Abbreviations
- ACTH Adrenocorticotropin hormone
- AFP Alph-afetoprotein
- AMH Anti-Müllerian hormone
- BMI Body mass index
- BV Bacterial vaginosis
- CRH Corticotropin-releasing hormone
- DHEA Dehydroepiandrosterone
- EGF Epidermal growth factor
- fFN Fetal fibronectin
- FGF Fibroblast growth factor
- GDM Gestational diabetes mellitus
- GH Growth hormone
- GnRH Gonadotropin-releasing hormone
- GTN Gestational trophoblastic neoplasia
- hCG human chorionic gonadotropin
- HELLP syndrome Hemolysis, elevated liver enzymes, and low platelets
- hPL Human placental lactogen
- IGF Insulin-like growth factor
- LH luteinizing hormone
- NSAID Nonsteroidal anti-inflammatory drug
- PDGF Platelet-derived growth factor
- PlGF Placental growth factor
- PRL Prolactin
- PROM Premature rupture of membranes
- PTB Preterm birth
- sEng Soluble endoglin
- sFlt-1 Soluble fms-like tyrosine kinase 1
- SHBG Sex hormone–binding globulin
- TRH Thyrotropin-releasing hormone
- TSH Thyroid-stimulating hormone
- VEGF Vascular endothelial growth factor
The Endocrinology of Pregnancy: Introduction
Throughout pregnancy, the fetal-placental unit secretes protein and steroid hormones that alter the function of every endocrine gland in the mother’s body. Both clinically and in the laboratory, pregnancy can mimic hyperthyroidism, Cushing disease, pituitary adenoma, diabetes mellitus, polycystic ovary syndrome, and more.
The endocrine changes associated with pregnancy are adaptive, allowing the mother to nurture the developing fetus. Although maternal reserves are usually adequate, in cases of gestational diabetes or hypertensive disease of pregnancy, a woman may develop overt signs of disease as a direct result of pregnancy.
Aside from creating a satisfactory nutritive environment for fetal development, the placenta serves as an endocrine, respiratory, alimentary, and excretory organ. Measurements of fetal-placental products in the maternal serum provide one means of assessing fetal well being. This chapter will consider the changes in maternal endocrine function in pregnancy and during parturition as well as fetal endocrine development. The chapter concludes with a discussion of some endocrine disorders complicating pregnancy.
Conception and Implantation
In fertile women, ovulation occurs approximately 12 to 16 days after the onset of the previous menses. The ovum must be fertilized within 24 to 48 hours if conception is to result. For about 48 hours around ovulation, cervical mucus is copious, nonviscous, slightly alkaline, and forms a gel matrix that acts as a filter and conduit for sperm. Sperm begin appearing in the outer third of the fallopian tube (the ampulla) 5 to 10 minutes after coitus and continue to migrate to this location from the cervix for about 24 to 48 hours. Of the 200 × 106 sperm that are deposited in the vaginal fornices, only approximately 200 reach the distal tube. Fertilization normally occurs in the ampulla.
Embryonic invasion of the uterus occurs during a specific window of implantation 8 to 10 days after ovulation and fertilization, when the conceptus is a blastocyst. Vitronectin, an alpha-v-beta-3 integrin receptor ligand, serves as one of several links between the maternal and embryonic epithelia. The two layers of placental epithelial cells, cytotrophoblasts and syncytiotrophoblasts, develop after the blastocyst invades the endometrium (Figure 16–1). Columns of invading cytotrophoblasts anchor the placenta to the endometrium. The differentiated syncytiotrophoblast, derived from fusion of cytotrophoblasts, is in direct contact with the maternal circulation. The syncytiotrophoblast is the major source of hormone production, containing the cellular machinery needed for synthesis and secretion of both steroid and polypeptide hormones.
Figure 16–1
Microanatomy of the human placental bed. Oxygen, nutrients, and waste products are exchanged between the fetus and the mother across this interface. In normal placental development (upper panel), invasive cytotrophoblasts of fetal origin invade the maternal spiral arteries, displacing maternal endothelial cells and transforming the vessels from small-caliber resistance vessels to high-caliber capacitance vessels capable of placental perfusion adequate to sustain fetal growth. In preeclampsia and some cases of fetal growth restriction, cytotrophoblasts fail to adopt an invasive endothelial phenotype. Instead, invasion of the spiral arteries is shallow and they remain small-caliber resistance vessels (lower panel). This can lead to placental ischemia.
