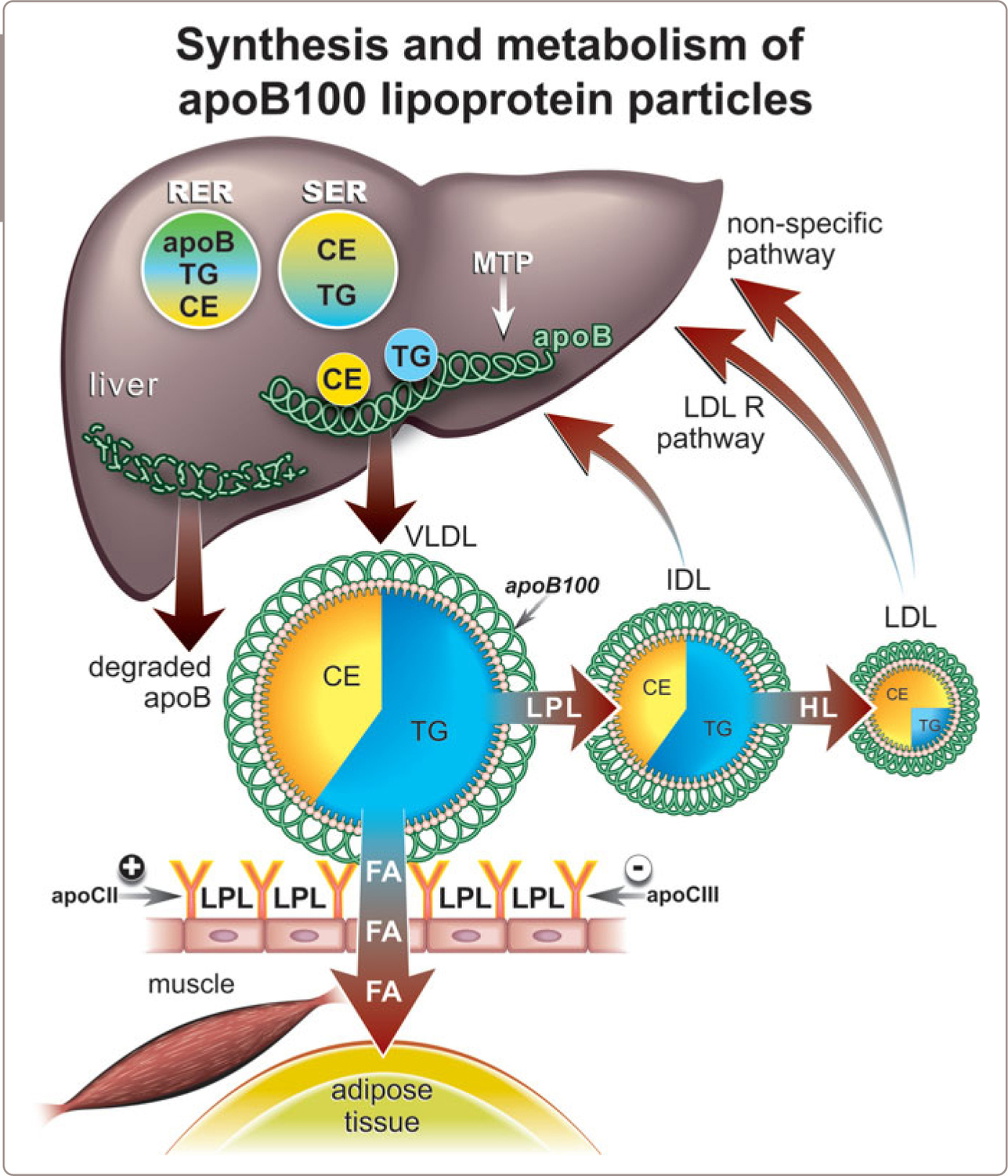
FIGURE 1.5
We do know that apoB100 – the key structural component of VLDL – is synthesized in the rough endoplasmic reticulum (RER) while the principal lipids it will transport from the cell – triglycerides and cholesterol ester – are synthesized in both the rough and smooth endoplasmic reticulum (SER). ApoB100 molecules are synthesized steadily throughout the day. However, only a relatively small number of these newly synthesized apoB molecules successfully associate with lipids and become nascent VLDL particles. Most are hydrolyzed shortly after they are synthesized and it is this step that appears to be the decisive determinant of the rate at which apoB100 lipoprotein particles are secreted by the liver. The early association with lipids is obviously a key step as absence of the protein that transfers cholesterol ester and triglycerides to be associated with nascent VLDL or chylomicron particles – microsomal triglyceride transport protein (MTP) – results in absent secretion of apoB particles from the intestines and the liver.
