Cell line
Reported IC50 (μM)
Concentration (in μg/mL)
Citations
Cervical HeLa
80
24.18
Priyadarsini et al. (2011)
Breast MCF-7
92.4
27.93
Chou et al. (2010)
Prostate PC-3
100
30.22
Senthilkumar et al. (2011)
Pancreatic MIA PaCa-2
178
53.80
Harris et al. (2012)
Glioblastoma U87
17.1
5.17
Michaud-levesque et al. (2012)
Glioblastoma T98G
24.3
7.34
Michaud-levesque et al. (2012)
Colon LoVo
40.2
12.15
Zhang et al. (2012)
Breast MCF-7
30.8
9.31
Zhang et al. (2012)
Liver HepG2
40.9
12.36
He and Liu (2008)
Breast MCF-7
137.5
41.56
He and Liu (2008)
Animal studies have further confirmed the chemopreventive and chemotherapeutic properties of quercetin. Administration of quercetin to rats provided protection from sodium fluoride-induced oxidative stress in N-Nitrosodiethylamine (NDEA)-induced hepatocarcinoma (Nabavi et al. 2012; Seufi et al. 2009). Chemopreventive effect was observed with a significant reduction in the incidence and tumor burden of 7,12-dimethylbenz[a] anthracene (DMBA)-induced hamster buccal pouch carcinomas when simultaneously treated by intragastric administration of quercetin, while hamsters treated after DMBA was discontinued benefitted from a significant delay in tumor growth (Priyadarsini et al. 2011). Quercetin provided a reduction of tumor burden in lung and mammary carcinomas as well as decreased incidence of aberrant crypt foci and pre-neoplastic lesions of the colon (Gibellini et al. 2011). Taken together, these results demonstrate the potential of quercetin in prevention and treatment of cancer, but also serve to illustrate the need for further mechanistic in vitro and in vivo research of this apple flavonoid.
While the data on quercetin are compelling, the role of other components in quercetin containing foods has become a subject of debate. In apples, there is preliminary evidence that procyanidins may exert stronger antioxidant and antiproliferative activity than quercetin (Gerhauser 2008). An apple juice procyanidin fraction inhibited proliferation of colon cancer cells more effectively than another fraction with catechin, epicatechin, and quercetin, even at double the dose (Gosse et al. 2005). Further inquiry in a rat model concurred by exhibiting a 50 % reduction in the number of pre-neoplastic colonic lesions in rats fed with 0.01 % procyanidin fraction added to drinking water when compared to control rats (Gosse et al. 2005). Apple procyanidins exhibited 2.5× higher antiproliferative activity than apple polyphenols in mouse melanoma cells and 5× higher in mouse mammary cells, results that were also supported by follow-up in vivo research (Miura et al. 2008). A comparison of the antiproliferative activity of apple waste fractions of extractable polyphenols (EPP) and non-extractable polyphenols (NEPP) in cervical, hepatic, and colon cancer cell lines suggested that the NEPP fraction, containing mostly anthocyanidins, exerted higher inhibitory activity in all cell lines (Tow et al. 2011). In addition to cyanidins, several triterpenoids isolated from apple peels have exhibited significant antiproliferative activity in liver, breast, and colon cancer cells at doses ranging from 4.7 μM to 136 μM (He and Liu 2007). Although continued research on these individual apple phytochemicals is vital to discover molecular drug targets and mechanisms, a comprehensive understanding of the interactions between these varied and plentiful constituents of apples warrants serious consideration.
2.1.2 A Whole Apple a Day Fights the Disease Away
While numerous studies have focused on the bioactivity of single phytochemicals, there is considerable data on whole apples, apple juices, and apple extracts, which supply ample evidence of synergistic effects between apple components. Apple extracts have shown in vitro efficacy in a broad array of cancer cell lines, and significant inhibition of mammary carcinogenesis, skin papillomas, solid tumors, and metastasis of lung and lymph nodes has been reported in several in vivo investigations.
Whole apple extracts from nine cultivars native to Portugal were evaluated in colon and gastric cancer cells, reporting significant antiproliferative activity from all cultivars with effective doses (ED50s) from 9.0 to 49.0 mg/mL (Serra et al. 2010). Intriguingly, the cultivar with the most antiproliferative activity did not have the highest quercetin content. In fact, an extract containing nearly four times the quercetin was a less potent inhibitor of cell growth, suggesting that while quercetin does have anticancer activity, it is not working alone and the observed bioactivity can be attributed to other compounds or synergy between the complex blend of phytochemicals in apples. Inhibition of breast cancer cell proliferation was accomplished by apple extracts in both MCF-7 estrogen receptor (ER)-positive cells and with twice the efficacy in ER-negative MDA-MB-231 cells (Sun and Liu 2008; Yoon and Liu 2007). Separately, apple peel extracts significantly suppressed cell growth in breast cancer cells overexpressing ErbB2 as well as those with normal levels of ErbB2 (Reagan-Shaw et al. 2010). Additionally, both androgen-dependent and androgen-independent prostate cancer cell proliferation was inhibited by these apple peel extracts, which could prove advantageous in some chemo-resistant cancers (Reagan-Shaw et al. 2010).