(Adapted, with permission, from Karumanchi SA, et al. Preeclampsia: a renal perspective. Kidney Int. 2005;67:2101.)
In most spontaneously conceived pregnancies, the dates of ovulation and implantation are not known. Weeks of gestation (gestational age) are by convention calculated from the first day of the last menstrual period. Within 24 hours after implantation, or at about 3 weeks of gestation, human chorionic gonadotropin (hCG), produced by syncytiotrophoblasts (see Figure 16–1), is detectable in maternal serum. Under the influence of increasing hCG production, the corpus luteum secretes progesterone and estradiol in increasing quantities.
The hormones produced by the corpus luteum include progesterone, 17-hydroxyprogesterone, relaxin, and estradiol. The indispensability of the corpus luteum in early pregnancy has been demonstrated by ablation studies, in which luteectomy or oophorectomy before 42 days of gestation results in precipitous decreases in levels of serum progesterone and estradiol, followed by abortion. Exogenous progesterone will prevent abortion, proving that progesterone alone is required for maintenance of early pregnancy. After about the seventh gestational week, the corpus luteum can be removed without subsequent abortion owing to compensatory progesterone production by the placenta.
Because the placenta does not express the 17α-hydroxylase enzyme (P450C17), it cannot produce appreciable amounts of 17-hydroxyprogesterone; thus, this steroid provides a marker of corpus luteum function. As shown in Figure 16–2, the serum concentrations of estrogens and total progesterone exhibit a steady increase, but the concentration of 17-hydroxyprogesterone rises and then declines to low levels that persist for the duration of the pregnancy. Another marker of corpus luteum function is the polypeptide hormone relaxin, a protein with a molecular mass of about 6000. Pharmacologically, relaxin ripens the cervix, softens the pubic symphysis, promotes decidual angiogenesis, and acts synergistically with progesterone to inhibit uterine contractions.
Fetal-Placental-Decidual Unit
The function of the placenta is to establish effective communication between the mother and the developing fetus while maintaining the immune and genetic integrity of both individuals. Initially, the placenta functions autonomously. By the end of the first trimester, however, the fetal endocrine system is sufficiently developed to complement placental function and to provide some hormone precursors to the placenta. From this time, it is useful to consider the endocrine conceptus as the fetal-placental unit.
The decidua is the endometrium of pregnancy. Decidual cells are capable of synthesizing a variety of polypeptide hormones, including relaxin, prolactin (PRL), and a variety of paracrine factors, in particular insulin-like growth factor (IGF)-binding protein 1. The precise function of the decidua as an endocrine organ has not been established, but its role as a source of prostaglandins during labor is certain (see “Endocrine Control of Parturition,” later).
Polypeptide Hormones
The first marker of trophoblast differenation and the first measurable product of the placenta is hCG, a glycoprotein consisting of 237 amino acids. It is similar in structure to the pituitary glycoprotein hormones in that it consists of two chains: a common alpha chain and a specific beta chain, which determines receptor interaction and ultimate biologic specificity. The alpha chain is identical in sequence to the alpha chains of thyroid-stimulating hormone (TSH), follicle-stimulating hormone (FSH), and luteinizing hormone (LH). The beta chain has 67% sequence homology with LH and an additional 30 amino acids not found in LH beta.
In early pregnancy (up to 6 weeks), the concentration of hCG doubles every 1.7 to 2 days, and serial measurements provide a sensitive index of early trophoblast function and viability. Maternal plasma hCG peaks during the 10th gestational week and then declines gradually in the third trimester. Peak concentrations correlate temporally with the establishment of maternal blood flow in the intervillous space (see Figure 16–1, upper panel).
The long plasma half-life of hCG (24 hours) allows the tiny mass of cells comprising the blastocyst to produce sufficient hormone to be detected in the peripheral circulation within 24 hours of implantation. Thus, pregnancy can be diagnosed several days before symptoms occur or a menstrual period has been missed. Antibodies to the unique beta-carboxyl terminal segment of hCG do not cross-react significantly with any of the pituitary glycoproteins.
hCG is also produced in gestational trophoblastic neoplasia (GTN) by hydatidiform mole and choriocarcinoma, and the concentration of hCG-beta is used as a tumor marker for diagnosis and for monitoring the success of chemotherapy in these disorders. Women with very high hCG levels due to GTN may become clinically hyperthyroid due to the action of hCG on TSH receptors; they can revert to euthyroidism as hCG is reduced during chemotherapy.
