Before the days of transplantation and tyrosine kinase inhibitor therapy, all cases of chronic myelogenous leukemia would inevitably proceed from chronic phase through accelerated and blast crisis, implying that there is a program of progression set in motion by unopposed BCR-ABL activity. Since then, much work has identified a plethora of genetic changes associated with progression, the common themes being changes in self-renewal, differentiation, and deregulation of apoptotic pathway. Some of the genes and pathways uncovered offer some progress for research on novel therapy for blast crisis.
Tyrosine kinase inhibitors (TKIs) have vastly and irreversibly changed the course of chronic myelogenous leukemia (CML) treatment. The vast majority of these CML cases present in chronic phase, and the use of TKI can yield complete cytogenetic remissions, and long-term progression-free survival, in approximately 80% of cases. Unfortunately, patients sometimes present in advanced-phase CML (accelerated or blast phase), and for these cases treatments with TKI alone are ineffective. Moreover, the approximately 10% of chronic-phase cases that have initial resistance to TKI therapy, as well as about another 10% who become resistant to primary and secondary TKI therapy, are likely to progress to advanced-phase disease. Thus, an understanding of the process of CML progression may (1) offer new targets for therapy; (2) allow us to develop prognostic markers to predict patients at high risk of progression; and (3) tell us something about the “biological clock” that drives progression in CML and (hopefully) other malignancies.
Recently several outstanding reviews of CML progression have been published, and it is not the author’s intention to simply recapitulate them here. Rather, this article takes a different approach to looking at CML, in the spirit of solving a crime or a mystery: the author asks who, what, where, and why? With enough evidence, one can then try to infer how progression might be treated.
Who progresses?
There are several classification models of CML ( Table 1 ) that rely on clinical and pathologic features (blast counts, cytogenetics, splenomegaly, and so forth). The most recent change in classification was introduced by the World Health Organization, which decreased the percentage of blast counts needed to qualify for acute leukemia from 30% to 20%. That being said, it is unlikely that there is any biological difference in an “accelerated-phase” case with 17% blasts and a “blast-crisis” case with 20% blasts. Indeed, gene expression studies have shown that there is little difference between accelerated and blast crisis; rather, the biology of progression appears at the critical transition from chronic phase to accelerated phase. At that point, the proverbial horse is out of the barn.
Features | Sokol | IBMT | MDA | WHO |
---|---|---|---|---|
Blast % | ≥5% | ≥10% | ≥15% | 10%–19% |
Clonal evolution? | Yes | Yes | Yes | Yes |
Platelet count | Increased | Decreased | Decreased | Increased/decreased |
Blast count (BC) | ≥30% | ≥30% | ≥30% | ≥20% |
In the days before “curative” therapy for CML (transplantation and now, potentially, TKI), all cases of chronic phase eventually progressed, with the median time from diagnosis to accelerated phase of around 4 years. From this point, further progression to blast crisis took on the order of months. In the Western world, CML is generally diagnosed in chronic phase, and progression is limited to those cases resistant to TKI therapy. However, in Asia the disease appears to occur less frequently but in a younger population, and some surveys suggest that response to therapy is worse compared with Western countries, though it is unclear as to what contribution access and patterns of care, and biology, play in this pattern.
There appears to be a relationship of progression and resistance to TKI therapy. The most obvious link is the association of response with phase of disease. Complete cytogenetic response (CCyR) to imatinib is the rule in chronic-phase CML; in blast crisis there is an initial response in most patients, but a CCyR is relatively unusual, and if it occurs is generally short-lived. TKI resistance is often mediated by point mutations in the Abl tyrosine kinase domain, which may be associated with a relief of kinase inhibition, or may alter the signaling pathways of BCR-ABL. Resistance associated with Abl mutations is uncommon in newly diagnosed chronic-phase CML, and increases in frequency as time from diagnosis, or phase, progresses. Indeed, point mutations can be found in blast-crisis samples never exposed to TKIs.