Oral administration of whole apple extracts equivalent to human consumption of 1, 3, and 6 apples per day demonstrated dose-dependent reduction of mammary tumor incidence and tumor burden in rats (Liu et al. 2005, 2009). A comparison of clear and cloudy apple juice ingestion in a rat colon cancer model revealed considerably increased preventive potential of cloudy apple juice despite comparable polyphenolic content of the two juices with the exception of 30 % more procyanidins and a fourfold increase of pectin in the cloudy juice (Barth et al. 2005). While clear apple juice provided modest reductions in genetic damage and proliferation, rats given cloudy apple juice were observed to develop 50 % fewer large aberrant crypt foci (ACF) as well as a significant decrease in the total number of these pre-neoplastic lesions (Barth et al. 2005). Subsequent research compared cloudy apple juice to polyphenolic and cloudy fractions as well as a combination of the two fractions in an effort to ascertain the fraction of the juice with the most potent anticancer properties (Barth et al. 2007). While all preparations reduced hyperproliferation of epithelial cells in the colon as evidenced by the percentage of BrdU-positive cells, cloudy apple juice outperformed the juice fractions and even the combination fraction in reducing DNA damage and provided a 35 % decrease in the development of large ACF. One of the prevailing theories for the effectiveness of natural products holds that the polyphenols are the active “ingredient.” These studies illustrate that it is the sum of the components, not just the polyphenols that impart the anticancer benefits seen with apple products (Barth et al. 2007).
There are only a few reports of direct comparisons between whole extracts and individual apple compounds including an in vitro investigation with colon cancer cells comparing an apple flavonoid extract, individual phytochemicals, and a synthetic extract mimicking the original (Veeriah et al. 2006). The natural apple extract was five times more effective than quercetin alone with an EC50 of 31.0 μM (phloridzin-equivalents, Ph.E) compared to 148.4 μM for quercetin. The synthetic extract, with an EC50 of 59.9 μM Ph.E, was more effective than quercetin but still not as effective as the natural extract (Veeriah et al. 2006), suggesting that there are other components in the natural extract that were not quantified and included in the synthetic extract, lending further support to the idea of superior benefits from whole foods.
An interesting comparison of a whole apple extract, quercetin 3-β-d-glucoside (Q3G), and a combination of the two showed that while both Q3G and apple extracts efficiently inhibited proliferation in MCF-7 breast cancer cells, the combination of the two was considerably more effective, resulting in an EC50 which was twofold lower than apple extracts and fourfold lower than Q3G (Yang and Liu 2009). These results suggest that improving on existing combinations from nature may yield more effective treatments than reducing these foods down to their most active ingredient.
Explorations of the anticancer properties of quercetin and whole apple extract have yielded convincing evidence to demonstrate synergy in apples. As shown in Table 1 , in most studies, the doses of quercetin required to inhibit cancer cell proliferation were between 17.1 and 178 μM (5.17 and 53.8 μg/mL), but as a portion of the whole apple extract, quercetin comprises just 0.0000193–0.0072 % meaning its contribution to the observed anticancer effects of whole apple extract amounts to only 0.001737–11.1816 μg/mL, a 2–5-fold difference (Table 2). Clearly, quercetin is only one piece of a complex puzzle (Fig. 1) when it comes to chemoprevention with apples. It is reasonable to suggest that no single component in apples can produce the benefits summarized in this review.
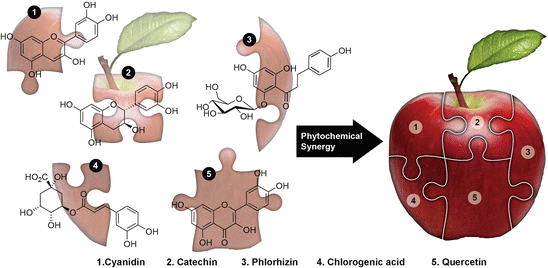
Table 2
Antiproliferative activity of whole apple extracts with Quercetin contribution to the effect of the whole
Material tested | Cell line | IC50 (in mg/mL) | Contribution range of quercetin to IC50 (μg/mL) of Whole apple extract | Citations | |
---|---|---|---|---|---|
Low | High | ||||
