Changes in diet
Hypercaloric (energy dense)
High fat
High saturated fat
Increased portion size
Less physical activity
Less manual/physical work
Increased use of cars
Sleep: less sleep, poor quality
Stress
Drug therapies that promote weight gain
Antipsychotics
Diabetes medication
Contraceptives
Steroids
Less smoking (nicotine promotes appetite suppression)
Maternal—gestational weight gain that favors obesity in offspring
Other putative factors
Plastics (endocrine disruptors)
Pollution (endocrine disruptors)
Less thermogenesis?
Genetics
FTO gene
Genetic syndromes (such as Prader-Willi)
Microbial and viral factors
Gut flora
Viruses associated with obesity
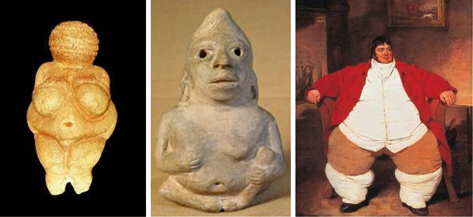
Fig. 1.1
Venus of Willendorf (c. 25,000 BC); Olmec figurine of an obese seated figure (c 400 BC); Daniel Lambert – portrait by Benjamin Marshall (c. 1806)
Obesity is defined by the World Health Organization using the body mass index (BMI): Obese refers to any person with a BMI greater than or equal to 30 [1]. BMI (a person’s weight in kilograms divided by the square of his/her height in meters (kg/m2)) is the most popular index, having the same reference values for both sexes and all ages. However, the BMI may not reflect most accurately a person’s adiposity levels. Other measures and indices are needed to reflect the actual body fat percentage and, more importantly, fat distribution, as visceral fat (the so-called apple shape) appears to have more deleterious effects on health than subcutaneous fat, deposited preferentially around hips, and resulting in the “pear shape” pattern .
Assessment of obesity, adiposity, and fat distribution can be done through a variety of methods, from the simplest ones, requiring just a tape measure, such as body adiposity index (BAI, calculated as (hip circumference(cm)/(height(m))^1.5) − 18) [8] or waist-to-hip ratio, WHR; or requiring tape and scale (BMI) to the most sophisticated technology (e.g., magnetic resonance imaging, MRI; Table 1.2) [9].
Table 1.2
Methods to assess adiposity and obesity
Indices |
BMI |
BAI |
Waist circumference |
Waist-to-hip ratio |
Skinfold thickness |
Underwater weighing (densitometry) |
Air displacement plethysmography (BodPod) |
Dilution method (hydrometry) |
Bioelectrical impedance weighing scale |
Dual energy X-ray absorptiometry (DEXA) |
CT and MRI (percent fat, distribution) |
Intra-organ fat quantification (MRS) |
But how do we get here? How is energy homeostasis regulated such that if chronically energy intake exceeds energy expenditure we end up obese?
Control of Energy Homeostasis
Food intake and energy expenditure are controlled by the brain, which integrates a large variety of signals and generates behavioral and physiological changes. The main area of the central nervous system (CNS) that regulates energy homeostasis is the hypothalamus, specifically the arcuate nucleus (ARC), paraventricular nucleus (PVN), the lateral hypothalamic area (LHA), the ventromedial hypothalamus (VMH), and the dorsomedial hypothalamus (DMH). In addition to the hypothalamus, neural systems in the brain stem, cerebral cortex, olfactory areas, and elsewhere are involved. There are two types of neurons that control food intake: the anorexigenic neurons that co-express proopiomelanocortin (POMC), cocaine- and amphetamine-regulating transcript (CART), and nesfatin and the orexigenic neuropeptide Y–agouti-related protein–gamma -aminobutyric acid (NPY/AgRP/GABA) neurons. Activation of the POMC neurons results in the release of α-melanocyte-stimulating hormone (α-MSH) from POMC axon terminals, leading to inhibition of food intake and increase in energy expenditure. Conversely, the activation of NPY/AgRP/GABA neurons leads to increases in AgRP, which inhibits melanocortin cells, and has the opposite effect: stimulation of food intake [10]. It is important to mention that the reverse is not true; the unidirectionality of NPY–POMC neurons inhibition thus reflects the wiring of the system towards favoring positive energy balance, which in turn provides an explanation why gaining weight is easy but losing it is hard [11].
The presence of a meal results in nutrient-activated signals: the nutrients themselves (glucose, free fatty acids, and amino acids), hormonal release, or generation of neural signals. But unlike, for example, glucose homeostasis or sodium balance regulation, food intake, and energy balance regulation have an important hedonic and cultural aspect. Thus, one has to distinguish between physiologic and reward-driven circuits of energy homeostatic regulation. In addition, the signals for short-term (meal-related) and long-term (related to adiposity) regulation are sometimes overlapping, but certainly not identical.
