Abstract
The gut microbiome is interlinked with renal cell carcinoma (RCC) and its response to systemic treatment. Mounting data suggests that certain elements of the gut microbiome may correlate with improved outcomes. New generation sequencing techniques and advanced bioinformatic data curation are accelerating the investigation of specific markers and metabolites that could predict treatment response. A variety of new therapeutic strategies, such as fecal microbiota transplantation, probiotic supplements, and dietary interventions, are currently being developed to modify the gut microbiome and improve anticancer therapies in patients with RCC. This review discusses the preliminary evidence indicating the role of the microbiome in cancer treatment, the techniques and tools necessary for its proper study and some of the current forms with which the microbiome can be modulated to improve patient outcomes.
Introduction
The gut microbiome has been recently implicated in multiple diseases, ranging from inflammatory bowel disease to cancer [ , ]. Although a more diverse and balanced gut microbiome correlates with a favorable health profile, it is still unclear what specific elements of its composition are fundamental for tumorigenesis, host protection against neoplasia, and how it may influence tumor progression or treatment response [ ].
Renal cell carcinoma (RCC) is the most common form of kidney cancer, and approximately 81,610 people are projected to be diagnosed with RCC in 2024 [ ]. Patients with RCC have a wide range of local and systemic treatment options, which have contributed to increasing survival rates in recent years [ ]. Previously, the gut microbiome has been linked to kidney disease through the gut-kidney axis [ ]. Gut dysbiosis has been associated with uremic toxin accumulation that leads to the pathogenesis of conditions like chronic kidney disease, hemodialysis, and IgA nephropathy [ ]. Despite the associations found between microbial species and kidney diseases, understanding of the microbiome in RCC is still developing.
RCC is a solid tumor known to have a strong interplay with inflammatory and immunologic mediators [ ]. One of the strongest associations of the gut microbiome with cancer has been in its role of regulating treatment response to immunotherapy [ , ]. For this reason, this review will focus on describing the composition, role, and therapeutic impact the gut microbiome has in RCC.
The microbiome
The human microbiome consists of over one hundred trillion bacteria, viruses, and fungi that colonize and interact with the body. These microbial species and the host have relationships that are symbiotic, mutualistic, and pathogenic in nature, with symbiosis assisting in maintaining physiological processes necessary for healthy living. They inhabit various tissues and fluids, including the skin, mucosa, urogenital tract, and saliva. Among the various sites, the gastrointestinal tract houses the largest community of microbes and is evidenced to have the most impact on health [ , , ].
When characterizing the composition and functions of the gut microbiome, most research focuses on identifying bacterial abundance through genetic sequencing. It is estimated that an individual’s gut microbiome alone carries at least 500 to 1000 different species at any given time [ ]. There is also great diversity in both bacterial strain and genetic composition that exists between each person’s unique microbiome [ ]. Individual variations have been suggested to be attributed to diet, antibiotic use, lifestyle, and environmental exposures, with research continuing to explore other potential associations [ ].
In a healthy human body, the gut microbiome plays various important roles essential for maintenance, growth, and survival. The microbiome synthesizes vitamins, bile acids, amino acids, and trimethylamines used for numerous metabolic and biosynthetic processes [ , ]. Symbiotic bacteria including Firmicutes, Fusobacteria, Actinobacteria, and Bacteroidetes also digest complex carbohydrates and fibers into short chain fatty acids (SCFAs) [ , ]. With the increased availability of SCFAs like acetate, butyrate, and propionate, multiple biochemical pathways benefit from these metabolites, including gluconeogenesis, lipogenesis, and cholesterol metabolism [ , , ].
The microbiome and renal cell carcinoma
Recent studies have begun to explore how the gut microbiome may impact RCC development and treatment outcomes. For example, a study by Chen et al. compared gut microbiota of patients with clear cell RCC and healthy individuals to find that clear cell RCC fecal samples had significantly different microbiome compositions. Blautia, Streptococcus, Ruminococcus, Romboustia, and Eubacterium were enriched genera in the clear cell RCC group while Prevotella, Lachnospira, Lachnoclostridium, and Roseburia were abundant in the healthy group [ ]. Upon further in vitro analysis with Streptococcus lutetiensis alone, this species influenced the TGF-beta signaling pathway to promote cancerous cell activity [ ].