Bravo de Esmolfe | Colon HT29 | 37 | 0.007141 | 2.664 | Serra (2010) |
Malapio Fino | 24.8 | 0.0047864 | 1.7856 | ||
Malapio de Serra | 37 | 0.007141 | 2.664 | ||
Pero Pipo | 49 | 0.009457 | 3.528 | ||
Golden | 42.2 | 0.0081446 | 3.0384 | ||
Starking | 24.1 | 0.0046513 | 1.7352 | ||
Fuji | 25.8 | 0.0049794 | 1.8576 | ||
Gala Galaxy | 48 | 0.009264 | 3.456 | ||
Reineta Parda | 21.1 | 0.0040723 | 1.5192 | ||
Bravo de Esmolfe | Gastric MKN45 | 15 | 0.002895 | 1.08 | Serra (2010) |
Malapio Fino | 11.9 | 0.0022967 | 0.8568 | ||
Malapio de Serra | 19.8 | 0.0038214 | 1.4256 | ||
Pero Pipo | 13.2 | 0.0025476 | 0.9504 | ||
Golden | 26.3 | 0.0050759 | 1.8936 | ||
Starking | 15 | 0.002895 | 1.08 | ||
Fuji | 17.2 | 0.0033196 | 1.2384 | ||
Gala Galaxy | 23.8 | 0.0045934 | 1.7136 | ||
Reineta Parda | 9 | 0.001737 | 0.648 | ||
Red Delicious (whole extract) | Breast MCF-7 | 65.1 | 0.0125643 | 4.6872 | Yoon and Liu (2007) |
Red Delicious (whole extract) | 70.7 | 0.0136451 | 5.0904 | Yang and Liu (2009) | |
Red Delicious (phenolic extract) | 69.9 | 0.0134907 | 5.0328 | Sun and Liu (2008) | |
Red Delicious (phenolic extract) | Breast MDA-MB-231 | 35.2 | 0.0067936 | 2.5344 | Sun and Liu (2008) |
Apple juice extract | Colon HT-29 | 31 μM | 0.005983 | 2.232 | Veeriah et al. (2006) |
Synthetic apple extract | 59.9 μM | 0.0115607 | 4.3128 | ||
Rome Beauty (flesh + peel) | Liver HepG2 | 26.5 | 0.0051145 | 1.908 | Wolfe et al. (2003) |
Rome Beauty (peel) | 12.4 | 0.0023932 | 0.8928 | ||
Idared (flesh + peel) | 125.1 | 0.0241443 | 9.0072 | ||
Idared (peel) | 13.6 | 0.0026248 | 0.9792 | ||
Cortland (flesh) | 103.9 | 0.0200527 | 7.4808 | ||
Cortland (flesh + peel) | 74.1 | 0.0143013 | 5.3352 | ||
Cortland (peel) | 15.7 | 0.0030301 | 1.1304 | ||
Golden Delicious (flesh) | 155.3 | 0.0299729 | 11.1816 | ||
Golden Delicious (flesh + peel) | 107.7 | 0.0207861 | 7.7544 | ||
Golden Delicious (peel) | 20.2 | 0.0038986 | 1.4544 | ||
Quercetin content of apples is in the range 0.0000193–0.0072 % |
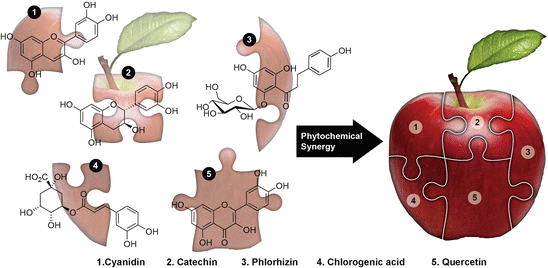
Fig. 1
The in’cider truth about apples: The apple phytochemicals including cyanidins, catechins, phlorhizin, chlorogenic acid, quercetin, etc., are nothing but the mystifying puzzle pieces of whole apples, which were brought together into a phytocomplex by Mother Nature to confer synergized health benefits
Extensive in vitro and in vivo studies have demonstrated the potential health benefits of apples with the majority of literature supporting both the health benefits from apples and the concept of synergy in apples, implying that this fruit should be consumed frequently and in forms utilizing the whole apple. Future research of mechanisms, molecular targets, and the interactions between apple components may provide direction on how best to capitalize on apple’s benefits.
2.2 Pomegranate: Pomtastic Truths About a Time-Honored Healing Fruit
Pomegranates have been consumed as food, used as medicine, and featured prominently in a number of ancient religious traditions. Despite its long history of human applications, scientific research of this fruit is still relatively new, having started in earnest approximately 20 years ago. Investigations of pomegranate fruit extracts (PFE), fractions, or purified compounds indicate that pomegranate has antioxidant, antiproliferative, anti-invasion, anti-angiogenic, and pro-apoptotic activity in a wide range of cancer cell lines (Adhami et al. 2009; Lansky and Newman 2007; Syed et al. 2007; Faria and Calhau 2011). In addition to data from in vitro evaluations, animal studies have confirmed the efficacy of pomegranate, even leading to human clinical research (Adhami et al. 2009). The phytocomplexity in pomegranates is driven by at least 124 different phytochemicals (Fig. 2), comprised primarily of ellagitannins specific to pomegranate, punicalagins, which can be hydrolyzed to ellagic acid, in addition to anthocyanins and flavonols (Faria and Calhau 2011; Heber 2011; Banerjee et al. 2012). The quantity of any of these polyphenols in pomegranate products is dependent on the preparation methods, with considerably greater amounts of polyphenols in extracts made from whole fruit juices as opposed to extracts from the juice of the arils only (Faria and Calhau 2011; Lansky et al. 2005).