Other apolipoproteins, such as apoCIII and apoAV, also influence the synthesis and secretion of VLDL particles, perhaps because of effects on triglyceride synthesis and secretion although perhaps also due to an effect on apoB secretion rates. Both apoCIII and apoAV may also interfere with VLDL clearance by diminishing the effectiveness of the interaction of VLDL and LPL.
Because the fraction of apoB molecules that survive to be incorporated in a VLDL particle is variable, the number of VLDL particles secreted into plasma per day can vary substantially. Because VLDL particles are transformed into LDL particles, the number of LDL particles produced per day can vary substantially. Because increased secretion of VLDL particles is the commonest cause of increased LDL particle number in plasma, understanding the determinants of VLDL secretion is of critical importance (Figure 1.5 ).
The key principles are:
1)
The rate at which VLDL particles are secreted can vary substantially and this matters because the rate at which LDL particles are formed is a function of the rate at which VLDL particles are secreted.
2)
The amount of triglyceride per VLDL particle can vary substantially. Therefore, the rate at which triglycerides can be removed from the liver is a function of the mass of triglyceride per VLDL particle that is secreted and the number of VLDL particles secreted per unit time.
3)
Secretion of VLDL particles is an integral mechanism to maintain cholesterol as well as triglyceride homeostasis within the hepatocyte.
Step 2: Interaction of VLDL with LPL
VLDL transports triglyceride to peripheral tissues, principally adipose tissue and skeletal muscle, where LPL hydrolyzes the triglyceride within VLDL just as LPL hydrolyzes the triglyceride within chylomicrons. There are three reasons why chylomicrons are cleared from the circulation in a few minutes whereas VLDL particles are cleared in a few hours. First, there are many more VLDL particles than chylomicron particles; second, VLDL particles bind less readily to LPL than chylomicrons; and third, chylomicron particles undergo only one interaction in peripheral tissue in which most of their triglyceride is removed whereas VLDL must bind to LPL several times (Figure 1.5 ).
On the other hand, because there are so many times more VLDL than chylomicron particles (10 times), even though the affinity of VLDL binding to LPL is so much less than chylomicrons (10 times), VLDL as a class can effectively compete for binding to LPL with chylomicrons. This likely explains why fasting levels of triglyceride, which reflects levels of VLDL, are the major predictor of the rate at which chylomicrons are cleared from plasma.16
Step 3: Fate of VLDL Particles after Release from Adipose Tissue
After their interactions with adipose tissue, the VLDL particles are now smaller, contain less triglyceride, but are relatively enriched in cholesterol ester and contain fewer apolipoproteins on their surface and may either be removed from the circulation by the liver or converted into IDL (Figure 1.5 ). IDL contain roughly equal amounts of triglyceride and cholesterol ester in their core and IDL, in turn, are either removed by the liver or converted into LDL particles by virtue of interaction with hepatic lipase. Unfortunately, little is understood as to what determines the fate of VLDL particles, which means that little is understood as to the regulation of the production of LDL particles. In rats and most other species, relatively few VLDL particles are converted to LDL particles. Thus rats, in contrast to man, in whom a relatively large portion of VLDL particles are converted to LDL particles, are resistant to atherogenesis whereas man is susceptible.
Step 4: LDL
LDL particles are the smallest and most numerous of the apoB100 particles. Most of the triglyceride has been removed from the core of the apoB100, which is now made up principally of cholesterol ester. All, or almost all of the apolipoproteins, have been removed from the surface except, of course, the single molecule of apoB100. The great majority of LDL particles – probably more than 90% – are removed from plasma by the liver either by the LDL-receptor pathway or by non-specific pathways (Figure 1.5 ). The general view is that the number of LDL particles is principally determined by the rate at which they are removed. This is not correct. Increased production of LDL particles is a much more common cause of increased LDL particle number than impaired clearance.17
1.1.5 Lipoprotein(a)
A particle of Lp(a) is a hybrid: an LDL particle which has been covalently linked by a disulfide bridge to a molecule of apolipoprotein (a). Apo(a) is a complex molecule, one component of which is a number of cysteine-rich Kringle IV repeats. Because the number of repeats is variable, there is great heterogeneity in isoform size making assays that accurately quantitate Lp(a) molecular mass challenging to develop. Although the strengths of the epidemiological relations have varied over time, there is credible evidence, particularly from Mendelian randomization studies, that increases in Lp(a) are associated with increases in cardiovascular risk.18,19,20 The evidence that Lp(a) is a causal factor in the pathogenesis of calcific aortic stenosis is, if anything, stronger.21 There has been much speculation as to how Lp(a) promotes atherogenesis – perhaps by inhibiting fibrinolysis, perhaps by inhibiting plasminogen and perhaps by local effects at the lesion site within the arterial wall. However, before Lp(a) should become a routine test, we believe further work is required on the assays to determine if risk relates to the number of Lp(a) particles or to properties of the (a) molecule such as its length. The distribution of Lp(a) levels within the population is highly skewed and dispute remains as to the level as which risk rises and there should be evidence of benefit from randomized trials of clinical benefit from lowering of Lpa(a). Nicotinic acid is the therapeutic agent of choice at the moment and it was disappointing not to see a positive effect on event rate in the AIM-HIGH clinical trial.22 These limitations in knowledge and laboratory technique explain why Lp(a) is not in the apoB algorithm. We look forward to this changing.
