Fig. 11.1
The major T cell-based immunotherapeutic targets and their tumor or antigen-presenting cell ligands. The activation of T cells and their conversion to a cytotoxic phenotype is governed by a network of activating and inhibitory receptors. Using immunotherapeutic agents to increase activation and decrease inhibitory signaling has the potential to generate T cells with enhanced tumor lytic capacity. APC antigen-presenting cell, GAL galectin-9, IDO indoleamine 2,3-dioxygenase, ICOS inducible T-cell costimulator, MHC major histocompatibility complex, PD-1 programmed death 1, PD-L PD-1 ligand, TCR T-cell receptor, TIM-3 T-cell immunoglobulin and mucin domain 3
Depleting Tregs
Targeting CD25: Anti-CD25 (Daclizumab) and Denileukin Diftitox
Tregs constitutively express the IL-2 receptor α chain (CD25). Daclizumab (Zenapax®, F. Hoffmann-La Roche) is an FDA-approved humanized IgG1 mAb that binds to CD25. Traditionally used to inhibit T-cell proliferation in autoimmune disorders, it has recently been used to deplete Tregs in combination with a metastatic breast cancer vaccine. Daclizumab administration led to a marked and prolonged decrease in Tregs and boosted T-cell responses to all vaccine antigens in absence of autoimmunity [114]. Daclizumab is currently being evaluated in combination with a DC-based vaccine in ovarian cancer (NCT01132014). Another approach to targeting CD25 is through denileukin diftitox (Ontak®, Eisai), an FDA-approved engineered fusion protein of IL-2 and diphtheria toxin. In a recent phase II trial of 28 patients with epithelial ovarian cancer, denileukin diftitox administration was well tolerated and significantly depleted functional Tregs from blood and the TME, but showed no significant clinical efficacy [115]. Combination strategies with checkpoint blockade may improve clinical efficacy and have shown promise in preclinical studies [116].
Cyclophosphamide
Cyclophosphamide is a chemotherapeutic agent that has immunomodulatory activity when administered in repeated, low doses (metronomically). It depletes Tregs and restores T-cell function and has been used to augment antitumor immune responses of ACT and vaccination strategies [117]. In a phase I/II trial in 11 patients with recurrent ovarian cancer, a single low dose of cyclophosphamide was explored as an adjuvant to a vaccine regimen of peptide-pulsed DCs. While the 3-year OS was 90 %, the single dose of cyclophosphamide did not reduce the number of circulating Tregs, and no significant survival benefit over controls was observed [118]. In another phase II trial in patients with recurrent ovarian cancer, low-dose cyclophosphamide administered prior to each dose of the p53-SLP vaccine led to p53-specific IFN-γ-producing T cells in 90 % of evaluable patients after two immunizations [119].
Checkpoint Blockade
Immune checkpoints tightly regulate the intensity and duration of the T-cell response and are critical for avoiding autoimmunity. These include the T-cell surface molecules cytotoxic T-lymphocyte antigen 4 (CTLA-4), programmed death 1 (PD-1), T-cell immunoglobulin and mucin domain-containing protein 3 (TIM-3), and lymphocyte activation gene-3 (LAG-3). In addition, the metabolic enzyme indoleamine 2,3-dioxygenase (IDO), which catalyzes the rate-limiting step of the oxidative catabolism of the amino acid tryptophan, regulates T-cell activation. By depleting tryptophan and generating the toxic metabolite kynurenine, IDO can inhibit T-cell proliferation and trigger cell cycle arrest and apoptosis. Kynurenine can also induce naive CD4 T cells into Tregs [120–123].
Activation of immune checkpoint pathways in the TME, however, limits the antitumor immune response. TILs upregulating the expression of checkpoints are hyporesponsive or functionally exhausted [124]. In patients with ovarian cancer, a significant fraction of antigen-specific CD8 TILs co-express LAG-3 and PD-1 and demonstrate impaired effector function [125]. Additionally, Tregs naturally express checkpoints and employ them to suppress effector T cells [124]. Moreover, 56 % of ovarian tumors have demonstrated IDO expression, which correlated with a reduced number of CD8 TILs and with reduced survival in serous (but not other) ovarian cancer histologies [126, 127]. IDO expression was also found to inhibit NK cell intratumoral accumulation and to promote tumor angiogenesis [128]. Reversing TME-mediated immunosuppression via targeting checkpoints with mAbs or inhibitors, an approach coined “checkpoint blockade,” was found to boost immune responses and is becoming increasingly valuable in the clinic.