A second placental polypeptide hormone, this one with homology to pituitary growth hormone (GH), is human placental lactogen (hPL). hPL is produced by early trophoblasts, but detectable serum concentrations are not reached until 4 to 5 gestational weeks (see Figure 16–2). hPL is a protein of 190 amino acids whose primary, secondary, and tertiary structures are similar to those of GH and PRL. hPL is diabetogenic and lactogenic, but it has minimal growth-promoting activity as measured by standard GH bioassays.
The physiologic role of hPL during pregnancy remains controversial, and normal pregnancy without detectable hPL production has been reported. Although not clearly shown to be a mammotropic agent, hPL contributes to altered maternal glucose metabolism and mobilization of free fatty acids; causes a hyperinsulinemic response to glucose loads; appears to directly stimulate pancreatic islet insulin secretion; and contributes to the peripheral insulin resistance characteristic of pregnancy. Along with prolonged fasting and insulin-induced hypoglycemia, pre-beta-HDL and apoprotein A-I are two factors that stimulate release of hPL. hPL production is roughly proportionate to placental mass. Actual production rates may reach as much as 1 to 1.5 g/d at term.
Serum hPL concentrations were used historically as a clinical indicator of the health of the placenta, but the range of normal values was wide, and serial determinations were necessary; it is therefore no longer used clinically.
Other chorionic peptides have been identified, but their functions remain poorly defined. Activin, inhibin, corticotropin-releasing hormone, and multiple peptide growth factors, including fibroblast growth factor (FGF), epidermal growth factor (EGF), platelet-derived growth factor (PDGF), and the IGFs all have been isolated from placental tissue. Placental growth factor (PlGF) and the related vascular endothelial growth factor (VEGF) are suggested to play a role in placental angiogenesis, preeclampsia, and fetal growth.
Steroid Hormones
In contrast to the impressive synthetic capability exhibited in the production of placental proteins, the placenta does not have the ability to synthesize steroids de novo. However, the trophoblasts have remarkable capacity to efficiently interconvert steroids derived from maternal or fetal precursors. This activity is demonstrable even in the early blastocyst, and by the seventh gestational week, when the corpus luteum undergoes involution, the placenta becomes the dominant source of steroid hormones.
The placenta relies on maternal cholesterol as its substrate for progesterone production. Fetal death has no immediate influence on progesterone production, suggesting that the fetus is a negligible source of substrate. Enzymes in the placenta cleave the cholesterol side chain, yielding pregnenolone, which in turn is isomerized to progesterone; 250 to 350 mg of progesterone are produced daily by the third-trimester placenta, and most enters the maternal circulation (see Figure 16–2). Whereas exogenous hCG stimulates progesterone production in early pregnancy, hypophysectomy, adrenalectomy, or oophorectomy have no effect on progesterone levels after the luteo-placental shift at 7 to 9 weeks’ gestation. Likewise, the administration of adrenocorticotropin (ACTH) or cortisol does not influence placental progesterone secretion.
Progesterone is necessary for establishment and maintenance of pregnancy. Insufficient production of progesterone may contribute to failure of implantation, recurrent pregnancy loss, and preterm delivery. Progesterone, along with relaxin and nitric oxide, maintains uterine quiescence during pregnancy. Clinical trials have indicated that the administration of 17α-hydroxyprogesterone caproate can reliably prevent preterm delivery in high-risk pregnancies. Progesterone also inhibits T cell–mediated allograft rejection. Thus, high local concentrations of progesterone may contribute to immunologic tolerance by the uterus of invading trophoblast tissue from the semiallogeneic fetus.
Estrogen production by the placenta also depends on circulating precursors, but in this case both fetal and maternal steroids are important sources. Most of the placental estrogens are derived from fetal androgens, primarily dehydroepiandrosterone (DHEA) sulfate. Fetal DHEA sulfate, produced mainly by the fetal adrenal, is converted by placental sulfatase to free DHEA and then, through enzymatic pathways common to steroid-producing tissues, to androstenedione and testosterone. These androgens are finally aromatized by the placenta to estrone and estradiol, respectively. 17α-Hydroxysteroid dehydrogenase type II prevents fetal exposure to potent estrogens by catalyzing the conversion of estradiol to less potent estrone.