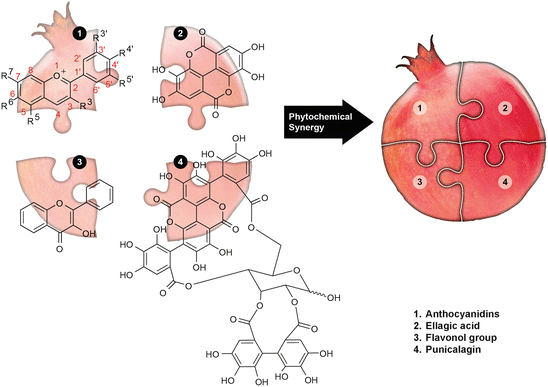
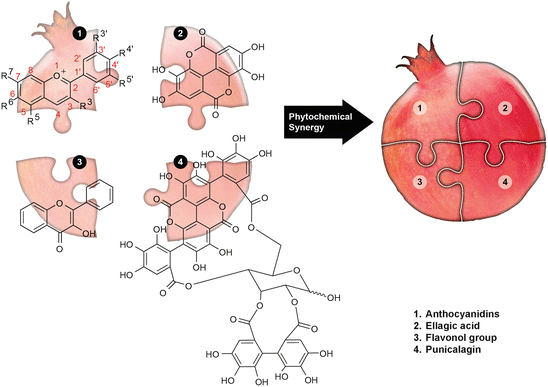
Fig. 2
Poms pack the punch in their paunch: Like there are no extra pieces in the universe, anthocyanidins, ellagic acid, flavonols, punicalgins, etc., make up the complex pomegranate jigsaw puzzle to exercise synergy, thus providing optimal health benefits
2.2.1 Ellagic Acid: The Hydrolyzed Antioxidant Tannin
Of these compounds, ellagic acid (EA) quickly became the phytochemical of research focus, demonstrating potent antioxidant and anticarcinogenic capabilities (Jurenka 2008). In vitro studies suggest an antiproliferative role for EA in a wide range of cancer cell lines. Neuroblastoma cell growth was inhibited by EA in concentrations between 10 and 30 μM (Fjaeraa and Nanberg 2009). As shown in Table 3, treatment with EA was also observed to significantly reduce cell growth in breast, prostate, colon, skin, ovarian, oral cavity, and bone cancer cells with IC50 doses between 21.5 and 462 μM (6.5–139.6 μg/mL) (Han et al. 2006; Kasimsetty et al. 2010; Malik et al. 2011; Seeram et al. 2007).
Table 3
Antiproliferative activity of ellagic acid
Cell line | Reported IC50 (μM) | Concentration (in μg/mL) | Citations |
---|---|---|---|
Prostate PC-3 | 80 | 24.18 | Malik et al. (2011) |
Human osteogenic sarcoma | 21.5 | 6.50 | Han et al. (2006) |
Prostate LNCaP | 62.4 | 18.86 | Seeram et al. (2007) |
Prostate LNCaP-AR | 78.7 | 23.87 | Seeram et al. (2007) |
Prostate DU145 | 74.3 | 22.45 | Seeram et al. (2007) |
Prostate 22RV1 | 108.7 | 32.85 | Seeram et al. (2007) |
Breast BT-549 | 198 | 59.84 | Kasimsetty et al. (2010) |
Colon HT-29 | 462 | 139.60 | Kasimsetty et al. (2010) |
Oral KB | 300 | 90.66 | Kasimsetty et al. (2010) |
Melanoma SK-MEL | 132 | 39.89 | Kasimsetty et al. (2010) |
Ovarian SK-OV-3 | 222 | 67.09 | Kasimsetty et al. (2010) |
In vivo research has demonstrated that a diet supplemented with EA in an estrogen-induced mammary tumor rat model significantly reduced the incidence of tumors by 19 %, tumor volume by 65 %, and tumor multiplicity by 41 % when compared to rats given a control diet (Aiyer and Gupta 2010). A mouse skin cancer model resulted in significant inhibition of DMBA-induced epidermal hyperplasia with topical application of EA while the incidence of aberrant crypt foci was significantly reduced in a DMH-induced colon cancer rat model (Kowalczyk et al. 2009; Umesalma and Sudhandiran 2010). Hamster buccal pouch carcinoma also induced by DMBA was nonexistent in hamsters with EA-supplemented diet, although hyperplasia was evident upon histopathological evaluation (Vidya Priyadarsini et al. 2012).
Cellular and animal investigations have thus presented strong evidence that EA is a promising phytochemical for the prevention and treatment of cancer. However, the doses required to provide these beneficial effects are considerably higher than the physiologically attainable blood plasma level of 0.018–0.033 μM (Mertens-Talcott et al. 2006; Seeram et al. 2004, 2006). Since inquiries into the anticancer properties of pomegranate phytochemicals other than EA have also shown potential for chemoprevention and treatment, the spotlight has turned to focus on properties of the whole pomegranate.
2.2.2 Whole Pomegranate Extract: The Highly Effective Antioxidant Concoction
Confirmation of the anticancer benefits of whole pomegranate has been provided by a number of animal studies of prostate, skin, and lung cancer. Inhibition of proliferation by pomegranate extracts has been explored in several in vitro studies of prostate, lung, oral, and colon cell lines with 30–100 % inhibition of proliferation observed with doses from 10 to 150 μg/mL (Khan et al. 2007b; Malik et al. 2005; Seeram et al. 2005). Intriguingly, one of these studies determined that greater antiproliferative activity was provided by whole pomegranate juice when compared to a total pomegranate tannin extract, punicalagin, and ellagic acid at normalized concentrations of punicalagin (Seeram et al. 2005). Pomegranate juice metabolites, including ellagic acid and urolithin A, were found to be synergistically interacting among themselves, thus emphasizing the idea of phytocomplexity, to inhibit growth of PC-3 and DU145 cells, even causing death by affecting the cell cycle kinetics and induction of apoptosis (Vicinanza et al. 2013). Pomegranate extract was also found to inhibit proliferation of breast cancer cells while inducing apoptosis (Shirode et al. 2014). The authors attribute the antiproliferative effects to reduced DNA repair gene expression and induction of double-stranded DNA damage (Shirode et al. 2014). Pancreatic cancer cell growth was decreased with anIC50value of 50 μg/mL while higher doses of 100 and 125 μg/mL were required for two oral cancer lines (Nair et al. 2011; Weisburg et al. 2010). In contrast, prostate cancer cells were inhibited by a whole pomegranate fruit extract (PFE) with an observed IC50 value of only 5.7 μg/mL (Sartippour et al. 2008). In addition to antiproliferative activity, a protective role for PFE was suggested when UVA- and UVB-induced damage in normal human epithelial keratinocytes (NHEK) was inhibited by treatment with PFE (Afaq et al. 2005a; Syed et al. 2006). Follow-up studies in mice further illustrated the photochemopreventive potential of pomegranate with oral administration of PFE demonstrating inhibition of UVB-induced damage and early carcinogenesis (Afaq et al. 2010). A summary of these studies is found in Table 4 .