Anti-CTLA-4 (Ipilimumab)
CTLA-4 (CD152) is an inhibitory co-receptor and member of the B7-CD28 immunoglobulin superfamily. It competes with the costimulatory receptor CD28 for the ligands (B7 molecules) on APCs, leading to downregulation of T-cell activation. Ipilimumab (Yervoy®, BMS) is an FDA-approved human IgG1 mAb that blocks the CTLA-4/B7 interaction to restore CD4 and CD8 effector T-cell activation and can also deplete tumor-infiltrating Tregs [129, 130]. Ipilimumab represents the first standard-of-care immune checkpoint inhibitor, and the majority of clinical experience is derived from studies in patients with melanoma. In a pilot study in two patients with advanced ovarian cancer previously vaccinated with GM-CSF-modified irradiated autologous tumor cells (GVAX), a single dose of ipilimumab triggered a decrease or stabilization of CA-125 levels for several months [131]. In a subsequent study in additional nine patients, three patients had SD, and the extent of therapy-induced tumor necrosis correlated with the intratumoral CD8 T-cell/Treg ratio [132]. An ongoing phase II trial is studying ipilimumab as monotherapy in patients with recurrent platinum-sensitive ovarian cancer (NCT01611558). The primary drawback of ipilimumab is the high frequency of immune-related adverse events (irAEs) like colitis or hypophysitis: approximately 25 % of patients experience an irAE, requiring aggressive management [133].
Anti-PD-1 (Nivolumab and Pembrolizumab) and Anti-PD-L1 (Several mAbs)
PD-1 is another co-inhibitory receptor member of the CD28/B7 immunoglobulin superfamily that binds to its ligands PD-L1 and PD-L2 (mainly expressed by epithelial cells, DCs, and macrophages) to down-modulate the immune response [124]. In addition to the high expression of PD-1 by TILs in ovarian tumors [10, 125], PD-L1 was also found to be highly expressed by ovarian tumor cells and is negatively correlated with CD8 TIL counts and with survival [11]. PD-L1 tumor expression has also been implicated in promoting peritoneal dissemination of ovarian cancer [12]. The blockade of the PD-1 inhibitory pathway is being clinically explored using mAbs targeting either the receptor or its ligands and has so far proven less immunotoxic than ipilimumab.
Nivolumab (Opdivo®, BMS) is an FDA-approved human IgG4 anti-PD-1 mAb that is being investigated in ovarian cancer. In a phase I trial in 15 patients with advanced platinum-resistant ovarian cancer (regardless of PD-L1 expression), nivolumab was well tolerated and led to 3 PR and 4 SD, with an ORR of 17 % [134]. In a recent update, 2 patients with CR survived without disease progression for 17 and 14 months each [135]. Another anti-PD-1 mAb is pembrolizumab (Keytruda®, Merck), an FDA-approved humanized IgG4 mAb. In a recent interim analysis of a phase Ib trial in 26 patients with heavily treated PD-L1+ advanced ovarian cancer, pembrolizumab was well tolerated and achieved 1 CR, 2 PR, and 6 SD with a durable ORR of 11.5 % [136].
Several mAbs that block PD-L1 are being investigated in numerous clinical trials that include ovarian cancer patients. Examples include avelumab (MSB0010718C, human IgG1, Merck Serono), BMS-936559 (human IgG4, BMS-ONO), MPDL3280A (human IgG1, Roche/Genetech), and durvalumab (MEDI4736, human IgG1, MedImmune). In an ongoing phase Ib trial in 75 patients with platinum-resistant or chemotherapy-refractory ovarian cancer (regardless of PD-L1 expression), avelumab demonstrated an acceptable safety profile and had an ORR of 10.7 % in 67 evaluable patients [137]. In a phase I trial involving 17 patients with ovarian cancer, BMS-936559 demonstrated safety and led to 1 PR and 3 SD lasting at least 24 weeks [138].