Most fetal DHEA sulfate is metabolized to produce a third estrogen: estriol. Estriol is a weak estrogen with 1/10 the potency of estrone and 1/100 the potency of estradiol. Serum estrone and estradiol concentrations are increased during pregnancy about 50-fold over their maximal prepregnancy values, but estriol increases approximately 1000-fold. The substrate for the reaction is 16α-hydroxy-DHEA sulfate produced in the fetal adrenal and liver, not in maternal or placental tissues. The final steps of desulfation and aromatization to estriol occur in the placenta. Maternal serum or urinary estriol measurements, unlike measurements of progesterone or hPL, reflect fetal as well as placental function. Rising serum or urinary estriol concentrations were once used as biochemical indicators of fetal well-being (see Figure 16–2). Decreased estriol production can result from congenital derangements or iatrogenic intervention. Maternal estriol remains low in pregnancies with placental sulfatase deficiency and in cases of fetal anencephaly. In the first case, DHEA sulfate cannot be hydrolyzed; in the second, little fetal DHEA is produced because fetal adrenal growth stimulation by ACTH is lacking. Maternal administration of glucocorticoids also inhibits fetal ACTH and lowers maternal estriol. Administration of DHEA to the mother during a healthy pregnancy increases estriol production. Placental corticotropin-releasing hormone (CRH) also may be an important regulator of fetal adrenal DHEA sulfate secretion.
Modern methods of screening for fetal chromosomal aneuploidy, particularly trisomy 21 (Down syndrome), utilize circulating biochemical markers. Screening by maternal age alone (>35 years) led to the prenatal identification of only about 25% of aneuploid fetuses. As an aneuploid chromosome complement affects both fetal and placental tissues, their protein and steroid products have been evaluated. A combination of alpha-fetoprotein (AFP), hCG, inhibin-A and unconjugated estriol concentrations, measured in maternal serum between 15 and 18 weeks’ gestation, can be used to identify fetal Down syndrome and trisomy 18 with a detection rate of 80% over all age groups.
Maternal Adaptation to Pregnancy
As a successful parasite, the fetal-placental unit manipulates the maternal host for its own gain but normally avoids imposing excessive stress that would jeopardize the pregnancy. The prodigious production of polypeptide and steroid hormones by the fetal–placental unit directly or indirectly results in physiologic adaptations of virtually every maternal organ system. These alterations are summarized in Figure 16–3. Most of the commonly measured maternal endocrine function tests are radically changed. In some cases, true physiologic alteration has occurred; in others, the changes are due to increased production of hormone-binding proteins by the liver or to decreased serum levels of albumin due to the mild dilutional anemia of pregnancy. In addition, some endocrine changes are mediated by altered clearance rates due to increased glomerular filtration, decreased hepatic excretion, or metabolic clearance of steroid and protein hormones by the placenta. The changes in endocrine function tests are summarized in Table 16–1. Failure to recognize normal pregnancy-induced alterations in endocrine function tests can lead to unnecessary diagnostic tests and therapy that may be seriously detrimental to mother and fetus.
Test | Result | |
---|---|---|
Pituitary | ||
FSH, LH | GnRH stimulation | Unresponsive from third gestational week until puerperium |
GH | Insulin tolerance test | Response increases during the first half of pregnancy and then is blunted until the puerperium |
Arginine stimulation | Hyperstimulation during the first and second trimesters, then suppression | |
TSH | TRH stimulation | Response unchanged |
Pancreas | ||
Insulin | Glucose tolerance | Peak glucose increases, and glucose concentration remains elevated longer |
Glucose challenge | Insulin concentration increases to higher peak levels | |
Arginine infusion | Insulin response is blunted in mid to late pregnancy | |
Adrenal | ||
Cortisol | ACTH infusion | Exaggerated cortisol and aldosterone responses |
Metyrapone | Diminished response | |
Mineralocorticoids | ACTH infusion | No DOC response |
Dexamethasone suppression | No DOC response |
The mother’s anterior pituitary gland hormones have little influence on pregnancy after implantation has occurred. The gland itself enlarges by about one-third, with the major component of this increase being hyperplasia of the lactotrophs in response to the high plasma estrogens. PRL, the product of the lactotrophs, is the only anterior pituitary hormone that rises progressively during pregnancy and peaks at the time of delivery. In spite of its high serum concentrations, pulsatile release of PRL and food-induced and nocturnal increases persist. Hence, the normal neuroendocrine regulatory mechanisms appear to be intact in the maternal adenohypophysis. In nonlactating women, maternal PRL decreases to pregestational levels within 3 months of delivery. Pituitary ACTH and TSH secretion remain unchanged. Serum FSH and LH fall to the lower limits of detectability and are unresponsive to GnRH stimulation during pregnancy. GH concentrations are not significantly different from nonpregnant levels, but pituitary response to provocative testing is markedly altered. GH response to hypoglycemia and arginine infusion is enhanced in early pregnancy but thereafter becomes depressed. Established pregnancy can continue in the face of hypophysectomy, and in women hypophysectomized prior to pregnancy, induction of ovulation and normal pregnancy can be achieved with appropriate gonadotropin replacement therapy. In cases of primary pituitary hyperfunction, the fetus is not affected.