The synthesis and release of these brain peptides involved in energy homeostasis are regulated by neural, hormonal, and metabolic-regulated signals related to meals, and control hunger and satiety: mostly gastrointestinal (hormones like cholecystokinin (CCK), glucagon-like peptide 1 (GLP-1), peptide YY (PYY)) as well as plasma glucose and amino acids but also others such as body temperature. The long-term circuits signal adiposity stores via leptin and insulin and are involved in regulating energy expenditure as well (Table 1.3) [12].
Positive energy balance | Negative energy balance |
---|---|
Proximal NPY AgRP Orexin A and B MCH Norepinephrine (α2, β-adrenergic) Endocannabinoids | Proximal POMC/ α-MSH CART Norepinephrine (a1 agonists) |
Distal Ghrelin | Distal CCK GLP-1 Oxyntomodulin PYY Amylin Adiponectin (increases energy expenditure) Pancreatic polypeptide PP Serotonin Insulin Leptin |
Gut Hormones
The gut secretes over 40 hormones with diverse cell localization and clustering. As expected, a large number of these hormones are related to nutrient sensing, relaying of information about nutrient amount and type, and to orchestration of energy balance and glucose, lipid, and protein.
CCK is a duodenal peptide released after high lipid and protein meals. It stimulates gall bladder contraction, exocrine pancreatic secretion, and inhibits gastric emptying and food intake through stimulation of vagal nerve endings. Moreover, there are recent data that CCK interacts with long-term signals of energy balance: CCK1R expressing neurons of the vagus also express receptors for ghrelin, orexin, cannabinoids (CB1), and leptin. CKK appears to control the expression of G-protein-coupled receptors and neurotransmitters involved in the control of food intake, further contributing to this regulation [15, 16].
Ghrelin, secreted by the P/D1 stomach cells, is a potent orexigenic hormone. Ghrelin levels increase before meals and decrease after meal ingestion, suggesting that ghrelin acts on the hypothalamic centers to promote meal initiation. Ghrelin infusion promotes food intake in humans, and it appears to change respiratory quotient, suggesting a role in energy expenditure. Ghrelin promotes food intake and adiposity by acting on brain centers, and is a weight regulator. In addition, ghrelin acts on vagal afferents in the gastrointestinal area to inhibit the effects of CCK and leptin [17, 18]. Ghrelin concentration in plasma is inversely related to fat mass; obese people have lower levels of ghrelin, but post-meal ghrelin levels remain higher than in lean people. Patients with Prader–Willi syndrome have high ghrelin levels, and consequently suffer from increased appetite, hyperphagia, and obesity . A disturbed gut–brain ghrelin axis has been suggested as one of the causes of anorexia nervosa [19].
GLP-1 is a 30-amino-acid peptide released from L cells in response to meal ingestion. L-cell stimulation increases not only GLP-1 but also GLP-1-related peptides, all derived from the same proglucagon molecule (glicentin, oxyntomodulin, intervening peptide-2, and GLP-2), as well as PYY. GLP-1 is a major insulinotropic hormone, but it also inhibits food and water intake and promotes satiety [20]. A meta-analysis of studies investigating the effect of GLP-1 on food intake found that GLP-1 reduced ad libitum caloric intake by 12 % in normal or obese humans [21]; subcutaneous administration of recombinant GLP-1 to obese humans reduced caloric intake by 15 % and produced weight loss [22]. A number of drugs based on GLP-1 and currently used for diabetes therapy (GLP-1 agonists and dipeptidyl peptidase IV (DPP-IV) inhibitors) have taken advantage of these effects and are currently investigated as obesity treatments. The GLP-1 agonists exenatide and liraglutide , which have longer half-life than the native compound, have been shown to inhibit food intake and promote weight loss (2.8–3.2 kg in one meta-analysis) [23]. The other group of GLP-1-based drugs, the DPP-IV inhibitors (sitagliptin, vildagliptin, linagliptin, saxagliptin, alogliptin), are weight neutral, a significant advantage in treating type 2 diabetes, since many anti-diabetes therapies result in weight gain [24].
PYY is a 36-amino-acid member of the polypeptide family that also includes NPY and pancreatic polypeptide. Though part of a different peptide family, PYY has many similarities with GLP-1. Like GLP-1, it is released by the L cells of the gastrointestinal tract, mainly in the ileum and colon, as well as by the brain. PYY is co-secreted from L cells with GLP-1 in response to meal stimulation (probably by both direct contact with luminal nutrients and via neural and endocrine mechanisms) . PYY inhibits gastric emptying and intestinal motility, being part of the “ileal brake” together with GLP-1. Its active form PYY 3-36 inhibits food intake by binding to Y-2 neuronal receptors and inhibiting the release of NPY [25, 26].