IDO Inhibitors (Indoximod, GDC-0919, and Epacadostat)
Indoximod (D-1-methyl-tryptophan (D-1-MT), NewLink Genetics) is the first small-molecule IDO inhibitor to enter clinical trials. Preclinical studies in murine models of ovarian cancer have shown that IDO inhibition with the racemic compound 1-MT is synergistic with chemotherapy and that the D (but not the L) racemer is responsible for the majority of antitumor activity [139, 140]. Another IDO inhibitor is the second-generation GDC-0919 (formerly NLG919, NewLink Genetics/Genentech) which specifically inhibits IDO1. Both inhibitors are currently being examined in several clinical trials for solid tumors. A third is the IDO1 inhibitor epacadostat (INCB024360, Incyte Corporation) that is in several clinical trials for ovarian cancer either as monotherapy (NCT01685255 and NCT02042430) or in combination with peptide vaccines (NCT02166905 and NCT02575807) or checkpoint blockade (NCT02178722 and NCT02327078). In a phase I trial in patients with advanced malignancies including ovarian cancer, 90 % inhibition of IDO activity was achieved [141].
Combination Therapies
Combination immunotherapies can synergize to enhance clinical responses by enhancing different stages of the antitumor immune response (antigen uptake and presentation, T-cell activation, and T-cell response maintenance) and modifying various aspects in the TME (angiogenesis and immunosuppression). Combining checkpoint blockade with other immunotherapeutic strategies that have shown limited efficacy as monotherapies, such as vaccination, is an area of intensive research. For example, a phase I trial in six patients with recurrent ovarian cancer examined a DC-based autologous whole tumor lysate vaccine in combination with Treg-depleting metronomic cyclophosphamide, the antiangiogenic mAb bevacizumab, and ACT (vaccine-primed ex vivo CD3/CD28-costimulated peripheral blood autologous T cells). Antitumor immune responses (in the form of increased tumor-reactive T cells and reduced Tregs) and clinical benefit were observed in four patients, including 1 CR, 1 PR, and 2 SD [142]. Checkpoint inhibitors in combination are also being clinically tested. For example, an ongoing phase I trial in patients with advanced solid tumors (including ovarian cancer) is evaluating the combination of tremelimumab (a humanized IgG2 anti-CTLA-4 mAb) with durvalumab (anti-PD-L1) (NCT01975831) [143]. Identification of the optimal immunotherapy combination will require evaluation of not only synergistic mechanisms of action and ideal sequence of dosing but also overlapping toxicities in order to maximize clinical benefit.
Conclusions
Recent advances and rational immunotherapeutic combinations hold immense potential to improve outcomes for patients with ovarian cancer. Nonetheless, a number of challenges remain that are being critically addressed. Immunotherapies are typically administered in conjunction with or post chemotherapy. Several chemotherapeutic drugs utilized in ovarian cancer exert immunostimulatory effects. Examples include platinum compounds (which reduce PD-L2 expression on DCs), trabectedin (which inhibits monocyte differentiation into TAMs), and decitabine (which triggers a type I IFN response) [144–146]. Integrating immunotherapy with chemotherapy requires careful consideration of drugs, dosing, and schedule in order to neutralize the unwarranted immunosuppressive effects and maximize the immunostimulatory effects of chemotherapy [147]. A second challenge is to better characterize the TME not only to identify the rare somatic mutations that are immunogenic but also to understand host-tumor interactions. Like other cancers, ovarian cancer is heterogenic, and recent gene expression profiling studies have recognized several distinct molecular subtypes with markedly different prognoses [148]. Identifying dominant immunosuppressive pathways as well as collateral pathways can improve response rates in immunotherapy by improving the design of combination treatment regimens [149, 150]. Lastly, identification of biomarkers that predict response to immunotherapies will allow patients to be optimally matched with therapies that are expected to deliver the maximum benefit while minimizing unnecessary toxicity.