The acquisition of point mutations may result from the genetic instability associated with BCR-ABL (see later discussion). Point mutations have been found in the CD34 + cells derived from CCyR patients. Thus, even in the context of an excellent response, residual CML stem cells remain, and while on therapy there is either an expansion of a resistant clone or the development of a clone. Lastly, gene expression arrays were performed on CML patients, who achieved a CCyR but then had a relapse into clinical chronic phase, most with point mutations. Many of these cases had gene expression profiles more similar to advanced-phase disease.
What is progression?
Blast crisis is fundamentally different to chronic phase in many aspects. By definition, accelerated phase and blast crisis are associated with other chromosomal structural changes ( Table 2 ). Often, progression is associated with additional chromosomal abnormalities that involve the Ph. “Double” Ph are seen in more than 30% of blast-crisis patients. Additional loss of chromosome 9 (der 9) occurs in 5% to 10% of chronic-phase patients; there are some data that these cases respond poorly to interferon, likely because this deletion eliminates critical interferon receptor genes. It is not clear whether the der 9 abnormality affects prognosis for patients treated with imatinib or transplantation.
Genetic Lesion | Mechanism | Functional Effects |
---|---|---|
Chromosomal | ||
BCR-ABL | Amplification | Myriad |
NUP98-HOXA9, AML1-EVI1 | Translocation | Differentiation |
TP53 | Deletion | Tumor suppressor |
P16/ARF | Deletion | Proliferation |
Point mutations | ||
TP53 | Point mutation | Tumor suppressor |
Expression/translation | ||
CEBPA | Translational block | Differentiation |
hnENPE2 | Increased expression | Differentiation |
PP2A | Inhibition by SET | Tumor suppressor |
Bcl2 | Increased expression | Apoptosis |
FOXO3A | Decreased expression | Apoptosis |
JunB | Decreased expression | Transcriptional regulation |
WT1 | Increased expression | Proliferation |
BMI1 | Expression | Proliferation |
Progression appears to be associated with an increase in BCR-ABL activity. The aberrant cellular activities of BCR-ABL have been well chronicled (reviewed nicely in Ref. ), including increased proliferation through the activation of RAS ; increased transcriptional activity via STAT recruitment ; decreases in apoptosis through activation of PI(3)K/AKT ; and changes in adhesion binding to actin with phosphorylation to cytoskeletal proteins. Blast phase is accompanied by an increase in both BCR-ABL mRNA and protein level. Although the levels are modestly increased (∼3 fold for both message and protein), they are associated with an increase in activation of signaling (as evidenced by increased Crkl phosphorylation) and in vitro changes of clonality, growth factor independence, proliferation, and block in apoptosis. In mouse models, cells constructed to produce more BCR-ABL have a more rapid tumor development. Because BCR-ABL level is associated with progression, a logical question is whether this phenomenon also plays a role in imatinib resistance. A model using cell lines cultured in imatinib to develop resistance suggested high BCR-ABL was associated with a shorter time to the development of Abl point mutations. Conversely, a model of human CD34 + cells transduced with BCR-ABL found that high BCR-ABL expression was associated with increased sensitivity to imatinib, suggesting that such cells were “addicted” to high levels of BCR-ABL. Of note is that the mechanism of increased BCR-ABL mRNA and protein levels in progressive disease is unclear, while because some cases of blast crisis have multiple copies of the Ph chromosome, such abnormalities are not the rule.
Increased BCR-ABL activity is also associated with a change in mRNA processing. The most notable changes associated with progression involve alternative splicing glycogen synthase kinase 3B (GSK3B). GSK3B is involved in the inactivation of β-catenin, and the aberrant splicing is thought to inhibit proper functioning, allowing β-catenin to activate transcription and thus increase self-renewal pathways. In addition, aberrant splicing of Ikaros (IKZF1) has been described in cases of lymphoid blast crisis, thus making the functional depletion of IKZF1 through deletion or missplicing quite common in that disease. In addition, studies of the RNA-binding proteins Lin28 and Lin28B have been shown to be elevated in CML progression. LIN28B is 1 of the 4 genes (with OCT4, NONOG, and SOX2) that can “reprogram” human fibroblast to a pluripotent state. Lin28/28B block let-7 miRNA processing into mature miRNAs; given that the let-7 family is repressed in human malignancies, the elevation of Lin28B in blast crisis is likely functionally significant.
Is Genetic Instability the Key Driver or Progression?