Relating microbial compositions with treatment toxicities, Pal et al. performed a stool bacteriomic profiling in 20 patients with metastatic RCC receiving vascular endothelial growth factor (VEGF) tyrosine kinase inhibitors (TKI) and showed that patients suffering diarrhea had increased levels of Bacteroides species and reduction of Prevotella species , while the opposite was true for patients with no diarrhea. Patients in the study who were treated with antibiotics with coverage for Bacteroides species showed improved progression-free survival, which further indicated the possibility of intervening in the gut microbiome for improving outcomes. This study was a landmark assessment of a possible causative mechanism for VEGF-TKI toxicity and anticipated future interventions for treating this condition [ , ].
The microbiome and the immune system
The gut microbiome influences the host immune system. Several bacterial species and their metabolites have been associated with promoting systemic myeloid and lymphoid immune cell production [ ]. On a local immunity level, gut microbes are responsible for protecting and maintaining intestinal epithelial cell (IEC) structures against pathogenic bacteria and their toxins [ ]. Species like Lactobacillus paracasei have demonstrated to regulate inflammation in the gut, such as through repressing IL-10 levels to decrease local lymphocyte recruitment [ ]. This IEC-protecting role of the microbiome ultimately protects against inflammatory bowel diseases, which has been well supported in past research [ ].
Dysbiosis, or the disruption of symbiotic microbiome compositions, is conversely associated with increased susceptibility to diseases [ , ]. Microbes can secrete ligands or induce epithelial cells through activation of damage sensors to secrete chemokines and cytokines that produce proliferation signals and inflammotory responses [ ]. This intricate relationship of the gut microbiome with the immune system has recently been described as an enabling mechanism for establishing cancer cells with their hallmark capabilities [ ].
Most research has been focused in establishing how gut bacterial species and dysbiosis may be implicated in influencing malignant cell proliferation or response to treatment. Understanding microbiome profiles that may indicate a response to treatment may help clinicians to identify candidates who would most benefit from receiving therapies while avoiding therapy-associated adverse reactions in patients who may receive little to no benefit. Even more, developing techniques to modulate the microbiome could help serve as therapeutic tool towards augmenting treatment response and reversing resistance to therapy [ ].
Modulating the microbiome
In the realm of cancer treatment, numerous strategies are being developed to modulate the microbiome, aiming to either enhance or elicit treatment responses across various cancer types. Some of these strategies include fecal material transplant (FMT), supplementation with prebiotics or probiotics, and limiting patient exposure to proton pump inhibitors (PPI) and antibiotics, all of which will be further discussed in the text [ ].
However, the field grapples with the challenge of moving beyond associative relationships between microbiome biomarkers and treatment outcomes to establish clear causative links [ ]. Understanding and measuring dysbiosis—the imbalance in microbial communities— is a preliminary step toward modulating the microbiome [ ]. To achieve this, one must start with sampling the microbiome, but also properly classifying which of its components influence the most towards the definition of a healthy microbiome. Research has produced contradictory data on whether the abundance of specific bacterial groups or the general composition expressed by diversity indices should be used as markers of microbiome activity in carcinogenesis, immune modulation, and therapy responses [ ]. Also, correct interpretation of the evidence must take into account which strategies are chosen for sampling and sequencing the microbiome. Most studies assessing microbiota-oriented interventions have focused on baseline characteristics of patients’ gut microbiota. However, dynamic studies, in which the gut microbiota is also sampled after treatment is given, have shown that tumor response and progression correlate with changes in the gut microbiome composition towards a healthier or unhealthier state, respectively [ ].
This complexity stems from inherent limitations in studying the gut microbiome, compounded by methodological heterogeneity across different studies [ ]. In the ensuing discussion, we aim to explore some of these challenges in detail.