Table 4
Antiproliferative activity of whole pomegranate extract
Cell line | Reported IC50 (μg/mL) of whole pomegranate extract | Contribution of ellagic acid to IC50 (μg/mL) of whole pomegranate extract | Citations |
---|---|---|---|
Pancreas PANC-1 | 50 | 1.35 | Nair et al. (2011) |
Oral HSC-2 | 100 | 2.70 | Weisburg et al. (2010) |
Tongue CAL-27 | 125 | 3.38 | Weisburg et al. (2010) |
Prostate LNCaP | 5.7 | 0.15 | Sartippour et al. (2008) |
Endothelial HUVEC | 6.7 | 0.18 | Sartippour et al. (2008) |
Prostate cancer was significantly inhibited in mouse xenograft models upon oral ingestion of PFE resulting in 35–60 % reduction in tumor volumes, delays in tumor incidence, and significantly increased survival times (Seeram et al. 2007; Malik et al. 2005; Adhami et al. 2012; Sartippour et al. 2008). Efficacy of PFE against lung cancer was explored in a xenograft model and a Benzo(a)pyrene [B(a)P] and N-nitroso-tris-chloroethylurea (NTCU)-induced model, both of which involved oral administration of PFE to mice which produced delayed progression of tumors, significantly increased survival time, and over 50 % reduction in tumor volume (Khan et al. 2007a, b). A 33 % tumor growth inhibition of tobacco smoke-induced lung adenomas was observed upon daily oral administration of pomegranate fruit extract (Naghma Khan et al. 2013). Skin tumors induced by 12-O-tetradecanoylphorbol-13-acetate (TPA) developed later, were fewer in number, and weighed less in mice treated with topical applications of PFE as opposed to control mice (Afaq et al. 2005b).
An initial human trial treating 46 men with rising prostate-specific antigen (PSA) values after primary treatment indicated a significant increase in the average PSA doubling time (PSADT) with daily oral ingestion of 8 oz of pomegranate juice. Untreated PSADT averaged 15 months while PSADT for treated patients averaged 54 months. As this was an open-label, single-arm, small clinical trial, future investigations are planned that will include more patients and placebo controls (Pantuck et al. 2006). More recently,a similar result of lengthened PSADT was observed in a randomized, double-blind phase II clinical trial, where PSA levels even declined in about 13 % of patients in the test group treated with pomegranate extract (POMx) (Paller et al. 2013). However, in direct contrast another placebo-controlled clinical trial in Switzerland re-reported that daily pomegranate intake does not influence the PSA levels when compared to the placebo group (Stenner-Liewen et al. 2013). It is important to note that these studies involved different populations, with the Swiss study treating advanced prostate cancer patients. Additionally, the studies used different preparations of pomegranate, a critical factor in this kind of research. The initial trial by Pantuck et al. used a juice made from crushing the whole fruit while the other studies used pomegranate extract capsules or a juice product that included 27 % pomegranate extract. As the quantity and composition of phytochemicals can vary widely based on preparation methods, the use of different study populations and products could be responsible for these contradictory results.
Several studies providing comparisons between whole products and fractions or constituents of pomegranate have suggested an additive or synergistic effect with treatment by whole pomegranate products. In pancreatic cancer cells, PFE treatment was observed to induce cell cycle arrest and inhibited cell proliferation at an IC50 of 50 μg/mL, while a dose of 25–30 μM (7.55–9.07 μg/mL) produced the same results with ellagic acid. Given that ellagic acid comprises only 2.7 % of PFE, its contribution to the effects provided by whole pomegranate extract equates to only 1.35 μg/mL, indicating a minimum fivefold difference in the concentration of EA required when administered as an isolated compound (Nair et al. 2011). Additionally, a comparison between pomegranate extract and paclitaxel determined that PFE inhibited cell proliferation more quickly and more effectively than this standard chemotherapeutic drug (Nair et al. 2011).
Pomegranate juice (PJ) exhibited greater inhibition of proliferation than constituent compounds punicalagin, ellagic acid, and a standardized total pomegranate extract in a variety of cancer cell lines (Seeram et al. 2005). While all treatments demonstrated dose-dependent reductions in cell growth, the inhibition provided by PJ at very low doses was not achievable by other compounds without considerable increases in dosage. Similarly, a comparison of pomegranate juice (PJ), POMx (whole extract), punicalagin, and ellagic acid revealed superior antiproliferative activity of PJ in all three prostate cancer cell lines evaluated (Hong et al. 2008). It is important to note that both PJ and POMx were processed from the whole pomegranate and should therefore provide similar levels of inhibition, but the effects of POMx did not approach those of PJ until higher doses were utilized.