The main functional changes that occur with progression of CML are marked changes in proliferation, differentiation, apoptosis, and adhesion. These functional changes accompanying progression are accompanied by profound changes in treatment response. But what drives progression?
There is growing evidence that BCR-ABL itself is a direct cause of the genetic instability seen in progression. While the contributions of these pathways in the initiation CML are logical, it is not clear that these pathways alone drive progression from chronic phase to advanced phase. However, insights that BCR-ABL causes genetic instability may prove to be a unifying theme in starting the basis for progression at the very beginning of CML. When considering the effect of BCR-ABL on genetic instability, one must confront a seeming paradox: cell lines with activated tyrosine kinases, such as BCR-ABL, accumulate more DNA damage than those cell lines without activated kinases, yet these cell lines also repair the DNA damage faster. The combination of more DNA damage and repair activity may lead to less accurate repair. As BCR-ABL upregulates antiapoptosis genes BCL2 and BCL-Xl, thus causing G2/M delay, cells accumulate DNA damage but are not targeted for cell death. It also appears that BCR-ABL directly causes DNA damage by increasing reactive oxygen species (ROS) ; ROS leads to DNA base-pair transversions (GC→TA) and transitions (GC→AT). Thus, unbridled BCR-ABL activity may contribute to the genetic instability leading to chromosomal aberrations, mutations, and changes in gene expression that cause progression.
Genetic Structural Changes
Chromosomal changes in addition to the Ph are the norm in blast crisis. Isochromosome i(17q) is a relatively common occurrence (∼20% of cases of blast-crisis cases), and this is likely associated with progression, as it means the loss of a copy of the p53 tumor suppressor gene. However, the remaining p53 allele does not seem to be mutated in these cases, so a direct link of p53 inactivation and progress to blast crisis is not so clear, though the reduction of the total cellular p53 level may upset the integration of genetic repair and apoptosis and thus contribute to progression. Alternatively, there may be critical but yet unknown genes on 17q contributing to progression. Trisomy 8 is also common in blast crisis (∼40%), which is interesting because Myc is located at 8q24. There are several lines of evidence linking MYC to progression. In vitro inhibition of c-Myc with antisense oligonucleotides, or dominant-negative constructs, can inhibit BCR-ABL transformation or leukemogenesis. Myc is often overexpressed in blast crisis compared with chronic phase ; whereas in acute myelogenous leukemia (AML) cases with trisomy 8, c-Myc is downregulated, but other genes on chromosome 8 are upregulated. However, trisomy 8 is a common feature of cases of CML that exhibit clonal evolution Ph-negative cells while in a CCyR (see later discussion). These cases of Ph-negative, trisomy 8 appear to have a benign course, suggesting that trisomy 8 may not be leukemogenic.
Translocations of known oncogenes in blast crisis occur relatively rarely (<5%). The most notable of these recurrent translocations are t(3;21) and t(7;11), involving the AML-1/EVI-1 and NUP98/HOXA9 genes, respectively. Evi-1 and HOXA9 are both transcription factors, and their aberrant expression in the context of these fusion proteins causes differentiation arrest in the case of AML-1/EVI-1, and increased proliferation in the case of NUP98/HOXAP. In mouse models, BCR-ABL and AML-1/EVI-1 coexpression creates a disease produces a picture of myeloid leukemia, whereas mice transplanted with cells transfected with NUP98/HOXAP develop a myeloproliferative disease that evolves into an acute leukemia. Recently it was shown that NUP98-HOXA9 increased the expression of the RNA binding protein Musashi 2 (Msi2), which in turn repressed the Numb expression, involved in the control of Notch signaling. Msi2 was found to be overexpressed in blast-crisis cases compared with chronic phase; moreover, increased Msi2 expression was found to be associated with poorer survival in all phases of CML following allogeneic transplantation.