Diversity indices
In assessing the health of a microbiome population, alpha and beta diversity indices serve as key measures, enabling comparisons both within and between microbiome communities [ ]. Alpha diversity looks at the richness and evenness within a specific community, offering insight into the variety and distribution of species present [ ]. Beta diversity, on the other hand, measures differences between ecological communities. Notably, interventions targeting the microbiome, such as probiotics or dietary changes, might not lead to marked changes in alpha diversity [ ]. However, this lack of change should be cautiously interpreted; it does not necessarily imply the absence of biological activity [ ]. The interplay between richness (the variety of species) and evenness (how evenly those species are distributed) can result in a net unchanged alpha diversity despite underlying shifts. Therefore, the specific nature of an intervention and the baseline state of the environment critically influence the observed impact on alpha diversity [ ].
Stool as a surrogate marker for the gut microbiome
The diversity of the gut microbiome is not only individual-specific but also varies significantly along the gastrointestinal (GI) tract. A study performed by Zmora et al., showed that the presence of probiotic bacteria in stool samples does not necessarily equate to their successful colonization of the gut, as it might merely reflect their passage through the GI tract [ ]. The actual engraftment of probiotics depends on a complex interplay of factors, including the host’s unique microbiome composition and other personal and environmental factors. Consequently, to accurately gauge the impact of bacteria or dietary interventions, sampling strategies would be optimally tailored to reflect the expected mechanisms of action [ ]. In line with this perspective, when bacteria secrete metabolites absorbed in different segments of the gastrointestinal tract, such as the small or large intestine, collecting luminal samples from these sites provides direct insight into metabolic interactions. Conversely, for microbes expected to interact directly with the immune system or epithelial cells, mucosal biopsies would be the ideal sampling site for showcasing such interactions.
Sequencing techniques
The traditional method for identifying microbiome species within a sample relies on 16S rRNA gene sequencing, a widely used approach in microbial ecology. This gene, a component of the 30S small subunit of a prokaryotic ribosome, is chosen for its unique combination of conserved and hypervariable regions. The conserved regions enable the design of universal primers that can amplify the gene across diverse bacterial taxa, while the hypervariable regions provide the sequence diversity necessary for phylogenetic classification [ ].
The technique typically involves polymerase chain reaction (PCR) amplification of the 16S rRNA gene, followed by next-generation sequencing to obtain a comprehensive profile of microbial communities within the sample. While 16S rRNA sequencing offers advantages such as cost-effectiveness and a standardized approach, it is not without limitations [ ]. One notable concern is the potential introduction of biases during the amplification step, which may impact the abundance estimation of specific bacterial groups in the sample. Additionally, 16S rRNA sequencing may lack species-level resolution, restricting identification to higher taxonomic levels and potentially obscuring finer-scale community dynamics. Despite these limitations, 16S rRNA sequencing remains a valuable tool for characterizing microbial communities and elucidating broad patterns of diversity and composition within ecosystems [ ].
Recent advancements in microbiome research have increasingly relied on shotgun metagenomic sequencing to offer a more comprehensive view of microbial community compositions [ ]. Unlike 16S rRNA sequencing, which targets a specific gene, shotgun metagenomics provides DNA sequencing of all microorganisms present in the sample including bacteria, viruses, archaea, and fungi. This approach offers higher species resolution, allowing for the identification of distinct microbial taxa within the community. This granularity is crucial as even bacteria from the same genus can exhibit diverse phenotypic behaviors and interactions with the host [ , ].
Despite its advantages, shotgun metagenomic sequencing comes with its own set of challenges. While the cost of this technique has been gradually decreasing, it remains more expensive compared to 16S rRNA sequencing. Additionally, the storage and curation of large sequencing datasets pose logistical and computational challenges, increasing the complexity and cost of analysis. Moreover, the accurate identification of less-studied microbes relies heavily on comprehensive bioinformatics pipelines and up-to-date reference databases. These factors necessitate careful consideration and robust infrastructure to ensure the reliability and validity of results obtained through shotgun metagenomic sequencing [ ].
