Plasma coagulation in the activated partial thromboplastin time assay is initiated by sequential activation of coagulation factors XII, XI, and IX. While this series of proteolytic reactions is not an accurate model for hemostasis in vivo, there is mounting evidence that factor XI and factor XII contribute to thrombosis, and that inhibiting them can produce an antithrombotic effect with a small effect on hemostasis. This article discusses the contributions of components of the intrinsic pathway to thrombosis in animal models and humans, and results of early clinical trials of drugs targeting factors IX, XI, and XII.
Key points
- •
The term intrinsic pathway refers to a series of sequential reactions involving the plasma proteins factors VIII, IX, XI, and XII; prekallikrein; and high-molecular-weight kininogen, which are required for initiation of coagulation in the activated partial thromboplastin time assay.
- •
Certain components of the intrinsic pathway that serve a limited role in hemostasis (factor XI), or are not required for hemostasis (factor XII, prekallikrein, and high-molecular-weight kininogen), are required for clot formation in animal models of thrombosis.
- •
Epidemiologic data indicate that factor XI contributes to venous thromboembolism and ischemic stroke, and may contribute to myocardial infarction in humans, whereas factor XII likely contributes to thrombus formation when blood is exposed to artificial surfaces, such as during cardiopulmonary bypass and extracorporeal membrane oxygenation.
- •
Reducing factor XI level to 20% of normal by antisense oligonucleotide technology was more effective than standard-dose low-molecular-weight heparin in preventing venous thrombosis during knee replacement surgery, without comprising intraoperative or postoperative hemostasis.
- •
By targeting components of the intrinsic pathway of coagulation with therapeutic inhibitors, it may be possible to uncouple antithrombotic effects from anticoagulant (antihemostatic) effects, improving the safety of antithrombotic therapy.
Introduction
The protease thrombin makes essential contributions to hemostasis through its capacity to catalyze conversion of fibrinogen to fibrin, to stimulate platelet and vascular endothelial cells, and to activate plasma coagulation factors. Thrombin also plays a central role in thrombosis, and several approaches have been developed to manipulate this enzyme to achieve an antithrombotic effect. The activity of thrombin or factor (f) Xa (the enzyme responsible for converting prothrombin to thrombin) can be inhibited directly with drugs targeting the enzyme active sites (argatroban, dabigatran, and bivalirudin for thrombin; rivaroxaban, apixaban, and edoxaban for fXa), or indirectly with heparin-related compounds (unfractionated or low-molecular-weight heparin or fondaparinux) that enhance the activity of the plasma inhibitor antithrombin. Alternatively, synthesis of prothrombin and fX, the precursors of thrombin and fXa, can be reduced with vitamin K antagonists such as warfarin. Use of these effective antithrombotic strategies comes with a well-recognized cost. Thrombin and fXa serve vital roles in hemostasis, and therapies directed at them increase bleeding. Use of heparin is associated with major bleeding rates of up to 3%, and 2% to 13% with warfarin. Newer oral thrombin and fXa inhibitors seem to cause less bleeding than the older drugs, and are easier to use. However, because of their mechanisms of action, there are limits on the types of patients who are eligible to receive them, the clinical settings in which they can be used, and the intensity of anticoagulation that can be applied.
The strategy of targeting thrombin and/or fXa to achieve an antithrombotic effect is based on the intuitive notion that formation of an intravascular thrombus is largely the result of dysregulation of processes normally involved in hemostasis. This premise is currently being reconsidered. There is substantial interest in developing and testing novel therapies that target the proteases of the plasma intrinsic pathway of coagulation (fIX, fXI, fXII, and prekallikrein [PK]) for treating or preventing thromboembolic disorders. The physiologic importance of the intrinsic pathway has been questioned since the original descriptions of the cascade-waterfall model of coagulation because, although some components of the pathway are clearly required for hemostasis, others are not. This article reviews preclinical and clinical data supporting the hypothesis that components of the intrinsic pathway, and perhaps the intrinsic pathway itself, contribute to thrombosis, and that a useful antithrombotic effect can be achieved by targeting plasma factors that serve minor roles in hemostasis. To understand how the intrinsic pathway might contribute to thrombosis, we first need to review how understanding of this pathway has evolved over the past 50 years.