Disruption of Critical Pathways in Progression
Activation of the MAPK proliferation pathway is central in myeloid leukemia (BCR-ABL in CML, RAS, FLT3, kit mutations in AML). Thus, it is not surprising that RAS and FLT3 mutations are rare in CML progression, because their activation would not add to the selective advantage already conferred by BCR-ABL. The tumor suppressor p53, inactivated in many solid tumors, is occasionally involved in blast crisis (∼20%–30%); it is not known if there are aberrations in genes involved in p53-mediated function. In lymphoid blast crisis (but not myeloid), a homozygous deletion of exon 2 of INK4A/ARF occurs commonly (∼50%). This deletion eliminates both p16 and p19, two proteins that normally check G1/S cell cycle progression and upregulate p53.
The activity of the tumor suppressor PP2A may be involved in the pathogenesis of CML progression and may provide a new drug target. PP2A activity is involved in regulating proliferation, survival, and differentiation, and is involved in the proteosomal degradation of BCR-ABL. Increasing BCR-ABL levels (induced in vitro or in human leukemia) increases the expression of the phosphoprotein SET, a negative regulator of PP2A. Thus, progression may set up a feedback loop whereby increasing BCR-ABL increases SET, decreasing PP2A, which further ensures the persistence of BCR-ABL. In in vitro and mouse models, restoration of PP2A activity by the activator forskolin appeared to decrease BCR-ABL leukemic potential, thus suggesting a potential therapeutic target to arrest or regress CML progression.
A block in myeloid differentiation occurs in progression, contributing to the accumulation of immature blasts. A search for mutations differentiation genes has been relatively unrewarding. For example, the CCAAT/enhancer binding protein α (CEBPA) is a member of the basic leucine zipper family of transcription factors essential to the control of granulocytic differentiation, regulating several genes necessary for orderly differentiation, including CSFR3. Inhibition of CEBPA in t(8;21) AML occurs through repression on CEBPA by the fusion AML1-ETO protein. In blast-crisis CML, high levels of BCR-ABL induce the MAPK phosphorylation of the poly(rC) binding protein hnENPE2, which then causes the translation block of CEBPA mRNA. However, complete loss of CEBPA, experimentally performed by transducing BCR-ABL into primitive murine cells engineered to have CEPBA deleted, confers an erythroid leukemia rather than a myeloproliferative disease. This implies that some residual CEPBA activity must be present, even in blast crisis, and that disturbances in proliferation in CML progression may result from subtle changes in the regulation of factors critical in controlling the flow of hematopoietic differentiation.
Genes associated with the maintenance of stem cell renewal have been logically associated with progression. Data from several sources seem to be converging on the Wnt/β-catenin pathway as critical for the evolution of CML. The Wnt/β-catenin signaling pathway has come front and center in normal and abnormal hematopoiesis. Wnt/β-catenin signaling is thought to be important in cell self-renewal, and mutations in β-catenin have been found in various epithelial solid tumors. Aberrant Wnt/β-catenin signaling has thus been demonstrated in CML and T-lineage acute lymphoblastic leukemia (ALL). In CML, activation of the Wnt/β-catenin pathway was observed in primary cell samples from patients with CML, with levels increasing with progression. β-Catenin activation in CML seemed to reside predominately in granulocyte-macrophage progenitor cells, and the self-renewal of these progenitors could be reduced by overexpression of Axin, which modulates free β-catenin.
Several transcription factors appear to be involved in CML progression. Jun B knock-out mice develop a myeloproliferative disorder similar to CML. Jun B has been shown to be downregulated in CML progression, and the decrease in junB does not appear to be mediated by methylation changes of junB promoters. Genes controlled by the transcription factors MZF1 and delta EF1 appear to be deregulated in CML progression. MZF1 is a member of the Kruppel family of zinc finger proteins originally cloned from a cDNA library from a blast-crisis CML patient, and plays a critical role in hematopoietic stem cell differentiation, including modulation of CD34 and c-myb expression, and MZF1 −/− knock-out mice display an increase in hematopoietic progenitor proliferation, which continues in long-term culture conditions. Both MZF1 and delta EF1 have been shown to influence cadherin expression. Moreover, MDFI, an inhibitor of myogenic basic helix-loop-helix transcription factors found overexpressed in CML progression, both interacts with axin and influences Jun signaling, thus perhaps linking these two pathways implicated in CML progression.
What is progression?