Other Hormones
Leptin is an adipocyte-derived hormone, an adipokine. Plasma leptin concentration correlates with body fat content and signals the adipose reserves: High leptin reduces food intake by inhibiting NPY/AgRP neurons and stimulating the α-MSH neurons. Leptin is an important signal for starvation: Low leptin increases food intake and suppresses energy expenditure; conversely, leptin is usually increased in obese subjects. Mice deficient in the leptin gene (ob/ob mice) or in the leptin receptor gene (db/db) mice are examples of genetically induced obesity, since the absence of the hormone or its receptor drives increased food intake and low energy expenditure resulting in obesity. Several medical conditions are associated with low leptin: lipodystrophy, hypothalamic amenorrhea, and anorexia nervosa . In contrast, obese patients have high leptin levels; diet-induced weight loss results in a decrease in plasma leptin concentration, which could contribute to the difficulty of weight loss maintenance, since it will promote hunger. In spite of the increased leptin levels, obese humans do not have abnormal appetite suppression. This is because most obese patients are leptin resistant, limiting the ability to treat obesity with leptin. The precise mechanism of this resistance is not known, though it is believed to include both impaired leptin transport into the brain and impaired signaling in the hypothalamus (ER (endoplasmic reticulum) stress; hypothalamic inflammation; and defective autophagy)[13, 27, 28].
Insulin, well known for its fundamental role in controlling blood glucose levels and glucose homeostasis, insulin plays an equally important role in controlling energy balance. Insulin receptors are abundant in CNS, and early work by Woods and Porte showed that intraventricular administration of insulin in laboratory animals inhibits food intake [29]. A large amount of research has been devoted since to understanding the role played by insulin in the CNS. Though the exact mechanisms are not completely understood, it is generally accepted that insulin crosses the blood–brain barrier in the hypothalamic area and inhibits the NPY/AgRP neurons (that stimulate food intake) via the insulin receptor substrate–phosphatidylinositol-3-OH kinase (IRS–PI3K) pathway. Moreover, insulin acts on nonhypothalamic areas, such as the midbrain areas (ventral tegmental area and substantia nigra), populated by dopaminergic neurons involved in reward pathways. The insulin inhibition of food intake is greatly decreased in obese patients. Recent research in preclinical models has shown that in cases of consumption of high-fat food and overweight (but not in lean controls) insulin release from the pancreas triggers a signaling cascade in steroidogenic factor 1 (SF-1)-expressing neurons of the VMH, resulting in inhibition of POMC neurons, which promotes food intake and perpetuates obesity [30, 31]. The action of insulin on the reward pathway dopaminergic neurons is thought to additionally contribute to development of obesity , since signaling of these higher neuronal circuits can override hypothalamic signaling. Wang et al. have proposed that reward-driven overconsumption of high-fat, high-sugar, and energy-dense foods leads to neuronal insulin resistance, dysregulation of dopamine homeostasis, and hypodopaminergic reward deficiency syndrome [32].
Afferent Pathways
Vagal neural afferents, which are abundant in the gastrointestinal area, integrate a variety of signals: hormonal (CCK, gastric leptin, GLP-1, ghrelin) or mechanical (gastric distension). The merging of multiple signals on the same vagal neuron allows integration of a large amount of information and rapid response and adaptability of the digestive system to the metabolic needs. Vagal afferents project to the nucleus of the solitary tract in the caudal brain stem; neurons in this area further project to the specific regions of hypothalamus involved in food intake and adiposity regulation [18].
Effector Signals
The brain modulates the activity of organs and systems involved in energy homeostasis (adipose tissue, pancreas, gut, liver, stomach, brain itself) via sympathetic and parasympathetic signals. Efferent pathways from hypothalamic energy expenditure network (EEN) and fat accrual network (FAN) transmit signals leading to effects on neuroendocrine systems (hormones), autonomic nervous system, and behavior, including feeding and physical activity [4]. Some of these effects favor weight loss: fatty acid oxidation and a reduction in adipose tissue mass, increased energy expenditure, decrease in food intake, and increased sympathetic activity to brown adipose tissue. Others favor fat accumulation and obesity: increased fat deposition, reduction in energy expenditure, and increased food intake. The sympathetic nervous system promotes a negative energy balance by increasing energy expenditure (movement and thermogenesis, while the parasympathetic system promotes energy storage by increasing alimentary tract digestion and absorption, adipose tissue insulin sensitivity, and insulin secretion [14].