Introduction
The protease thrombin makes essential contributions to hemostasis through its capacity to catalyze conversion of fibrinogen to fibrin, to stimulate platelet and vascular endothelial cells, and to activate plasma coagulation factors. Thrombin also plays a central role in thrombosis, and several approaches have been developed to manipulate this enzyme to achieve an antithrombotic effect. The activity of thrombin or factor (f) Xa (the enzyme responsible for converting prothrombin to thrombin) can be inhibited directly with drugs targeting the enzyme active sites (argatroban, dabigatran, and bivalirudin for thrombin; rivaroxaban, apixaban, and edoxaban for fXa), or indirectly with heparin-related compounds (unfractionated or low-molecular-weight heparin or fondaparinux) that enhance the activity of the plasma inhibitor antithrombin. Alternatively, synthesis of prothrombin and fX, the precursors of thrombin and fXa, can be reduced with vitamin K antagonists such as warfarin. Use of these effective antithrombotic strategies comes with a well-recognized cost. Thrombin and fXa serve vital roles in hemostasis, and therapies directed at them increase bleeding. Use of heparin is associated with major bleeding rates of up to 3%, and 2% to 13% with warfarin. Newer oral thrombin and fXa inhibitors seem to cause less bleeding than the older drugs, and are easier to use. However, because of their mechanisms of action, there are limits on the types of patients who are eligible to receive them, the clinical settings in which they can be used, and the intensity of anticoagulation that can be applied.
The strategy of targeting thrombin and/or fXa to achieve an antithrombotic effect is based on the intuitive notion that formation of an intravascular thrombus is largely the result of dysregulation of processes normally involved in hemostasis. This premise is currently being reconsidered. There is substantial interest in developing and testing novel therapies that target the proteases of the plasma intrinsic pathway of coagulation (fIX, fXI, fXII, and prekallikrein [PK]) for treating or preventing thromboembolic disorders. The physiologic importance of the intrinsic pathway has been questioned since the original descriptions of the cascade-waterfall model of coagulation because, although some components of the pathway are clearly required for hemostasis, others are not. This article reviews preclinical and clinical data supporting the hypothesis that components of the intrinsic pathway, and perhaps the intrinsic pathway itself, contribute to thrombosis, and that a useful antithrombotic effect can be achieved by targeting plasma factors that serve minor roles in hemostasis. To understand how the intrinsic pathway might contribute to thrombosis, we first need to review how understanding of this pathway has evolved over the past 50 years.
The intrinsic pathway in models of blood coagulation
The Cascade-Waterfall Model of Thrombin Generation
The cascade-waterfall hypotheses of intrinsic coagulation was first proposed in 2 landmark articles in 1964 by Macfarlane, and by Davie and Ratnoff. In subsequent models based on this scheme, the process of thrombin generation is the result of amplification of a procoagulant signal initiated by conversion of fXII to fXIIa, followed sequentially by activation of the enzyme precursors fXI, fIX, fX, and prothrombin ( Fig. 1 A ). At the time this model was proposed, it was recognized that much of the cascade could be bypassed through a process involving fVIIa (see Fig. 1 A), but the importance of this was not clear. The scheme in Fig. 1 A depicts the major enzyme reactions that contribute to plasma clotting in the activated partial thromboplastin time (aPTT) and prothrombin time (PT) assays used in clinical practice. There are 2 triggering mechanisms that converge at the level of fX activation. Activation through the intrinsic pathway (see Fig. 1 A, yellow arrows) is assessed by the aPTT assay, and is initiated by a process involving fXII called contact activation (discussed later). In the PT assay, coagulation is triggered through the extrinsic pathway by adding tissue extracts to the plasma that contain tissue factor (TF), a cofactor for fVIIa.