1.2 Heterogeneity in the ApoB Lipoprotein Particles
VLDL heterogeneity
VLDL particles are the triglyceride-rich lipoproteins secreted by the liver. They differ in size, composition and metabolic fate. If only plasma triglyceride is measured, physicians do not know which VLDL particles are present. However, by combining triglycerides with cholesterol and apoB and applying the apoB diagnostic algorithm, VLDL particles, chylomicron particles, chylomicron remnant particles and VLDL remnants can be differentiated and identified.
LDL heterogeneity
LDL particles are cholesterol-rich lipoproteins, which are generally products of VLDL metabolism. LDL particles differ in size and cholesterol content. All LDL particles are atherogenic although opinions differ whether small cholesterol-depleted LDL are more atherogenic than large cholesterol-enriched LDL particles. Accordingly, LDL-C does not accurately reflect LDL particle number when either cholesterol-enriched or cholesterol-depleted LDL particles are present. Since there are 9 times more LDL than VLDL particles, LDL particle number determines plasma apoB.
1.2.1 Introduction
There are major differences in the composition of VLDL and LDL particles, differences which influence their metabolic fate and their atherogenic risk and therefore differences which should be taken into account for maximal accuracy in diagnosis and greatest benefit from therapy. In this section we will outline how both VLDL and LDL particles can differ in composition and therefore why the concentration of triglycerides and LDL-C do not necessarily equal the number of VLDL and LDL particles respectively.
1.2.2 VLDL Heterogeneity