One of the key advantages of shotgun metagenomic sequencing is its ability to provide functional insights into the metabolic pathways within a microbiome community [ ]. For instance, a study by Frankel et al. in melanoma patients undergoing immunotherapy revealed that those with positive treatment responses showed increased levels of anacardic acid, a dietary molecule rather than a microbial metabolite, underscoring the importance of diet and metabolism in therapy outcomes [ ]. This contrasts with the lack of observed increase in inositol metabolites, previously thought to be associated with treatment response, highlighting the complexity of the microbiome’s role in cancer therapy and the need for comprehensive metabolic profiling.
The next sections will discuss emerging microbiota-oriented interventions and further describe how the microbiome affects outcomes of patients with cancer. Figure 1 showcases an illustrative scheme of the strategies discussed in the following sections.
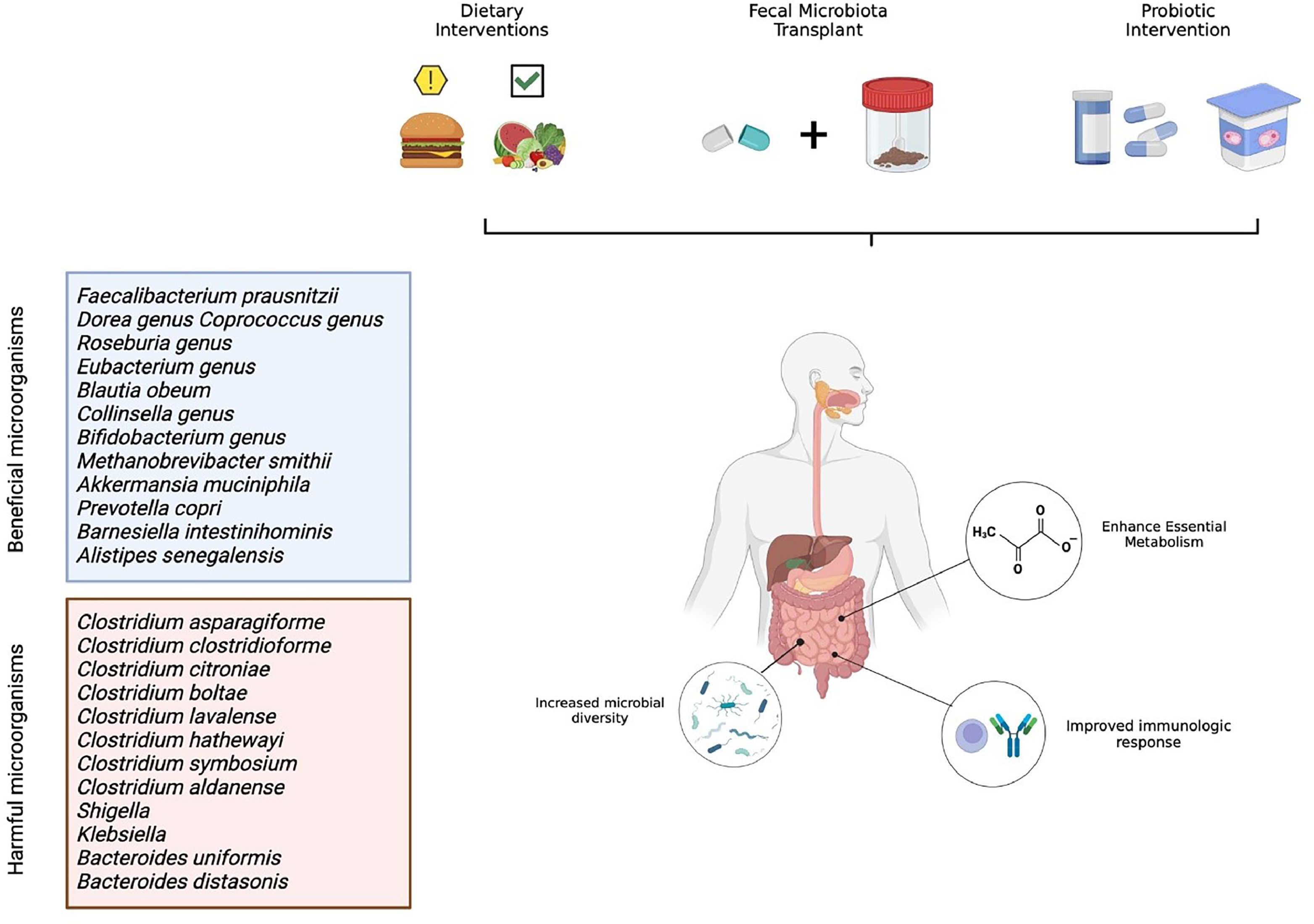
Fecal microbiota transplant as a therapeutic approach for RCC
Fecal microbiota transplantation (FMT) is arguably the strongest intervention to modify the gut microbiome composition in patients. This process consists of transplanting fecal material from a screened host to an individual with a disrupted microbiome composition, either through colonoscopy or oral capsules. As this process has long been established as a safe and efficacious method in the treatment of long-standing refractory Clostridioides difficile infection, FMTs have extended to multiple diseases and neoplasms evidenced to be influenced by the immune system [ ].