The sequential steplike nature of the coagulation cascade implies that complete deficiency of any component would break the reaction chain and cause bleeding, but this is not what is observed in clinical practice. Absence of fIX or its cofactor fVIII causes the severe bleeding disorder hemophilia, implying an important role for the intrinsic pathway in hemostasis. However, complete fXII deficiency, despite causing a marked prolongation of the aPTT, does not cause abnormal bleeding, whereas fXI deficiency causes a mild hemorrhagic disorder that involves tissues distinct from those commonly affected in fIX or fVIII deficiency. These observations indicate that the classic intrinsic pathway does not accurately describe the manner in which its components contribute to hemostasis, and have led to revisions in the models that are more in line with clinical phenotypes.
Tissue Factor–initiated Thrombin Generation: The Role of Factor XI
It is the current consensus that thrombin generation at a site of vascular injury is initiated primarily by fVIIa in complex with TF ( Fig. 1 B), an integral membrane protein expressed on cells underlying blood vessel endothelium. FVIIa/TF initiates coagulation by activating fX, as in the PT assay (see Fig. 1 A), and also activates fIX, which sustains fXa and thrombin production. The protease precursors (prothrombin, fVII, fIX, and fX) and cofactors (TF, fVa, and fVIIIa) shown within the gray area in Fig. 1 B form the core mechanism for thrombin generation in almost all vertebrate organisms. Total deficiency of any one of these proteins results in a severe bleeding disorder or is not compatible with life. This core mechanism is the target of all currently approved anticoagulants. Thus, it is to be expected that use of these compounds will increase bleeding risk.
In addition to the core proteins, mammals have fXI, the precursor of the protease fXIa (see Fig. 1 B). Severe fXI deficiency may cause excessive bleeding with trauma, particularly if tissues with robust fibrinolytic activity, such as the oropharynx or urinary tract, are involved. However, unlike fIX deficiency, severe spontaneous bleeding is not common in fXI deficiency. Furthermore, bleeding is highly variable, and some fXI-deficient individuals are asymptomatic despite marked abnormalities in the aPTT. Although fXI is activated by fXIIa in the cascade-waterfall model (see Fig. 1 A), the absence of abnormal bleeding in fXII-deficient individuals indicates that other mechanisms for fXI activation must exist. For example, thrombin generated early in coagulation can activate fXI (see Fig. 1 B, green arrow).
In the scheme shown in Fig. 1 B, fXIa sustains thrombin generation by activating fIX, rather than contributing to initiation of thrombin formation, as in the cascade-waterfall model and the aPTT assay. A key feature of the newer model is that there are 2 mechanisms for fIX activation, explaining the differences in bleeding phenotypes associated with fIX and fXI deficiencies. In the absence of fXI, fIX still contributes to thrombin production because it can be activated by factor VIIa/TF. Clinical observations suggest that factor VIIa/TF is probably the more important mechanism for fIX activation. Along similar lines, the different phenotypes associated with fXI and fXII deficiency are explained by the presence of more than 1 mechanism for fXI activation. Current models of thrombin generation often do not include a role for fXII, based largely on the observation that fXII deficiency is not associated with a bleeding diathesis. However, as discussed later, it does not necessarily follow that fXIIa does not contribute to thrombin generation under any circumstances.
The Kallikrein-Kinin System and Contact Activation
The plasma protease precursors fXII and PK and the cofactor high-molecular-weight kininogen (HK) form the plasma kallikrein-kinin system. fXII was first identified as a plasma constituent missing in a patient with a very long aPTT, but without abnormal bleeding. Subsequent work identified PK and HK as necessary for normal fXII activation in the aPTT. When plasma is exposed to artificial surfaces or anionic substances, fXII and PK undergo reciprocal conversion to fXIIa and alpha-kallikrein by a process called contact activation ( Fig. 1 C). HK serves as a cofactor during this process. In the aPTT assay, a purified earth such as silica or Celite is used to induce contact activation, with the resulting fXIIa promoting thrombin generation by activating fXI. The absence of bleeding symptoms in individuals lacking fXII, PK, or HK shows that these proteins are not required for hemostasis, either because they do not normally contribute to thrombin generation or because other mechanisms, such as thrombin-mediated feedback activation of fXI (see Fig. 1 B), compensate for their absence. The kallikrein-kinin system is thought to contribute to several homeostatic and host-defense mechanisms, including inflammation and the innate immune response to microorganisms. It is also clear that fXIIa and fXIa, despite their limited roles in hemostasis, are required for thrombosis in experimental animal models. If these proteins prove to be important contributors to thrombosis in humans, inhibiting them may produce an antithrombotic effect without significantly affecting hemostasis. The current understanding of the intrinsic pathway proteins in thrombosis in animal models and in human populations is discussed later.