Conceptually, VLDL particles can be divided into three subclasses: VLDLI (Sf 100-400), VLDLII (Sf 60-100), and VLDLIII (Sf 20-60).1,23,24 VLDLI are the largest, most triglyceride-rich VLDL particles secreted by the liver whereas VLDLIII are the smallest and contain the most cholesterol ester. VLDLII are intermediate in composition. (Figure 1.6 part A) These are normal variants of VLDL composition and their atherogenic risk – one versus another or indeed any at all – is uncertain. An alternate approach distinguishes two subclasses: VLDL1 (Sf 60-400) and VLDL2 (Sf 20-60): VLDL1 encompassing both VLDLI and VLDLII. No matter how much or how little core lipid they contain, each VLDL particle has one apoB100 molecule (Figure 1.6 part A).
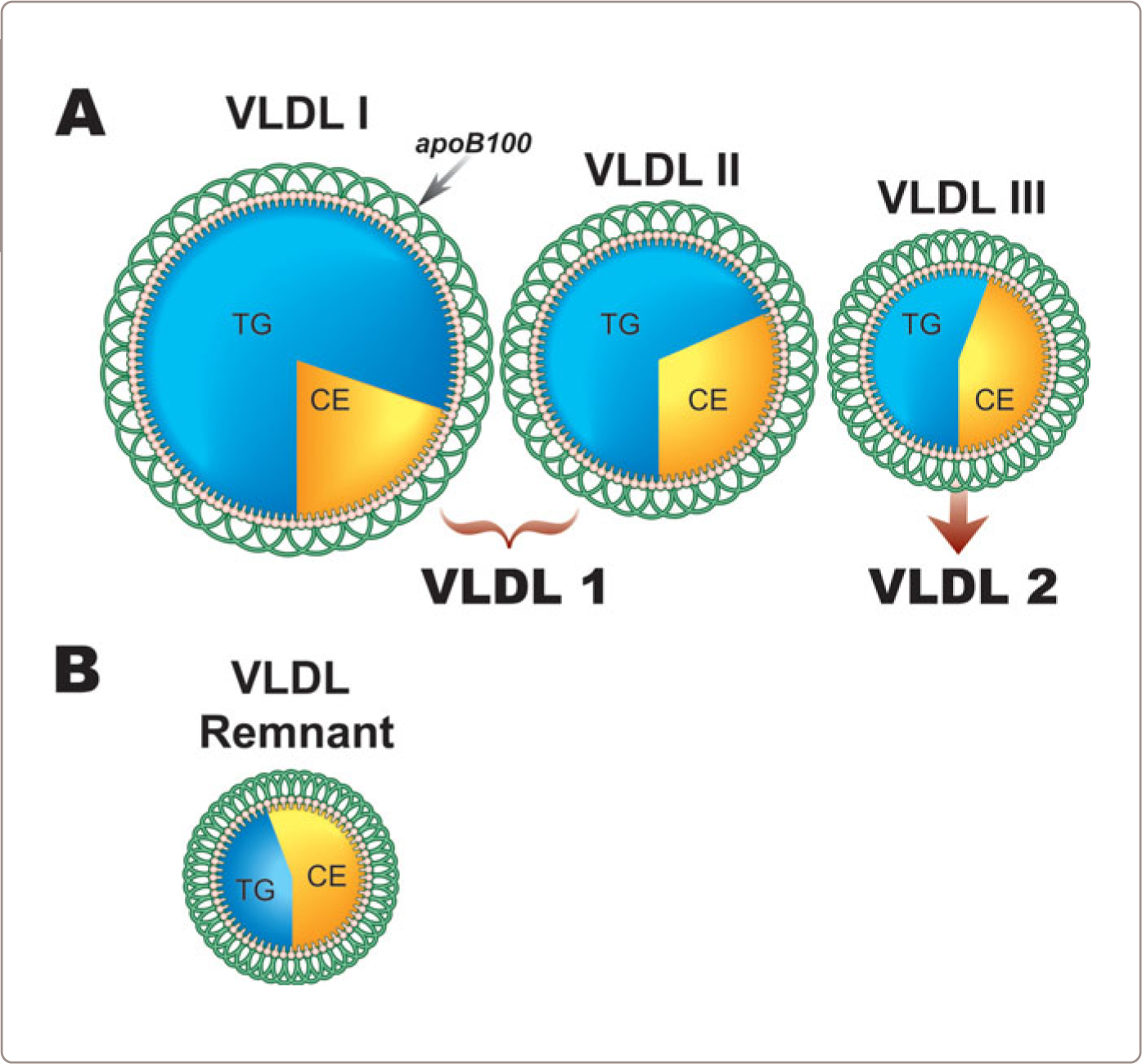
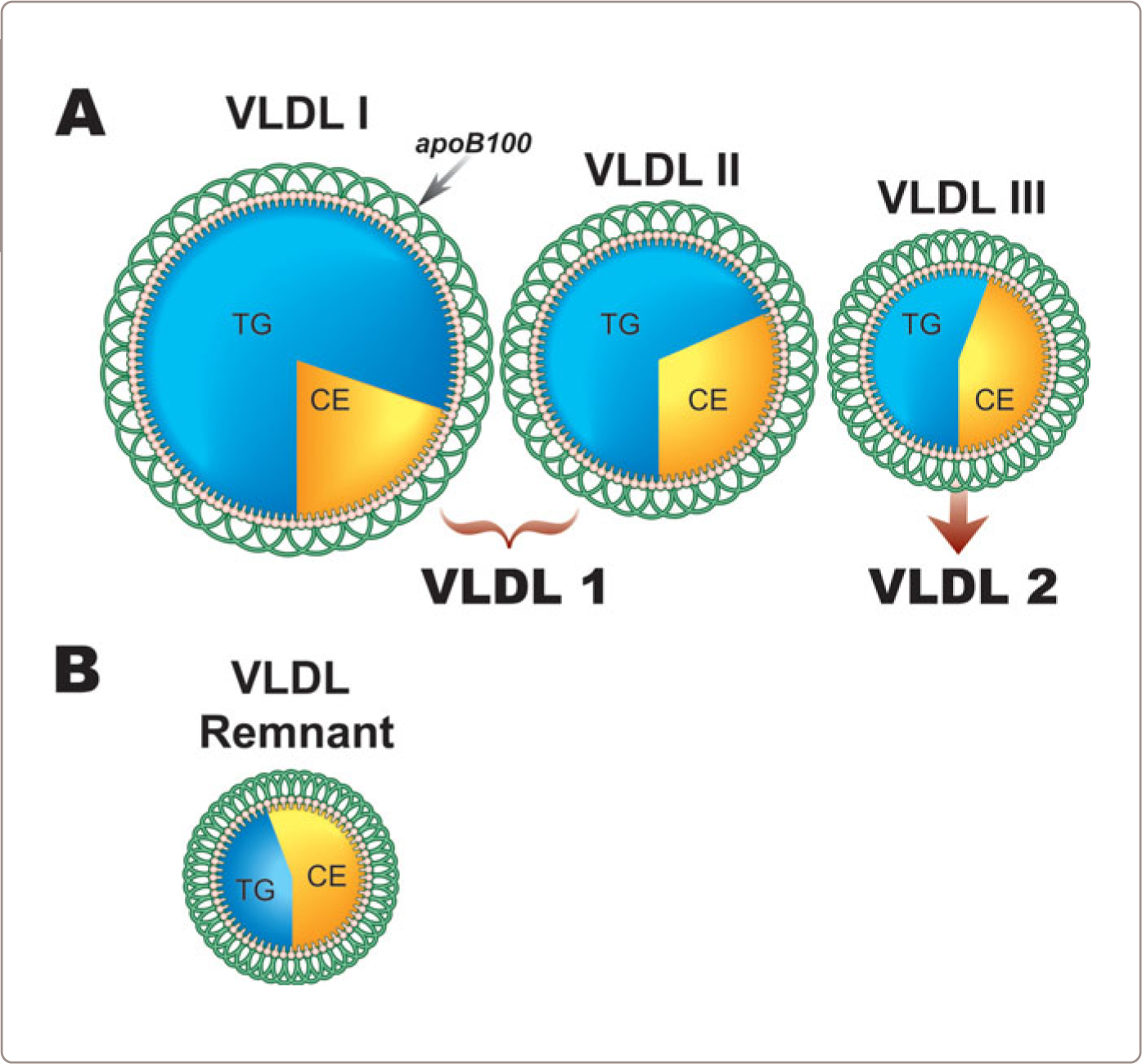
FIGURE 1.6
VLDL particles are normally hydrolyzed by LPL and the progressive removal of triglyceride produces smaller VLDL particles and ultimately IDL and LDL particles (Figure 1.3 and 1.5 ). These are all normal particles, present in different numbers in different individuals. The normal metabolic transformation of VLDL particles occurs within a few hours (half-life of 4-6 hours). By contrast, VLDL remnants are abnormal VLDL particles, which are enriched in cholesterol ester compared with other VLDL particles (Figure 1.6 part B). The excess cholesterol is due to the continued exchange of core lipids – cholesterol ester and triglyceride – between VLDL and HDL/LDL particles under circumstances when clearance of VLDL and partially metabolized chylomicron particles is markedly delayed. It is the marked delay that produces the extra time for the marked increase in cholesterol ester in the VLDL and CR to occur.