Blast crisis is fundamentally different to chronic phase in many aspects. By definition, accelerated phase and blast crisis are associated with other chromosomal structural changes ( Table 2 ). Often, progression is associated with additional chromosomal abnormalities that involve the Ph. “Double” Ph are seen in more than 30% of blast-crisis patients. Additional loss of chromosome 9 (der 9) occurs in 5% to 10% of chronic-phase patients; there are some data that these cases respond poorly to interferon, likely because this deletion eliminates critical interferon receptor genes. It is not clear whether the der 9 abnormality affects prognosis for patients treated with imatinib or transplantation.
Genetic Lesion | Mechanism | Functional Effects |
---|---|---|
Chromosomal | ||
BCR-ABL | Amplification | Myriad |
NUP98-HOXA9, AML1-EVI1 | Translocation | Differentiation |
TP53 | Deletion | Tumor suppressor |
P16/ARF | Deletion | Proliferation |
Point mutations | ||
TP53 | Point mutation | Tumor suppressor |
Expression/translation | ||
CEBPA | Translational block | Differentiation |
hnENPE2 | Increased expression | Differentiation |
PP2A | Inhibition by SET | Tumor suppressor |
Bcl2 | Increased expression | Apoptosis |
FOXO3A | Decreased expression | Apoptosis |
JunB | Decreased expression | Transcriptional regulation |
WT1 | Increased expression | Proliferation |
BMI1 | Expression | Proliferation |
Progression appears to be associated with an increase in BCR-ABL activity. The aberrant cellular activities of BCR-ABL have been well chronicled (reviewed nicely in Ref. ), including increased proliferation through the activation of RAS ; increased transcriptional activity via STAT recruitment ; decreases in apoptosis through activation of PI(3)K/AKT ; and changes in adhesion binding to actin with phosphorylation to cytoskeletal proteins. Blast phase is accompanied by an increase in both BCR-ABL mRNA and protein level. Although the levels are modestly increased (∼3 fold for both message and protein), they are associated with an increase in activation of signaling (as evidenced by increased Crkl phosphorylation) and in vitro changes of clonality, growth factor independence, proliferation, and block in apoptosis. In mouse models, cells constructed to produce more BCR-ABL have a more rapid tumor development. Because BCR-ABL level is associated with progression, a logical question is whether this phenomenon also plays a role in imatinib resistance. A model using cell lines cultured in imatinib to develop resistance suggested high BCR-ABL was associated with a shorter time to the development of Abl point mutations. Conversely, a model of human CD34 + cells transduced with BCR-ABL found that high BCR-ABL expression was associated with increased sensitivity to imatinib, suggesting that such cells were “addicted” to high levels of BCR-ABL. Of note is that the mechanism of increased BCR-ABL mRNA and protein levels in progressive disease is unclear, while because some cases of blast crisis have multiple copies of the Ph chromosome, such abnormalities are not the rule.
Increased BCR-ABL activity is also associated with a change in mRNA processing. The most notable changes associated with progression involve alternative splicing glycogen synthase kinase 3B (GSK3B). GSK3B is involved in the inactivation of β-catenin, and the aberrant splicing is thought to inhibit proper functioning, allowing β-catenin to activate transcription and thus increase self-renewal pathways. In addition, aberrant splicing of Ikaros (IKZF1) has been described in cases of lymphoid blast crisis, thus making the functional depletion of IKZF1 through deletion or missplicing quite common in that disease. In addition, studies of the RNA-binding proteins Lin28 and Lin28B have been shown to be elevated in CML progression. LIN28B is 1 of the 4 genes (with OCT4, NONOG, and SOX2) that can “reprogram” human fibroblast to a pluripotent state. Lin28/28B block let-7 miRNA processing into mature miRNAs; given that the let-7 family is repressed in human malignancies, the elevation of Lin28B in blast crisis is likely functionally significant.
Is Genetic Instability the Key Driver or Progression?
The main functional changes that occur with progression of CML are marked changes in proliferation, differentiation, apoptosis, and adhesion. These functional changes accompanying progression are accompanied by profound changes in treatment response. But what drives progression?