References
1.
Zhang L, Conejo-Garcia JR, Katsaros D, Gimotty PA, Massobrio M, Regnani G, et al. Intratumoral T cells, recurrence, and survival in epithelial ovarian cancer. N Engl J Med. 2003;348(3):203–13. doi:348/3/203 [pii].PubMed
2.
Sato E, Olson SH, Ahn J, Bundy B, Nishikawa H, Qian F, et al. Intraepithelial CD8+ tumor-infiltrating lymphocytes and a high CD8+/regulatory T cell ratio are associated with favorable prognosis in ovarian cancer. Proc Natl Acad Sci U S A. 2005;102(51):18538–43. doi:10.1073/pnas.0509182102.PubMedPubMedCentral
3.
Tomsová M, Melichar B, Sedláková I, Steiner I. Prognostic significance of CD3+ tumor-infiltrating lymphocytes in ovarian carcinoma. Gynecol Oncol. 2008;108(2):415–20. doi:10.1016/j.ygyno.2007.10.016.PubMed
4.
Hwang WT, Adams SF, Tahirovic E, Hagemann IS, Coukos G. Prognostic significance of tumor-infiltrating T cells in ovarian cancer: a meta-analysis. Gynecol Oncol. 2012;124(2):192–8. doi:10.1016/j.ygyno.2011.09.039.PubMed
5.
Curiel TJ, Coukos G, Zou L, Alvarez X, Cheng P, Mottram P, et al. Specific recruitment of regulatory T cells in ovarian carcinoma fosters immune privilege and predicts reduced survival. Nat Med. 2004;10(9):942–9. doi:10.1038/nm1093.PubMed
6.
Chu CS, Kim SH, June CH, Coukos G. Immunotherapy opportunities in ovarian cancer. Expert Rev Anticancer Ther. 2008;8(2):243–57. doi:10.1586/14737140.8.2.243.PubMed
7.
Walters CL, Arend RC, Armstrong DK, Naumann RW, Alvarez RD. Folate and folate receptor alpha antagonists mechanism of action in ovarian cancer. Gynecol Oncol. 2013;131(2):493–8. doi:10.1016/j.ygyno.2013.07.080.PubMed
8.
Yakirevich E, Sabo E, Lavie O, Mazareb S, Spagnoli GC, Resnick MB. Expression of the MAGE-A4 and NY-ESO-1 cancer-testis antigens in serous ovarian neoplasms. Clini Cancer Res Off J Am Asso. 2003;9(17):6453–60.
9.
Lin CK, Chao TK, Yu CP, Yu MH, Jin JS. The expression of six biomarkers in the four most common ovarian cancers: correlation with clinicopathological parameters. APMIS Acta Pathologica Micro Immunolo Scandinavica. 2009;117(3):162–75. doi:10.1111/j.1600-0463.2008.00003.x.
10.
Webb JR, Milne K, Nelson BH. PD-1 and CD103 are widely coexpressed on prognostically favorable intraepithelial CD8 T cells in human ovarian cancer. Cancer Immunol Res. 2015;3(8):926–35. doi:10.1158/2326-6066.CIR-14-0239.PubMed
11.
Hamanishi J, Mandai M, Iwasaki M, Okazaki T, Tanaka Y, Yamaguchi K, et al. Programmed cell death 1 ligand 1 and tumor-infiltrating CD8+ T lymphocytes are prognostic factors of human ovarian cancer. Proc Natl Acad Sci U S A. 2007;104(9):3360–5. doi:10.1073/pnas.0611533104.PubMedPubMedCentral
12.
Abiko K, Mandai M, Hamanishi J, Yoshioka Y, Matsumura N, Baba T, et al. PD-L1 on tumor cells is induced in ascites and promotes peritoneal dissemination of ovarian cancer through CTL dysfunction. Clini Cancer Res Off J Am Asso. 2013;19(6):1363–74. doi:10.1158/1078-0432.CCR-12-2199.