The thyroid becomes palpably enlarged during the first trimester, and a subtle bruit may be present. Thyroid iodide clearance and thyroidal 131I uptake, which are clinically contraindicated in pregnancy, have been shown to be increased. These changes are due in large part to the increased renal clearance of iodide, which causes a relative iodine deficiency. Although total serum thyroxine is elevated as a result of estrogen-stimulated increased thyroid hormone–binding globulin (TBG), free thyroxine and triiodothyronine are normal (see Figure 16–2). High circulating concentrations of hCG, particularly asialo-hCG, which has weak TSH-like activity, contribute to the thyrotropic action of the placenta in early pregnancy. In fact, there is significant, though transient, biochemical hyperthyroidism associated with hCG stimulation in early gestation.
The net calcium requirement imposed by fetal skeletal development is estimated to be about 30 g by term. This is met by hyperplasia of the maternal parathyroid glands and elevated serum levels of parathyroid hormone. The maternal serum calcium concentration declines to a nadir at 28 to 32 weeks, largely due to the mild hypoalbuminemia of pregnancy but also to fetal bone formation. Ionized calcium is maintained at normal concentrations throughout pregnancy.
The nutritional demands of the fetus require alteration of maternal metabolic homeostatic control, which results in both structural and functional changes in the maternal pancreas. The size of pancreatic islets increases, and insulin-secreting beta cells undergo hyperplasia. Basal levels of insulin are lower or unchanged in early pregnancy but increase during the second trimester as a result of increased secretion rather than decreased metabolic clearance. Thereafter, pregnancy is a hyperinsulinemic state, with resistance to its peripheral metabolic effects. Pancreatic production of glucagon remains responsive to the usual endocrine stimuli and is suppressed by glucose loading, although the degree of responsiveness has not been well evaluated in pregnancy.
The major roles of insulin and glucagon involve the intracellular transport of nutrients, specifically glucose, amino acids, and fatty acids. Insulin is not transported across the placenta, but rather exerts its effects on transportable metabolites. During pregnancy, peak insulin secretion in response to meals is accelerated, and glucose tolerance curves are characteristically altered. Fasting glucose levels are maintained at low-normal levels. Excess carbohydrate is converted to fat, and fat is readily mobilized during decreased caloric intake.
Total plasma cortisol concentrations increase to three times nonpregnant levels by the third trimester. Most of the changes can be explained by increased estrogens causing higher corticosteroid-binding globulin (CBG) production. The actual production of cortisol by the zona fasciculata also is increased in pregnancy. The net effect of these changes increases plasma-free cortisol, which is approximately doubled by late pregnancy. In spite of cortisol concentrations approaching those found in Cushing syndrome, diurnal variation in plasma cortisol is maintained. The elevated free cortisol concentration probably contributes to the insulin resistance of pregnancy and possibly to the appearance of striae, but most signs of hypercortisolism do not occur in pregnancy. It is suggested that high progesterone levels act to antagonize glucocorticoid effects.