There is growing evidence that BCR-ABL itself is a direct cause of the genetic instability seen in progression. While the contributions of these pathways in the initiation CML are logical, it is not clear that these pathways alone drive progression from chronic phase to advanced phase. However, insights that BCR-ABL causes genetic instability may prove to be a unifying theme in starting the basis for progression at the very beginning of CML. When considering the effect of BCR-ABL on genetic instability, one must confront a seeming paradox: cell lines with activated tyrosine kinases, such as BCR-ABL, accumulate more DNA damage than those cell lines without activated kinases, yet these cell lines also repair the DNA damage faster. The combination of more DNA damage and repair activity may lead to less accurate repair. As BCR-ABL upregulates antiapoptosis genes BCL2 and BCL-Xl, thus causing G2/M delay, cells accumulate DNA damage but are not targeted for cell death. It also appears that BCR-ABL directly causes DNA damage by increasing reactive oxygen species (ROS) ; ROS leads to DNA base-pair transversions (GC→TA) and transitions (GC→AT). Thus, unbridled BCR-ABL activity may contribute to the genetic instability leading to chromosomal aberrations, mutations, and changes in gene expression that cause progression.
Genetic Structural Changes
Chromosomal changes in addition to the Ph are the norm in blast crisis. Isochromosome i(17q) is a relatively common occurrence (∼20% of cases of blast-crisis cases), and this is likely associated with progression, as it means the loss of a copy of the p53 tumor suppressor gene. However, the remaining p53 allele does not seem to be mutated in these cases, so a direct link of p53 inactivation and progress to blast crisis is not so clear, though the reduction of the total cellular p53 level may upset the integration of genetic repair and apoptosis and thus contribute to progression. Alternatively, there may be critical but yet unknown genes on 17q contributing to progression. Trisomy 8 is also common in blast crisis (∼40%), which is interesting because Myc is located at 8q24. There are several lines of evidence linking MYC to progression. In vitro inhibition of c-Myc with antisense oligonucleotides, or dominant-negative constructs, can inhibit BCR-ABL transformation or leukemogenesis. Myc is often overexpressed in blast crisis compared with chronic phase ; whereas in acute myelogenous leukemia (AML) cases with trisomy 8, c-Myc is downregulated, but other genes on chromosome 8 are upregulated. However, trisomy 8 is a common feature of cases of CML that exhibit clonal evolution Ph-negative cells while in a CCyR (see later discussion). These cases of Ph-negative, trisomy 8 appear to have a benign course, suggesting that trisomy 8 may not be leukemogenic.
Translocations of known oncogenes in blast crisis occur relatively rarely (<5%). The most notable of these recurrent translocations are t(3;21) and t(7;11), involving the AML-1/EVI-1 and NUP98/HOXA9 genes, respectively. Evi-1 and HOXA9 are both transcription factors, and their aberrant expression in the context of these fusion proteins causes differentiation arrest in the case of AML-1/EVI-1, and increased proliferation in the case of NUP98/HOXAP. In mouse models, BCR-ABL and AML-1/EVI-1 coexpression creates a disease produces a picture of myeloid leukemia, whereas mice transplanted with cells transfected with NUP98/HOXAP develop a myeloproliferative disease that evolves into an acute leukemia. Recently it was shown that NUP98-HOXA9 increased the expression of the RNA binding protein Musashi 2 (Msi2), which in turn repressed the Numb expression, involved in the control of Notch signaling. Msi2 was found to be overexpressed in blast-crisis cases compared with chronic phase; moreover, increased Msi2 expression was found to be associated with poorer survival in all phases of CML following allogeneic transplantation.
Disruption of Critical Pathways in Progression
Activation of the MAPK proliferation pathway is central in myeloid leukemia (BCR-ABL in CML, RAS, FLT3, kit mutations in AML). Thus, it is not surprising that RAS and FLT3 mutations are rare in CML progression, because their activation would not add to the selective advantage already conferred by BCR-ABL. The tumor suppressor p53, inactivated in many solid tumors, is occasionally involved in blast crisis (∼20%–30%); it is not known if there are aberrations in genes involved in p53-mediated function. In lymphoid blast crisis (but not myeloid), a homozygous deletion of exon 2 of INK4A/ARF occurs commonly (∼50%). This deletion eliminates both p16 and p19, two proteins that normally check G1/S cell cycle progression and upregulate p53.