An interesting study evaluating antiproliferative activity of individual pomegranate phytochemicals compared to the whole extract found that while both the extract and the phytochemicals inhibited proliferation and reduced viability of mouse mammary cancer stem cells in a time- and dose-related manner, the doses of phytochemicals were higher than those found in the whole extract. EA inhibited cell growth with an IC50 of ~7.5 μM (2.27 μg/mL), but its contribution to the IC50 of PE was 1.35 μg/mL, a difference of 41 %, suggesting that the effect of EA is enhanced when administered as part of the whole extract (Dai et al. 2010).
Evaluation of the reported effective doses for whole pomegranate extracts versus ellagic acid provides considerable evidence for synergistic interactions between naturally occurring phytochemicals in pomegranates. Pomegranate extracts proved to be effective in doses between 50 and 200 μg/mL, providing an ellagic acid content between 0.15 and 5.4 μg/mL (Table 4 ). In contrast, the IC50s for ellagic acid were reported to be between 22 and 462 μM, correlating to 6.5–139.6 μg/mL (Table 3), which are substantially higher, thus lending support to our idea that greater anticancer activity can be observed with whole foods than with constituents alone.
2.3 What Tomatoes Truly Bring to the Table: Juicy Facts
According to the United States Department of Agriculture, the average American consumes more than seventy pounds of tomato products each year, primarily in the form of sauces and other processed tomato products (Lucier 2011). Numerous epidemiological studies investigating diet and disease have suggested that consumption of tomato products or the carotenoid lycopene from tomatoes is associated with a reduced risk of cancer (Campbell et al. 2004; Chan et al. 2006, 2009; Etminan et al. 2004; Giovannucci et al. 2007). Multiple analyses of the prospective Health Professional’s Follow-up Study has uncovered an inverse relationship between tomato product intake and prostate cancer (PC) suggesting a significantly lower risk of PC for subjects who consumed two to four servings of tomato sauce per week (Giovannucci et al. 2007). A more recent report from a prospective hospital-based multicenter case–control study also demonstrated an inverse relationship between tomato/tomato products and PC (Salem et al. 2011). Additionally, tomato was found to significantly reduce the odds ratio of pancreatic cancer (Jansen et al. 2011). While no causality has been proven, the available data collectively support the idea that consumption of tomatoes and tomato products containing lycopene may have anticarcinogenic activity. Mounting evidence from cellular and animal studies in addition to human clinical trials seems to validate the continued research into this commonly consumed food. While variations exist dependent on cultivar, growing conditions, and processing, tomatoes contain carotenoids, flavonoids, and other important phytochemicals like phytoene, phytofluene, beta-carotene, lycopene, quercetin, kaempferol, and vitamins (Capanoglu et al. 2008). As observed with apples and pomegranates, the presence and concentration of compounds can be vastly different in the flesh as opposed to the skin of tomatoes and inclusion of the tomato skin and seeds is important to the phytochemical content, antioxidant potential, and the associated anticancer properties (Capanoglu et al. 2008).
2.3.1 Lycopene: The Red Carotenoid
Much of the early tomato research focused on lycopene, a fat-soluble carotenoid providing the red pigment found in red tomatoes, watermelon, and other fruits (Kelkel et al. 2011). There is agreement that lycopene is a powerful antioxidant, often referred to as the most efficient carotenoid quencher of singlet oxygen (Di Mascio et al. 1989). In addition to antioxidant properties, lycopene displays potent anticancer activity including inhibition of cell growth, anti-inflammatory effects, cell cycle arrest, and alteration of cell signaling pathways (Kelkel et al. 2011). Interestingly, absorption of lycopene is affected by cooking as heat processing results in higher levels of cis-lycopene, the form found in human plasma, as opposed to the trans configuration dominant in fresh tomatoes (Tan et al. 2010). Raw tomatoes have an estimated lycopene concentration of 8.8–42 μg/g, whereas the range for tomato juice and tomato sauce is 86–100 and 63–131 μg/g, respectively (Russo et al. 2010). The bioavailability of lycopene can also be increased with the addition of lipids to the processing or ingestion of tomato products (Tan et al. 2010). However, there seems to be a maximum level of absorption regardless of the dose administered, suggesting the presence of saturable absorptive mechanisms (Diwadkar-Navsariwala et al. 2003). Keeping these factors in mind, cellular, animal, and human studies have provided considerable evidence for chemoprevention by lycopene.
Lycopene has been shown to significantly inhibit the proliferation of prostate, liver, lung, breast, and colon cancer cell lines with inhibition of at least 50 % cell growth for doses between 1.3 and 50 μM, concentrations that are well above the reported serum lycopene concentration of 0.34 and 0.65 μM achievable upon acute lycopene supplementation (Burgess et al. 2008; Diwadkar-Navsariwala et al. 2003; Hwang and Bowen 2005a, b; Hwang and Lee 2006; Koh et al. 2010; Salman et al. 2007; Tang et al. 2008; Palozza et al. 2010; Takeshima et al. 2014; Asmah Rahmat et al. 2002). Lycopene may exert more suppressive activity in androgen-independent prostate cancer cells as demonstrated by IC50s of 26.6 and 40.3 μM compared to 168.5 μM for an androgen-dependent cell line (Tang et al. 2005). These studies are summarized in Table 5. However, several limitations exist in assessing the in vitro efficacy of tomato products containing lycopene as they are particularly sensitive to light, oxygen, and temperature in addition to their incompatibility as hydrophobic molecules in aqueous culture media (Ford et al. 2011; Hwang and Bowen 2005a).