The intrinsic relationship between the gut microbiomes´ composition and cancer development was first introduced in 2015, when Vétizou et al. showed results that antibiotic-treated, germ-free mice elicited poor responses to anti-CTLA4 therapy. When this group of mice was treated with Bacteroides species , the anti-CTLA4 response was recovered, as demonstrated by a more potent Th1 immune response [ ]. More recently, newer clinical studies evaluated the relation and effect that gut microbiota composition has on renal cell carcinoma [ ]. Derosa et al. studied the role that microbiota composition had on 69 patients with RCC receiving treatment with nivolumab. They concluded that a higher diversity of Bacteroides salyersia, Eubacterium siraeum, and Akkermansia muciniphilia were associated with higher responses to immunotherapy [ ]. Similarly enough, abundance of Akkermansia muciniphila has also been implicated in dendritic cell activation and CD4 effector function, thereby inducing a stronger immune response against the primary tumor [ , ]. Salgia et al. sought to characterize the stool microbiome composition of 31 patients with metastatic renal cell carcinoma (mRCC) before and after receiving treatment with nivolumab or nivolumab plus ipilimumab. Their results showed that abundance of Barnesiella intestinihominis, Bifidobacterium adolescentis, Odoribacter splanchnicus, Bacteroides eggerthii and Akkermansia muciniphilia were associated with higher response rates on both treatment arms, showing the benefit of microbiota diversity with immune therapy response [ ]. Although concrete results from longitudinal, randomized, clinical trials evaluating the effect of FMT in RCC treatments are still ongoing, there is already evidence suggesting it can improve outcomes. In a randomized control trial performed in 2020 by Ianiro et al., 20 patients with mRCC were randomized to receive FMT from healthy donors or a placebo to evaluate if this intervention could treat TKI-induced diarrhea. Results showed that FMT is superior to placebo in treating TKI-associated diarrhea, with no serious adverse events observed in either treatment arm [ ]. In 2022, Ninjov et al. assessed peripheral blood mucosa-associated invariant T (MAIT) cell frequencies and functions in patients with mRCC before and after FMT. Analysis showed that FMT was associated with upregulation of MAIT cell population and decreased levels of PD-1, which suggests that FMT improved MAIT cell functions and allowed them to mount a stronger immunologic response [ ]. Of note, the TACITO trial (NCT04758507) seeks to evaluate the efficacy of FMT from donors who are responding to ICI with the goal of improving response rates to ICIs in patients with advanced RCC. The PERFORM trial (NCT04163289) seeks to evaluate the efficacy of FMT in reducing immunotherapy-related adverse events in patients with intermediate and poor risk RCC on ipilimumab and nivolumab.
All results support the strengthening notion that modulating the gut microbiome could work as an effective and safe therapeutic weapon in the arsenal against RCC. More clinical trials are currently undergoing that seek to better answer this question and clarify the nuances around this topic. However, the current research precedent is already showing promising results.