13.
Makkouk A, Weiner GJ. Cancer immunotherapy and breaking immune tolerance: new approaches to an old challenge. Cancer Res. 2015;75(1):5–10. doi:10.1158/0008-5472.CAN-14-2538.PubMed
14.
Martinez Forero I, Okada H, Topalian SL, Gajewski TF, Korman AJ, Melero I. Workshop on immunotherapy combinations. Society for Immunotherapy of Cancer annual meeting Bethesda, November 3, 2011. J Transl Med. 2012;10:108. doi:10.1186/1479-5876-10-108.PubMed
15.
Geskin LJ. Monoclonal antibodies. Dermatol Clin. 2015;33(4):777–86. doi:10.1016/j.det.2015.05.015.PubMed
16.
Vanneman M, Dranoff G. Combining immunotherapy and targeted therapies in cancer treatment. Nat Rev Cancer. 2012;12(4):237–51. doi:10.1038/nrc3237;10.1038/nrc3237.PubMedPubMedCentral
17.
Weiner GJ. Building better monoclonal antibody-based therapeutics. Nat Rev Cancer. 2015;15(6):361–70. doi:10.1038/nrc3930.PubMedPubMedCentral
18.
Siwak DR, Carey M, Hennessy BT, Nguyen CT, McGahren Murray MJ, Nolden L, et al. Targeting the epidermal growth factor receptor in epithelial ovarian cancer: current knowledge and future challenges. J Oncol. 2010;2010:568938. doi:10.1155/2010/568938.PubMed
19.
Lu Y, Li X, Liang K, Luwor R, Siddik ZH, Mills GB, et al. Epidermal growth factor receptor (EGFR) ubiquitination as a mechanism of acquired resistance escaping treatment by the anti-EGFR monoclonal antibody cetuximab. Cancer Res. 2007;67(17):8240–7. doi:10.1158/0008-5472.CAN-07-0589.PubMed
20.
Patel D, Lahiji A, Patel S, Franklin M, Jimenez X, Hicklin DJ, et al. Monoclonal antibody cetuximab binds to and down-regulates constitutively activated epidermal growth factor receptor vIII on the cell surface. Anticancer Res. 2007;27(5A):3355–66.PubMed
21.
Schilder RJ, Pathak HB, Lokshin AE, Holloway RW, Alvarez RD, Aghajanian C, et al. Phase II trial of single agent cetuximab in patients with persistent or recurrent epithelial ovarian or primary peritoneal carcinoma with the potential for dose escalation to rash. Gynecol Oncol. 2009;113(1):21–7. doi:10.1016/j.ygyno.2008.12.003.PubMedPubMedCentral
22.
Secord AA, Blessing JA, Armstrong DK, Rodgers WH, Miner Z, Barnes MN, et al. Phase II trial of cetuximab and carboplatin in relapsed platinum-sensitive ovarian cancer and evaluation of epidermal growth factor receptor expression: a Gynecologic Oncology Group study. Gynecol Oncol. 2008;108(3):493–9. doi:10.1016/j.ygyno.2007.11.029.PubMedPubMedCentral
23.
Steffensen KD, Waldstrom M, Pallisgard N, Lund B, Bergfeldt K, Wihl J, et al. Panitumumab and pegylated liposomal doxorubicin in platinum-resistant epithelial ovarian cancer with KRAS wild-type: the PaLiDo study, a phase II nonrandomized multicenter study. Int J Gynecolo Cancer Off J. 2013;23(1):73–80. doi:10.1097/IGC.0b013e3182775fae.
24.
Gordon AN, Fleagle JT, Guthrie D, Parkin DE, Gore ME, Lacave AJ. Recurrent epithelial ovarian carcinoma: a randomized phase III study of pegylated liposomal doxorubicin versus topotecan. J Clin Oncol. 2001;19(14):3312–22.PubMed
25.
Tang Z, Qian M, Ho M. The role of mesothelin in tumor progression and targeted therapy. Anticancer Agents Med Chem. 2013;13(2):276–80.PubMedPubMedCentral
26.