Remnant lipoprotein disorder, or type III dyslipoproteinemia, is the paradigmatic clinical example of this metabolic abnormality.25,26 Atherogenic risk in these patients is markedly increased and that is why there is such great concern about remnant lipoprotein particles. However, remnant particle number in these patients is increased at least 20 to 40 fold above that in other circumstances.27,28 So, in remnant lipoprotein disorder, remnants equal or outnumber normal VLDL particles. How much additional clinical risk there is with remnant particle numbers in the more usual range when remnants are one-fifth or less of the total VLDL particle number remains unknown.
VLDL Heterogeneity: Regulation of VLDL Composition and Particle Number
Three different processes interact to account for heterogeneity in the composition of VLDL particles. The first is differences in the composition of the VLDL particles that are secreted. Thus, the mass of triglyceride incorporated into each nascent apoB particle may differ substantially: the more triglyceride that incorporated into a nascent particle, the larger the VLDL particle that will be secreted.1,23,24,29 The actual mass of triglyceride that is incorporated will be determined by the mass of triglyceride available to be incorporated in the smooth endoplasmic reticulum or the Golgi versus the number of nascent apoB molecules available to incorporate these masses of triglyceride (Figure 1.5 ).
If large amounts of triglyceride are incorporated into small numbers of apoB particles, the liver will secrete small numbers of large triglyceride-rich VLDL I particles (Figure 1.7 , number 1). However, if large amounts of triglyceride are incorporated into large numbers of apoB particles, the liver will secrete large numbers of VLDL particles with average amounts of triglyceride (VLDL II) (Figure 1.7 , number 2). Increased secretion of relatively triglyceride-poor, cholesterol-rich VLDL particles (VLDL III) (Figure 1.7 , number 3) has also been documented in patients with NormoTG HyperapoB, such as patients with familial hypercholesterolemia.17