The activity of the tumor suppressor PP2A may be involved in the pathogenesis of CML progression and may provide a new drug target. PP2A activity is involved in regulating proliferation, survival, and differentiation, and is involved in the proteosomal degradation of BCR-ABL. Increasing BCR-ABL levels (induced in vitro or in human leukemia) increases the expression of the phosphoprotein SET, a negative regulator of PP2A. Thus, progression may set up a feedback loop whereby increasing BCR-ABL increases SET, decreasing PP2A, which further ensures the persistence of BCR-ABL. In in vitro and mouse models, restoration of PP2A activity by the activator forskolin appeared to decrease BCR-ABL leukemic potential, thus suggesting a potential therapeutic target to arrest or regress CML progression.
A block in myeloid differentiation occurs in progression, contributing to the accumulation of immature blasts. A search for mutations differentiation genes has been relatively unrewarding. For example, the CCAAT/enhancer binding protein α (CEBPA) is a member of the basic leucine zipper family of transcription factors essential to the control of granulocytic differentiation, regulating several genes necessary for orderly differentiation, including CSFR3. Inhibition of CEBPA in t(8;21) AML occurs through repression on CEBPA by the fusion AML1-ETO protein. In blast-crisis CML, high levels of BCR-ABL induce the MAPK phosphorylation of the poly(rC) binding protein hnENPE2, which then causes the translation block of CEBPA mRNA. However, complete loss of CEBPA, experimentally performed by transducing BCR-ABL into primitive murine cells engineered to have CEPBA deleted, confers an erythroid leukemia rather than a myeloproliferative disease. This implies that some residual CEPBA activity must be present, even in blast crisis, and that disturbances in proliferation in CML progression may result from subtle changes in the regulation of factors critical in controlling the flow of hematopoietic differentiation.
Genes associated with the maintenance of stem cell renewal have been logically associated with progression. Data from several sources seem to be converging on the Wnt/β-catenin pathway as critical for the evolution of CML. The Wnt/β-catenin signaling pathway has come front and center in normal and abnormal hematopoiesis. Wnt/β-catenin signaling is thought to be important in cell self-renewal, and mutations in β-catenin have been found in various epithelial solid tumors. Aberrant Wnt/β-catenin signaling has thus been demonstrated in CML and T-lineage acute lymphoblastic leukemia (ALL). In CML, activation of the Wnt/β-catenin pathway was observed in primary cell samples from patients with CML, with levels increasing with progression. β-Catenin activation in CML seemed to reside predominately in granulocyte-macrophage progenitor cells, and the self-renewal of these progenitors could be reduced by overexpression of Axin, which modulates free β-catenin.
Several transcription factors appear to be involved in CML progression. Jun B knock-out mice develop a myeloproliferative disorder similar to CML. Jun B has been shown to be downregulated in CML progression, and the decrease in junB does not appear to be mediated by methylation changes of junB promoters. Genes controlled by the transcription factors MZF1 and delta EF1 appear to be deregulated in CML progression. MZF1 is a member of the Kruppel family of zinc finger proteins originally cloned from a cDNA library from a blast-crisis CML patient, and plays a critical role in hematopoietic stem cell differentiation, including modulation of CD34 and c-myb expression, and MZF1 −/− knock-out mice display an increase in hematopoietic progenitor proliferation, which continues in long-term culture conditions. Both MZF1 and delta EF1 have been shown to influence cadherin expression. Moreover, MDFI, an inhibitor of myogenic basic helix-loop-helix transcription factors found overexpressed in CML progression, both interacts with axin and influences Jun signaling, thus perhaps linking these two pathways implicated in CML progression.