Felder M, Kapur A, Gonzalez-Bosquet J, Horibata S, Heintz J, Albrecht R, et al. MUC16 (CA125): tumor biomarker to cancer therapy, a work in progress. Mol Cancer. 2014;13:129. doi:10.1186/1476-4598-13-129.PubMedPubMedCentral
27.
Hassan R, Cohen SJ, Phillips M, Pastan I, Sharon E, Kelly RJ, et al. Phase I clinical trial of the chimeric anti-mesothelin monoclonal antibody MORAb-009 in patients with mesothelin-expressing cancers. Clin Cancer Res. 2010;16(24):6132–8. doi:10.1158/1078-0432.CCR-10-2275.PubMedPubMedCentral
28.
Sabbatini P, Harter P, Scambia G, Sehouli J, Meier W, Wimberger P, et al. Abagovomab as maintenance therapy in patients with epithelial ovarian cancer: a phase III trial of the AGO OVAR, COGI, GINECO, and GEICO–the MIMOSA study. J Clin Oncol. 2013;31(12):1554–61. doi:10.1200/JCO.2012.46.4057.PubMed
29.
Berek JS, Taylor PT, Gordon A, Cunningham MJ, Finkler N, Orr J, et al. Randomized, placebo-controlled study of oregovomab for consolidation of clinical remission in patients with advanced ovarian cancer. J Clin Oncol. 2004;22(17):3507–16. doi:10.1200/JCO.2004.09.016.PubMed
30.
Berek J, Taylor P, McGuire W, Smith LM, Schultes B, Nicodemus CF. Oregovomab maintenance monoimmunotherapy does not improve outcomes in advanced ovarian cancer. J Clin Oncol. 2009;27(3):418–25. doi:10.1200/JCO.2008.17.8400.PubMed
31.
Bagnoli M, Canevari S, Figini M, Mezzanzanica D, Raspagliesi F, Tomassetti A, et al. A step further in understanding the biology of the folate receptor in ovarian carcinoma. Gynecol Oncol. 2003;88(1 Pt 2):S140–4.PubMed
32.
Lutz RJ. Targeting the folate receptor for the treatment of ovarian cancer. Transl Cancer Res. 2015;4(1):118–26. doi: 10.3978/j.issr2218-676X.2015.01.04.
33.
Armstrong DK, White AJ, Weil SC, Phillips M, Coleman RL. Farletuzumab (a monoclonal antibody against folate receptor alpha) in relapsed platinum-sensitive ovarian cancer. Gynecol Oncol. 2013;129(3):452–8. doi:10.1016/j.ygyno.2013.03.002.PubMed
34.
Drerup JM, Liu Y, Padron AS, Murthy K, Hurez V, Zhang B, et al. Immunotherapy for ovarian cancer. Curr Treat Options Oncol. 2015;16(1):317. doi:10.1007/s11864-014-0317-1.PubMedPubMedCentral
35.
Ferrara N, Gerber HP, LeCouter J. The biology of VEGF and its receptors. Nat Med. 2003;9(6):669–76. doi:nm0603-669 [pii].PubMed
36.