The intrinsic pathway and thrombosis
Animal Models of Thrombosis
Mice lacking individual components of the intrinsic pathway, including the kallikrein-kinin system, have been tested for resistance to thrombus formation. FIX-deficient mice have a significant bleeding disorder (the murine equivalent of hemophilia B), and are resistant to thrombus formation induced by injuring blood vessels with concentrated ferric chloride (FeCl 3 ). In contrast, mice lacking fXI or fXII do not have obvious defects in hemostasis. Despite this, counterintuitively, both fXI-deficient and fXII-deficient mice are as resistant to FeCl 3 -induced thrombosis as are fIX-deficient mice. The findings have been corroborated using a variety of other models and methods for inducing thrombosis, and clearly indicate that an anticoagulant (antihemostatic) phenotype is not a prerequisite for an antithrombotic effect, at least under experimental conditions. More recent work has shown that mice lacking PK or HK are also resistant to injury-induced thrombus formation. Overall, these data imply that a process similar to classic contact activation may be driving thrombus formation through the intrinsic pathway in the mouse models.
Studies in primates have also shown roles for fXI and fXII in experimental thrombosis. This work used inhibitory antibodies or antisense oligonucleotides (discussed later) to produce transient deficiency states. Thrombus formation was studied in prothrombotic collagen–coated or TF-coated vascular grafts inserted into temporary arteriovenous shunts in olive baboons ( Papio anubis ). In these studies the antithrombotic effect of fXI inhibition seemed to be greater than that of fXII inhibition. A reduction in thrombus formation was detectable with as little as a 50% reduction in plasma fXI level, with the maximum effect achieved at less than or equal to 20% of normal plasma concentration.
Although the data from the animal studies support the notion that the intrinsic pathway contributes to thrombosis, there are limitations to the preclinical analyses that must be considered. Because the animal species tested do not have natural propensities to form arterial or venous thrombi in the same manner as humans, vessels must be injured in some manner to produce a thrombus. Injury-induced thrombosis in an otherwise healthy, and usually young, animal may not mimic formation of thrombi in the diseased vessels of older humans.
Factor IX and Factor VIII in Thrombosis in Humans
FIX is the precursor of the protease fIXa, and activated fVIII (fVIIIa) is its cofactor. The severe bleeding disorders associated with deficiency of fIX (Hemophilia B) or fVIII (Hemophilia A) show their importance to hemostasis. There is a consistent correlation between plasma levels of fVIII in complex with von Willebrand factor (vWF) and risk for venous and arterial thrombosis in humans. Supporting these observations, individuals with blood group O, who have 25% to 50% lower vWF and fVIII levels than individuals with a non-O blood group, are at lower risk for venous and arterial thrombosis. High plasma levels of fIX are associated with modestly increased risks for venous thromboembolism (VTE), myocardial infarction (MI), and stroke. These findings are supported by the impression that hemophiliacs have a lower risk of arterial thrombosis than nonhemophiliacs.
Factor XI in Thrombosis in Humans
For fXI levels, the strongest correlations are with risk for VTE and ischemic stroke. The 10% of subjects in the Leiden Thrombophilia Study with the highest plasma fXI levels had a 2-fold higher risk of VTE compared with the lower 90%, a result supported by recent data from the Longitudinal Investigation of Thromboembolism Etiology (LITE) cohort. Consistent with these findings, fXI deficiency was associated with a reduced incidence of VTE in a study from Israel, where severe deficiency is present in 1 in 450 individuals. High plasma levels of fXI or fXIa were associated with increased risk for ischemic stroke in a study by Yang and colleagues, in the Atherosclerosis Risk in Communities (ARIC) study, and in the Risk of Arterial Thrombosis In relation to Oral contraceptives (RATIO) study. Similarly, severe fXI deficiency was associated with a reduced incidence of stroke.