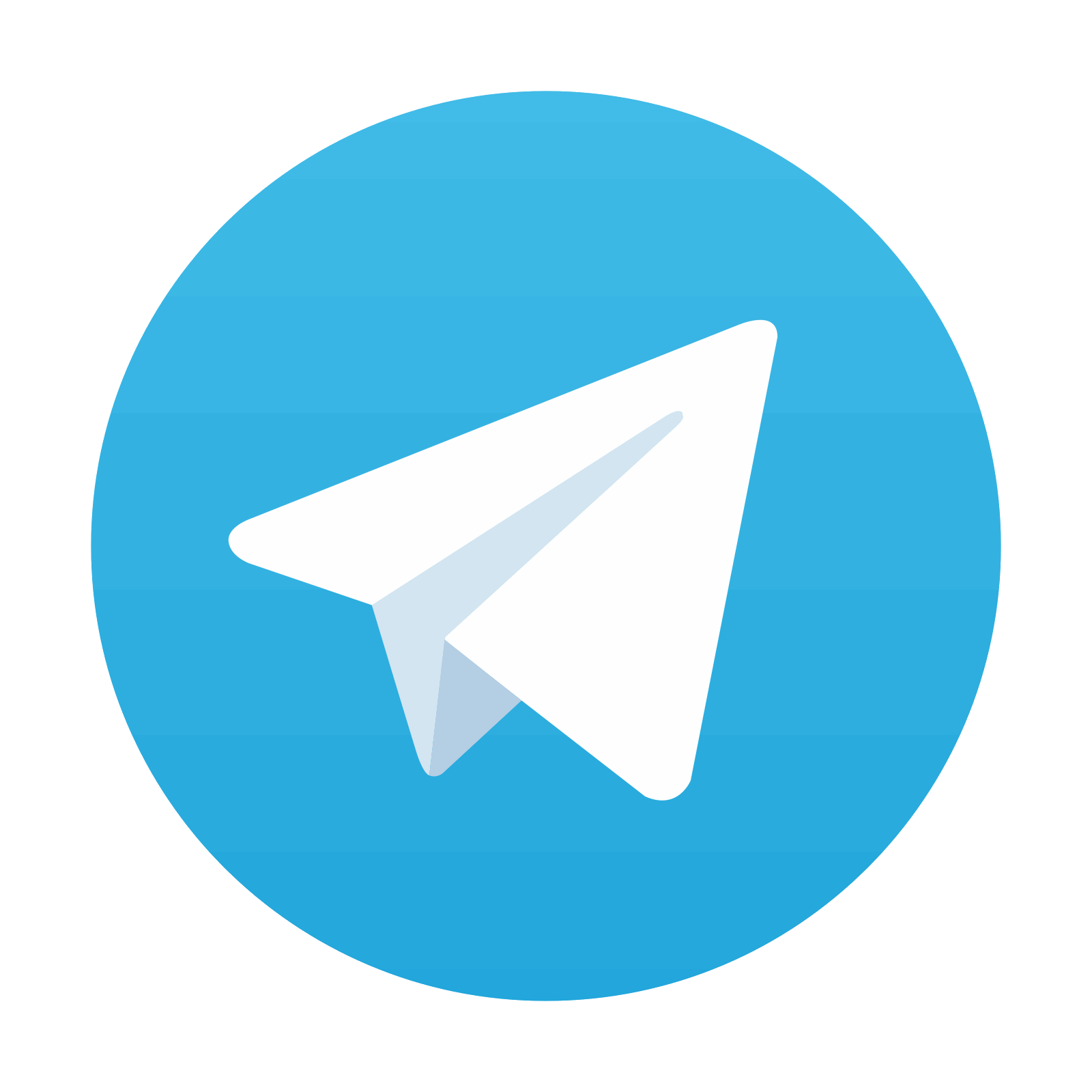
Stay updated, free articles. Join our Telegram channel

Full access? Get Clinical Tree
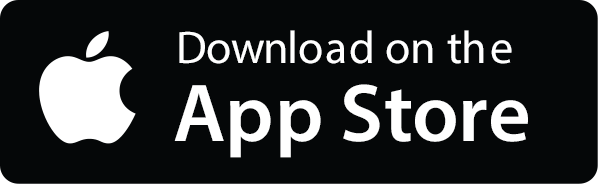
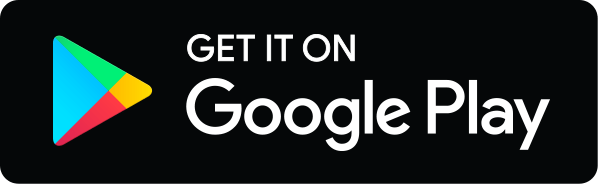