Prebiotic interventions as a therapeutic approach for RCC
Prebiotics are nondigestible food components that selectively stimulate the growth and activity of beneficial gut bacteria, such as Bifidobacteria and Lactobacilli . Prebiotics are typically types of dietary fiber that the human body cannot digest, which include inulin, fructooligosaccharides (FOS), and galactooligosaccharides (GOS) [ , ].
Historically, diet has been strongly implicated in the gut microbiomes’ composition. High-fat, low-fiber diets have been associated with a lower microbiome diversity and decreased immunomodulative effect [ ]. Currently, ongoing clinical trials seek to evaluate how different dietary interventions can potentially affect the effectiveness of immunotherapy on a range of solid neoplasms (NCT03700437, NCT03950635 and NCT04909034). Albeit, RCC-tailored clinical trials are still an undiscovered area of opportunity [ ].
On a similar note, camu camu, an Amazonian fruit, has been shown to increase the density of the Ruminococcus species and increase the immunomodulative effect of the gut microbiome [ ]. There is currently an ongoing trial that seeks to evaluate the effect that camu camu in combination with nivolumab/ipilimumab has on patients with mRCC (NCT06049576). Previous evidence has shown that in patients with melanoma, the presence of bacteria from the Ruminococcaceae family were associated with disease response to anti-PD1 inhibitors [ ]. The same researchers showed that patients who had high dietary-fiber intake had the best outcomes and a numerical trend of increased Ruminococcaceae bacteria was found in these patients gut microbiome, although this finding was not statistically significant [ ]. Paradoxically, patients who took commercial probiotics containing Bifidobacterium longum or Lactobacillus rhamnosus had the opposite effect, which cautions against generalizations of cause and correlation in microbiome studies [ ]. When feeding germ-free mice with high fiber diets, the researchers found that their tumors after anti-PD-L1 treatment harbored an increased level of CD4 T cells and an interferon response gene expression profile, which further indicates the role of fiber-fermenting Ruminococcaceae family of bacteria in the immune response against malignancies [ ].
Antibiotics
Routy et al. recently developed a study in which they evaluated 100 patients, 60 with nonsmall cell lung cancer and 40 with renal cancer, who received PD-1 inhibitors. When patients were given antibiotics prior to initiating treatment, quinolones and broad-spectrum B lactams were notably associated with gut dysbiosis and poor response to treatment. Specifically, antibiotic use was associated with worsening progression-free survival (HR 3.1, CI 95%, 1.4–6.9, P < 0.01) and primary progressive disease (75% versus 22%, P < 0.01) [ ]. In 2022, Luo et al. published a systematic review in which they included 6 studies (1,104 patients) that evaluated the effect that antibiotics have on immunotherapy in patients with RCC. They found that antibiotic use correlated with shorter PFS (HR = 2.10, 95% CI [1.54; 2.85], I2 = 2%), shorter overall survival (OS) (HR = 1.69, 95% CI [1.34; 2.12], I2 = 25%) and worse overall response rate (ORR) (OR = 0.58, 95% CI [0.41; 0.84]) [ ].
Of note, authors concluded that while concomitant use of antibiotics and immunotherapy seem to be detrimental for effectiveness of the latter, further studies evaluating appropriate timing of antibiotic administration may provide a solution for this conundrum. These results are particularly problematic as patients with RCC, and patients with cancer in general, often have infectious complications during the course of their treatment which warrant the use of broad-spectrum agents. More caution should be elicited to the selection of antibiotics in these scenarios, as unnecessary or aggressive use of them could potentially hinder the beneficial effects of immunotherapy on the primary neoplasm.
Proton pump inhibitors
Proton pump inhibitors (PPIs) are another class of medication that has also been implicated as a potential modifying agent in the gut’s microbiota composition. Presumably, PPI induced changes of the gut microbiome should be related to decreased stomach acidity and increased representation of oral bacteria, which would not otherwise survive the acidic environment. This hypothesis was supported by Imhann et al. in a study in which they used 16S rRNA gene sequencing in oral and stool samples from 211 patients to find an association of PPI use with reduced alpha diversity index and overrepresentation of oral-microbiota bacteria in the gut, represented by genera Enterococcus, Streptococcus, Staphylococcus and the potentially pathogenic species Escherichia coli [ ] . A different study also showed increased correlation of Streptococcus species associated with PPI use [ ].