Hata K, Watanabe Y, Nakai H, Hata T, Hoshiai H. Expression of the vascular endothelial growth factor (VEGF) gene in epithelial ovarian cancer: an approach to anti-VEGF therapy. Anticancer Res. 2011;31(2):731–7. doi:31/2/731 [pii].PubMed
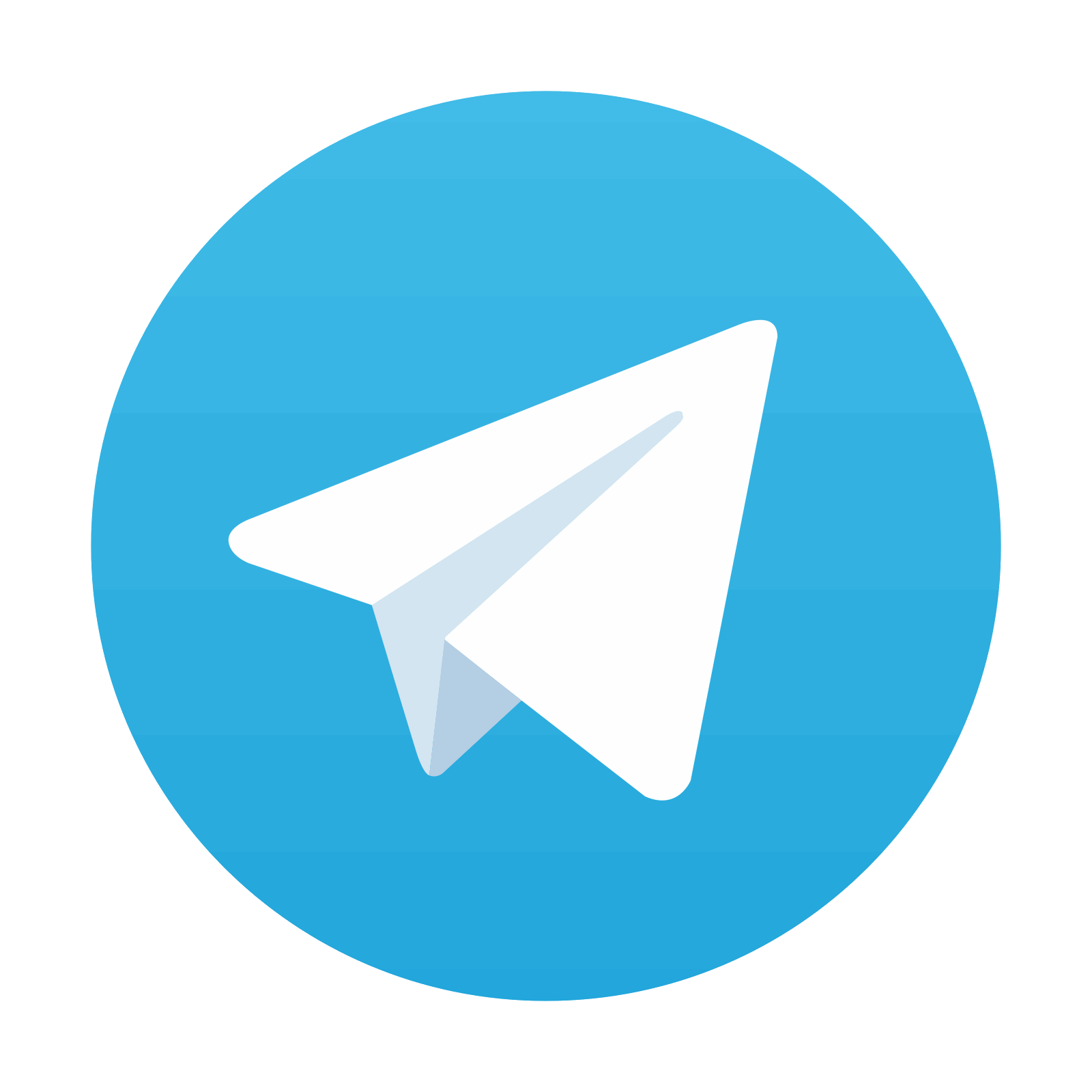
Stay updated, free articles. Join our Telegram channel

Full access? Get Clinical Tree
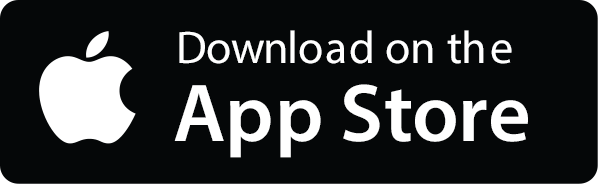
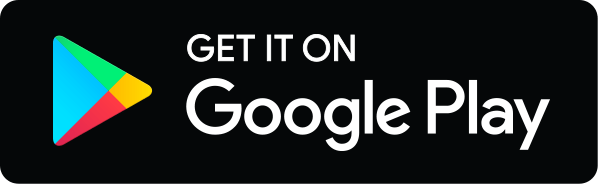