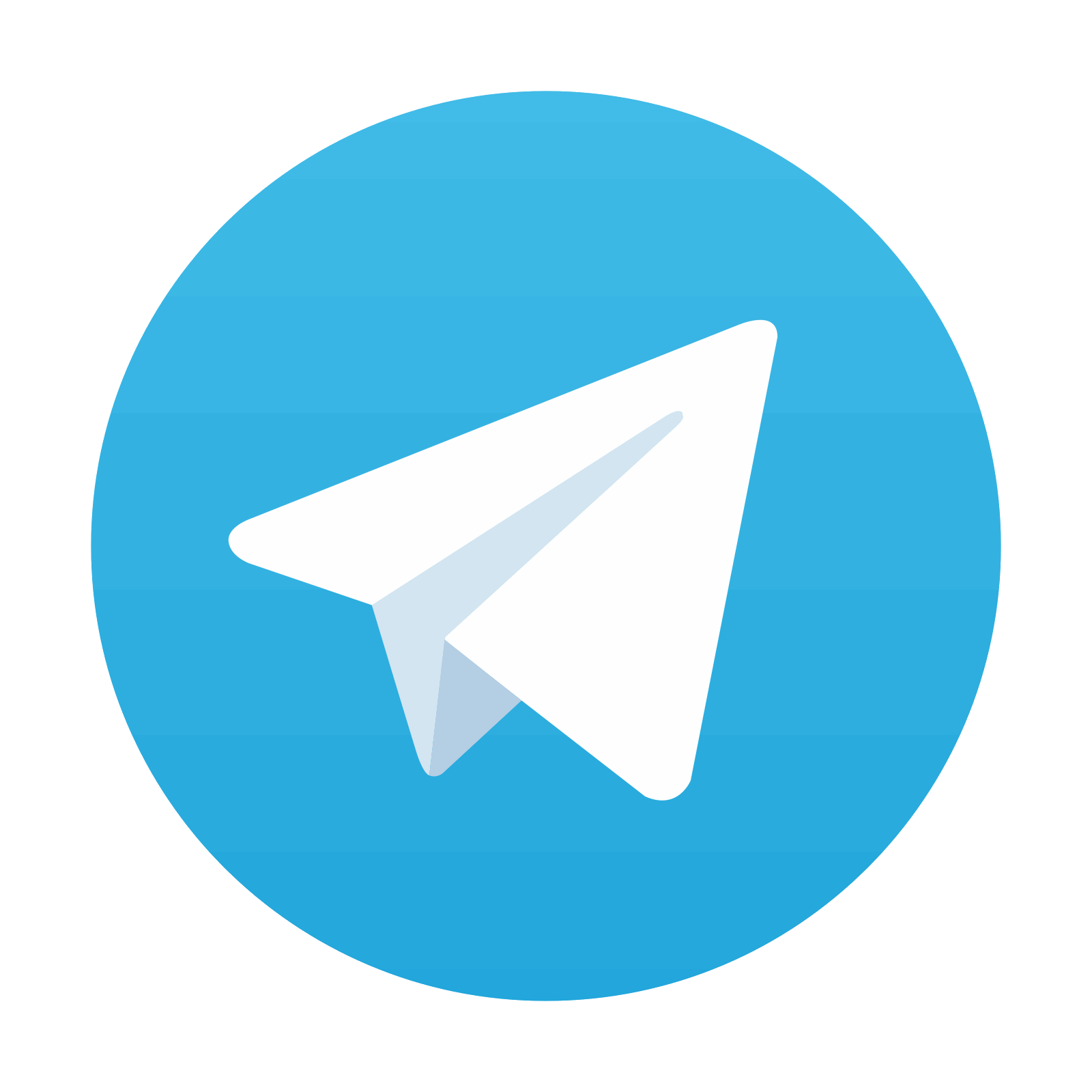
Stay updated, free articles. Join our Telegram channel

Full access? Get Clinical Tree
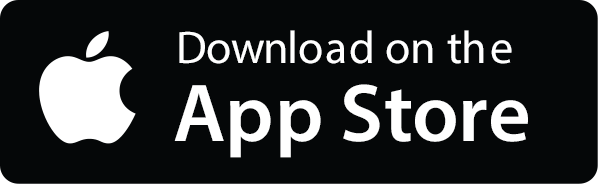
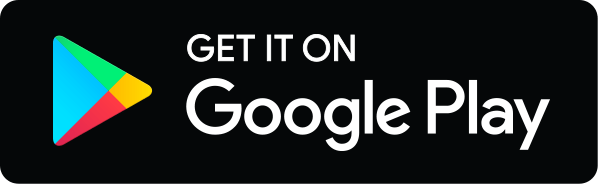