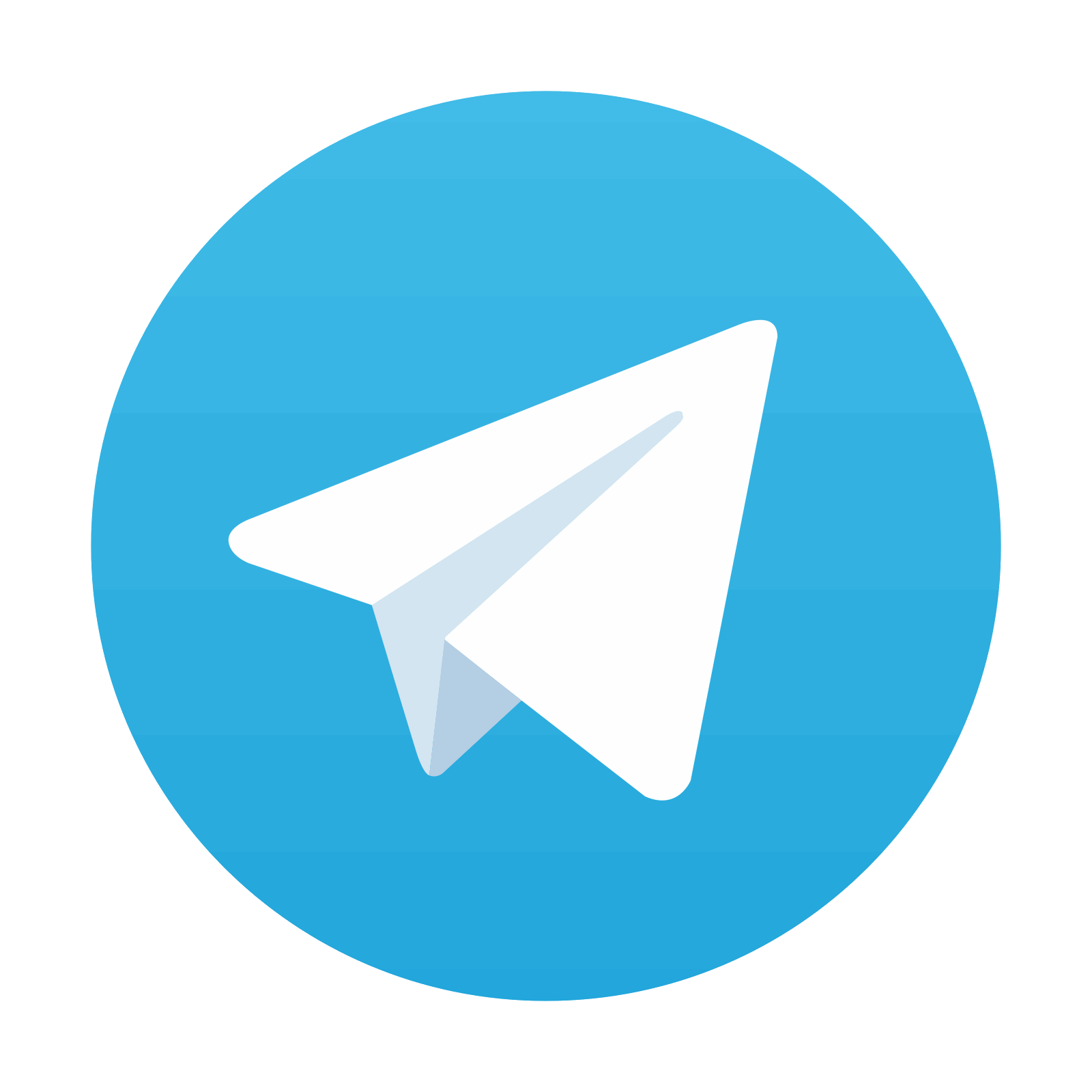
Stay updated, free articles. Join our Telegram channel

Full access? Get Clinical Tree
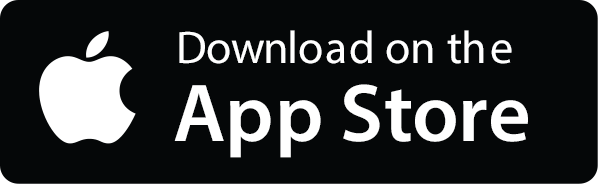
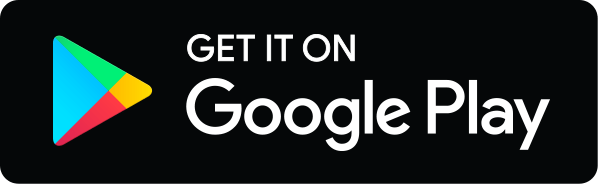