A role for fXI in MI is not as clear as for VTE or stroke. Plasma fXI levels correlated with MI risk in men in the Study of Myocardial Infarction Leiden (SMILE), and were higher in women with coronary disease than in women without it in a group undergoing cardiac catheterization. However, fXI was not a risk factor for MI in the ARIC or RATIO studies, and fXIa levels were not linked to coronary disease in the second Northwick Park Heart Study (NPHS-II). The incidence of MI in 96 individuals with severe fXI deficiency was similar to the expected incidence for age-matched controls. The data are in line with recent work by Siegerink and colleagues showing that an increased level of fXIa correlated more closely with risk for ischemic stroke than for MI in young women, and suggest that the contribution of fXI to thrombus formation is greater in some vascular beds than in others.
The Kallikrein-Kinin System in Thrombosis in Humans
Data supporting a role for fXII in VTE, stroke, or MI in humans are weak, and there is insufficient information to assess PK and HK. Note that congenital deficiency of C1-inhibitor (C1-INH), the major plasma regulator of fXIIa and alpha-kallikrein, causes hereditary angioedema, and does not seem to predispose to thrombosis. FXII-deficient individuals are not protected from VTE, and no differences in VTE incidences were noted across a range of fXII levels in LETS and LITE study participants. Data on fXII in arterial thrombosis are conflicting. FXII deficiency does not seem to protect individuals from stroke. Plasma levels of fXIIa were inversely correlated with stroke risk in NPHS-II, but were directly correlated with it in the RATIO study. However, plasma fXII levels did not correlate with stroke risk in either study, or in the ARIC study. In NPHS-II, increased fXIIa levels measured by specific enzyme-linked immunosorbent assay was associated with increased risk of MI, but fXIIa level measured as a complex with C1-INH indicated the opposite effect. In the RATIO study, fXIIa, fXII, and PK levels were not associated with MI, and risk of coronary events did not correlate with fXII in the ARIC study. In addition, data from the SMILE cohort showed an inverse relationship between fXII levels and cardiovascular disease. Endler and colleagues also noted an inverse association between fXII and death from ischemic heart disease, although the relationship did not hold for severe fXII deficiency (<10% of normal), for which the risk was comparable with that of the population mean.
The Intrinsic Pathway and Thrombosis in Humans: Summary
Although it is difficult to draw firm conclusions from the complicated human epidemiologic data, it seems reasonable to conclude that the contribution of fXII to VTE, stroke, and MI in humans is probably smaller than for fXI and fIX. Although this conclusion conflicts with data from mouse models, in which fXII is a major contributor to thrombus formation, it is in reasonable agreement with results obtained in primates. It is possible that feedback activation of fXI by thrombin (see Fig. 1 B) is stronger in primates than in mice, with fXIIa serving a smaller role in fXI activation. Another possibility is that a greater degree of fXIIa inhibition is required to produce an antithrombotic effect comparable with what is seen with fXIa inhibition in primates. These observations notwithstanding, there are situations in which fXIIa is likely to be a major driver of thrombosis in humans. For example, contact activation is triggered when blood is exposed to artificial surfaces during cardiopulmonary bypass and extracorporeal membrane oxygenation (ECMO), requiring full anticoagulation with heparin to prevent thrombotic complications.