Table 5
Antiproliferative activity of lycopene or tomatine
Tomato phytochemical | Cell line | Reported IC50 (μM) | Concentration (in μg/mL) | Citations |
---|---|---|---|---|
Lycopene | Breast MCF-7 | 42.8 | 22.9 | Takeshima et al. (2014) |
Lycopene | Breast SK-BR-3 | 41 | 22 | Takeshima et al. (2014) |
Lycopene | Breast MDA-MB-468 | 41.7 | 22.4 | Takeshima et al. (2014) |
Lycopene | Breast H-Ras MCF10A | 1.3 | 0.698 | Koh et al. (2010) |
Lycopene | Breast MDA-MB-231 | 13.4 | 7.19 | Koh et al. (2010) |
Lycopene | Colon HT-29 | 10 | 5.37 | Tang et al. (2008) |
Lycopene | Prostate DU-145 | 26.6 | 14.28 | Tang et al. (2005) |
Lycopene | Prostate PC-3 | 40.3 | 21.64 | Tang et al. (2005) |
Lycopene | Prostate LNCaP | 168.5 | 90.46 | Tang et al. (2005) |
Lycopene | Liver HepG2 | 42.4 | 22.8 | Rahmat et al. (2002) |
Lycopene | Breast MDA-MB-231 | 21 | 11.3 | Rahmat et al. (2002) |
α-Tomatine | Liver HepG2 | 41.6 | 43 | Friedman et al. (2009) |
α-Tomatine | Breast MCF-7 | 4.9 | 5.1 | Friedman et al. (2009) |
Animal experiments have reinforced the link between lycopene and reduced risk of carcinogenesis. Androgen-independent xenograft prostate tumor growth rate in rats was significantly reduced from ~50 to 76 % by oral ingestion of lycopene with a correlating reduction in tumor volume of ~43–81 % for doses between 10 and 300 mg/kg (Tang et al. 2005). Oral consumption of lycopene has also been found to inhibit hepatoma tumor metastasis and reduce colon cancer tumor growth in nude mice models in addition to upregulating antioxidant and immune system activity in a gastric cancer rat model (Huang et al. 2008; Luo and Wu 2011; Tang et al. 2011). Human clinical studies of lycopene are under way and a number of these studies investigating lycopene’s purported anticancer benefits will likely provide valuable information regarding absorption, disposition, and any chemopreventive or therapeutic properties of lycopene.
The anticarcinogenic properties of tomatoes have also been attributed to flavonoids, which have individually displayed antiproliferative effects but when combined with other flavonoids the effects were far more striking, strongly suggestive of additive interactions between these phytochemicals (Campbell et al. 2004; 2006). Another line of research investigated the tomato glycoalkaloid referred to as tomatine, found in green tomatoes, with promising initial results (Friedman et al. 2009).
2.3.2 Whole Tomatoes: The Ripened Efficacy
A 2007 review of earlier clinical trials concluded that while lycopene containing tomato products are significantly beneficial, the effects are not the result of lycopene exclusively, but rather the combination of phytochemicals and other components in the whole tomato (Basu and Imrhan 2007). Research on whole tomatoes and the phytocomplexity found naturally in tomatoes has provided support for this conclusion. As observed with other foods reviewed here, the results have suggested that superior benefits may be realized by utilizing the whole tomato (Fig. 3) as opposed to selectively using only one component.