However, a meta-analysis of 41 retrospective studies demonstrated the negative effect of PPI on OS outcomes in patients with multiple malignancies, individual studies in the mRCC setting did not show the same effect [ ]. In a multicenter, retrospective study carried out by Mollica et al., patients with mRCC treated with nivolumab or nivolumab and ipilimumab who were concomitantly prescribed PPIs were analyzed. The authors found no difference in objective response rate, progression-free survival or overall survival in both arms, showing that PPI use concomitantly with immunotherapy did not seem to affect survival [ ]. Of note, another retrospective study performed by Yin et al. analyzed 176 patients with mRCC treated with ipilimumab and found that although immunotherapy effectiveness was not hindered by concomitant use of PPI, chronic exposure to PPIs (> 8 weeks) was significantly associated with suffering immunotherapy-related colitis [ ]. Although PPI usage has been demonstrated to alter the composition of the gut microbiome, its impact on anticancer therapy is still not clear.
Probiotic interventions as a therapeutic approach for RCC
The Food and Agriculture Organization of the United Nations and the World Health Organization define probiotics as “live microorganisms which when administered in adequate amounts confer a health benefit on the host” [ ]. This intervention of prescribing a specific microorganism to patients that can, in theory, booster the gut´s microbiome and improve the response to immunotherapy has been a popular topic in recent years.
Specifically, Dizman et al. designed the first prospective study to evaluate the impact of a probiotic intervention on anticancer therapy for mRCC. In their study, they assessed if a Bifidobacterium-containing yogurt product could modulate the gut microbiome and treatment outcomes in patients with mRCC initiating VEGF-TKI therapy, regardless of the line of treatment they were on. Results showed that in patients with clinical benefit, Barnesiella intestinihominis and Akkermansia muciniphila were significantly more abundant compared with patients that showed no clinical benefit [ ]. In 2022, the same authors published a similar line of analysis in which 30 treatment-naive patients with mRCC were randomized to receive nivolumab/ipilimumab with or without CBM588. CBM588 is a bifidogenic live bacterial product constituted of a strain of Clostridium butyricum and is postulated to increase response to immune checkpoint inhibitors by modulating the gut microbiome. It was previously shown that bacteria from the Bifidocterium genus were implicated in improved outcomes in patients with mRCC treated with anti-PD1s [ ]. Additional retrospective evidence from Japanese patients with nonsmall cell lung cancer showed improved PFS and OS in patients who had taken CBM588, which was even more pronounced in patients with prior antibiotic exposure [ ]. Between the ipilimumab plus nivolumab and CBM588 group and placebo group, progression-free survival was significantly longer in patients receiving CBM588 (12.7 months versus 2.5 months, hazard ratio 0.15, 95% confidence interval 0.05–0.47, P = 0.001). CBM588 could improve clinical outcomes in this patient population without generating additional toxicities [ ].
Several other ongoing trials are attempting to evaluate the effectiveness of additional probiotic supplements. The MCGRAW study analyzed the safety, tolerability, and clinical outcomes of treatment with SER-401 (an oral probiotic) or matching placebo in combination with nivolumab in participants with unresectable or metastatic melanoma. Most recently they have submitted results to the US Government Clinical Trial Agency and are waiting for quality control approval for publication (NCT03817125).
Likewise, another clinical trial was designed to evaluate the efficacy of MRx0518 in combination with pembrolizumab in patients with a variety of solid tumors, including RCC and bladder cancer. MRx0518 is a strain of Enterococcus gallinarum, which has been shown to be more abundant in patients that respond to checkpoint inhibitors [ ]. This strain is believed to induce its immunostimulant effect by expressing flagellin which is recognized by toll-like receptor 5 (TLR5), that is linked to the interplay of activation of the innate and adaptive immune systems [ ]. Unfortunately, the trial has been terminated early because of sponsor insolvency [ ]. A nonexhaustive list of clinical trials evaluating different therapeutic interventions in RCC is shown in Table 1 .