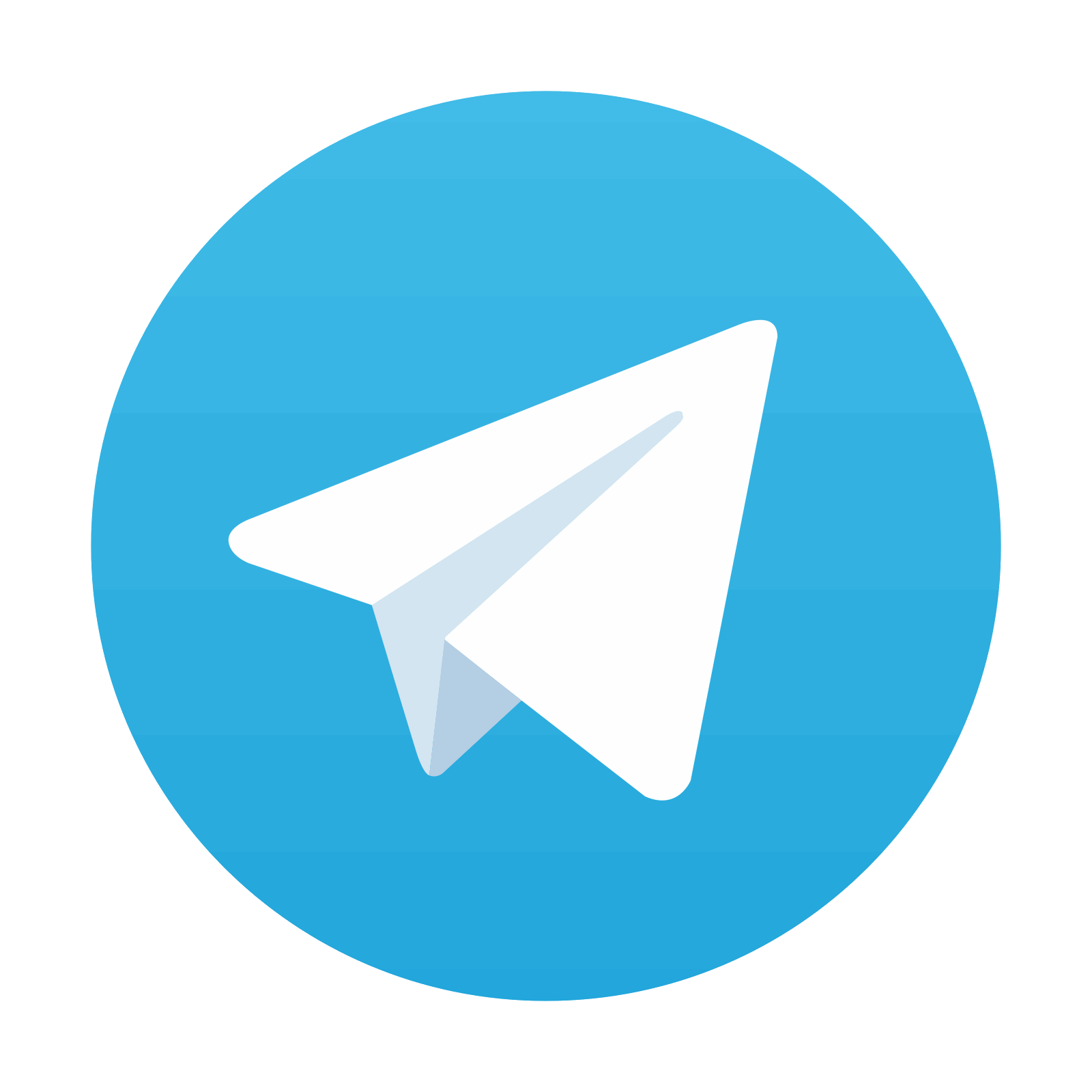
Stay updated, free articles. Join our Telegram channel

Full access? Get Clinical Tree
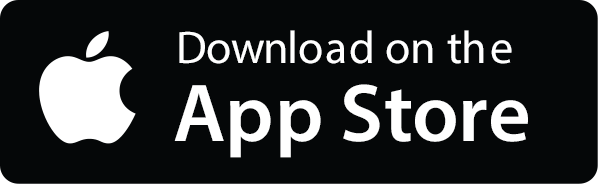
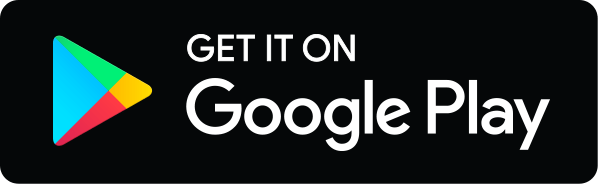