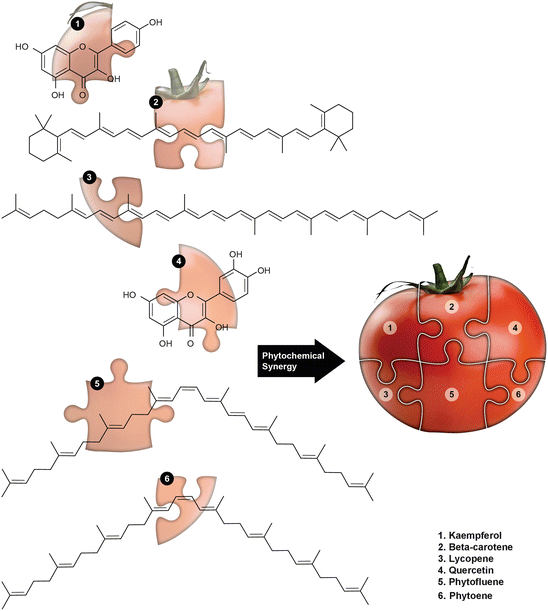
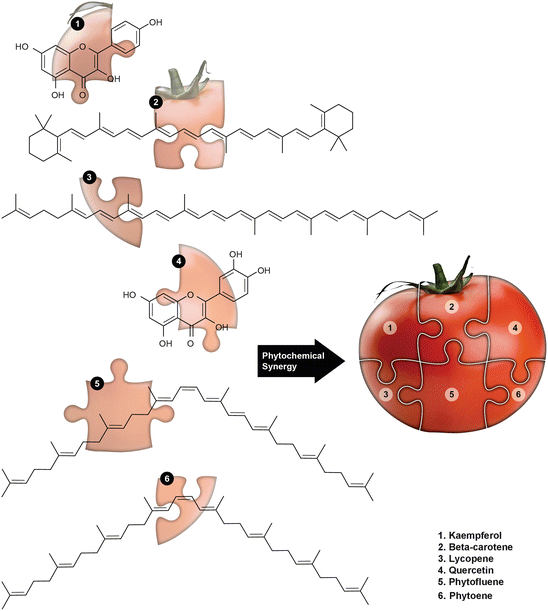
Fig. 3
Tomato is to salsa as phytocomplexity is to well-being: The excellent chemotherapeutic and chemopreventive benefits offered by a whole tomato are the result of phytochemical synergy activated when its constituents including kaempferol, β-carotene, lycopene, quercetin, phytofluenes, phytoenes, etc., fit together as pieces in a puzzle
Colon adenocarcinoma cell growth was inhibited and apoptosis induced in a dose-dependent manner by tomato digestate from in vitro simulated digestion of a tomato genetically engineered to be β-carotene rich (Palozza et al. 2009). A dose of 100 mL/L of tomato digestate inhibited cell growth by 55 %. This dose corresponds to a β-carotene concentration of 0.08 μM, but when purified β-carotene was compared in an identical assay, doses as high as 10 μM did not inhibit cell growth as effectively as tomato digestate, suggesting the involvement of other compounds or processes (Palozza et al. 2009). LycoC, a lycopene phytocomplex with 6 % purified lycopene, inhibited HL60 leukemia cells at concentrations between 10 and 25 μM which corresponds to a lycopene concentration of 0.6–1.5 μM (Ettorre et al. 2010). Explorations of extraction methods and cytotoxity in HT29 colon cancer cells determined the IC50s of 62.5 μg/mL for the petroleum ether extract and 87.0 μg/mL for an in vitro digested tomato extract (Guil-Guerrero et al. 2011). In another study, Bhaumik et al. have performed cell growth inhibition studies employing a variety of tomato extracts prepared in various solvents including methanol, ethanol, acetone, and chloroform against HeLa cells. Results from this study revealed that these tomato extracts exhibited moderate efficacy with IC50 values ranging from 57 to 69 μg/mL (Bhaumik 2014).
A comparison of the effects on human sera of ingestion of lycopene-rich red tomatoes (RTS), lycopene-free yellow tomatoes (YTS), and purified lycopene (LYS) confirmed similar lycopene concentrations in RTS and LYS sera, but produced some surprising results in terms of anticarcinogenic gene expression (Talvas et al. 2010). While increases in beneficial gene expression were observed in RTS and YTS, decreases of anticarcinogenic and increases of pro-carcinogenic gene expression were revealed with evaluation of LYS sera samples, suggesting that the food matrix in whole tomatoes may provide positive benefits and modulate negative effects. While cell proliferation was unaffected by any of the samples at physiological levels, it may be important to note that cells in this experiment were exposed to the human subject sera for only 24 h while a number of other studies have utilized 48 h or longer exposures (Talvas et al. 2010).
Not all investigations into the anticancer properties of tomatoes have focused on lycopene. Research of the isolated green tomato glycoalkaloid tomatine revealed growth inhibition of stomach, liver, colon, and breast cancer cell lines when treated with purified α-tomatine. Inhibition was also observed in normal liver cells. When extracts of a variety of green tomatoes were tested, a number of them were also found to inhibit these cell lines. A comparison of the IC50s for purified tomatine and the quantities of tomatine in the green tomato extracts suggests that the blend of glycoalkaloids found in these tomatoes is more effective than the individual component. Results for HepG2 liver cancer cells and MCF-7 breast cancer cells are shown in Tables 5 and 6.
Table 6
Antiproliferative activity of tomato extract
Substance evaluated | Cell line | Reported IC50 (μg/mL) of tomato product | Contribution of phytochemical to IC50 (μg/mL) of whole tomato extract | Citations |
---|---|---|---|---|
Lycopene | ||||
LycoC (6 % lycopene) | Leukemia HL60 | 5.37–13.4 | 0.32–0.8 | Ettorre et al. (2010) |
Petroleum ether extract of tomato | Colon HT-29 | 62.5 | 0.0146 | Guil-Guerrero et al. (2011) |
Tomato digestate | Colon HT-29 | 87 | 0.0204 | Guil-Guerrero et al. (2011) |
Ethanolic extract of tomato | Cervical HeLa | 68.2 | 3.75 | Bhaumik et al. (2014) |
α-Tomatine | ||||
Sancheri, small green | Liver HepG2 | 12.3 | 0.00386 | Friedman et al. (2009) |
Sancheri, medium green | Liver HepG2 | 3.2 | 0.0003456 | Friedman et al. (2009) |
Sancheri, large green | Liver HepG2 | 1 | 0.0000575 | Friedman et al. (2009) |
Yoyo, green tomato | Liver HepG2
![]() Stay updated, free articles. Join our Telegram channel![]() Full access? Get Clinical Tree![]() ![]() ![]() |