Abbreviations
- ACTH Adrenocorticotropic hormone
- cAMP Cyclic adenosine monophosphate
- DHEA Dehydroepiandrosterone
- DHT Dihydrotestosterone
- FSH Follicle-stimulating hormone
- GnRH Gonadotropin-releasing hormone
- hCG Human chorionic gonadotropin
- ICSI Intracytoplasmic sperm injection
- IVF In vitro fertilization
- LH Luteinizing hormone
- mRNA Messenger ribonucleic acid
- PDE5 Type 5 phosphodiesterase
- PRL Prolactin
- SHBG Sex hormone–binding globulin
- TGFβ Transforming growth factor β
Testes: Introduction
The testes contain two major components that are structurally separate and serve different functions. The Leydig cells, or interstitial cells, comprise the major endocrine component. The primary secretory product of these cells, testosterone, is responsible either directly or indirectly for embryonic differentiation along male lines of the external and internal genitalia, male secondary sexual development at puberty, and maintenance of libido and potency in the adult male. The seminiferous tubules comprise the bulk of the testes and are responsible for the production of approximately 30 million spermatozoa per day during male reproductive life (puberty to death).
Both of these testicular components are interrelated, and both require an intact hypothalamic-pituitary axis for initiation and maintenance of their function. In addition, several accessory genital structures are required for the functional maturation and transport of spermatozoa. Thus, disorders of the testes, hypothalamus, pituitary, or accessory structures may result in abnormalities of androgen or gamete production, infertility, or a combination of these problems.
Anatomy and Structure-Function Relationships
Figure 12–1
Male genital system. A. The testis and the epididymis are in different scales from the other parts of the reproductive system. Observe the communication between the testicular lobules. B. Structural organization of the human seminiferous tubule and interstitial tissue. This figure does not show the lymphatic vessels frequently found in the connective tissue.
(A and B reproduced, with permission, from Junqueira LC, Carneiro J, Kelley RO. Basic Histology. 9th ed. McGraw-Hill; 1999.)
C. Section of human testis.
(C reproduced, with permission, from Ganong WF. Review of Medical Physiology. 20th ed. McGraw-Hill; 2001.)
The adult testis is a prolate spheroid with a mean volume of 18.6 ± 4.8 mL. The average length is 4.6 cm (range, 3.6-5.5 cm), and the average width is 2.6 cm (range, 2.1-3.2 cm). The testes are located within the scrotum, which not only serves as a protective envelope but also helps to maintain the testicular temperature approximately 2°C (35.6°F) below abdominal temperature. Three layers of membranes—visceral tunica vaginalis, tunica albuginea, and tunica vasculosa—comprise the testicular capsule. Extensions of the tunica albuginea into the testicle as fibrous septa result in the formation of approximately 250 to 300 pyramidal lobules, each of which contains coiled seminiferous tubules. Within each testis there are over 400 meters of seminiferous tubules, and these structures account for about half of the testicular mass. The approximately 200 million androgen-producing Leydig cells, as well as the blood and lymphatic vessels, nerves, and fibroblasts, are interspersed between the seminiferous tubules.
The blood supply to the testes is derived chiefly from the testicular arteries, which are branches of the internal spermatic arteries. After traversing a complicated capillary network, blood enters multiple testicular veins that form an anastomotic network, the pampiniform plexus. The pampiniform plexuses coalesce to form the internal spermatic veins. The right spermatic vein drains directly into the vena cava; the left enters the renal vein.
The seminiferous tubules in the adult average 165 μm in diameter and are composed of Sertoli cells and germinal cells. The Sertoli cells line the basement membrane and form tight junctions with other Sertoli cells. These tight junctions prevent the passage of proteins from the interstitial space into the lumens of the seminiferous tubules, thus establishing a blood–testis barrier. Through extension of cytoplasmic processes, the Sertoli cells surround developing germ cells and provide an environment essential for germ cell differentiation. In addition, these cells have been shown to be responsible for the movement of germ cells from the base of the tubule toward the lumen and for the release of mature sperm into the lumen. These cells also actively phagocytose damaged germ cells and residual bodies, which are portions of the germ cell cytoplasm not used in the formation of spermatozoa. Finally, in response to follicle-stimulating hormone (FSH) or testosterone, the Sertoli cells secrete androgen-binding protein, a molecule with high affinity for androgens. This substance, which enters the tubular lumen, provides a high concentration of testosterone to the developing germinal cells during the process of spermatogenesis.
More than a dozen different types of germ cells have been described in males. Broadly, they can be classified as spermatogonia, primary spermatocytes, secondary spermatocytes, spermatids, and spermatozoa. Spermatogenesis occurs in an orderly fashion, with the spermatocytes being derived from the spermatogonia via mitotic division. Through meiotic (or reduction) division, the spermatids are formed; they contain a haploid number of chromosomes (23). The interval from the beginning of spermatogenesis to release of mature spermatozoa into the tubular lumen is approximately 64 days. Although there is little variation in the duration of the spermatogenic cycle, a cross-section of a seminiferous tubule demonstrates several stages of germ cell development.
The seminiferous tubules empty into a highly convoluted anastomotic network of ducts called the rete testis. Spermatozoa are then transported through efferent ductules and into a single duct, the epididymis, by testicular fluid pressure, ciliary motion, and contraction of the efferent ductules. During the approximately 12 to 14 days required for transit through the epididymis, spermatozoa undergo morphologic and functional changes essential to confer on the gametes the capacity for fertilizing an ovum. The epididymis also serves as a reservoir for sperm. Spermatozoa stored in the epididymis enter the vas deferens, a muscular duct 35 to 50 cm long that propels its contents by peristaltic motion into the ejaculatory duct.
In addition to the spermatozoa and the secretory products of the testes, retia testis, and epididymides, the ejaculatory ducts receive fluid from the seminal vesicles. These paired structures, 4.5 to 5.5 cm long, are composed of alveolar glands, connective tissue, and muscle. They are the source of seminal plasma fructose, which provides nourishment to the spermatozoa. In addition, the seminal vesicles secrete seminogelin I, phosphorylcholine, ergothioneine, flavins, prostaglandins, and antioxidants, including superoxide dismutase, catalase, and ascorbic acid. About 60% of the total volume of seminal fluid is derived from the seminal vesicles.
The ejaculatory ducts terminate in the prostatic urethra. There additional fluid (∼20% of total volume) is added by the prostate, a tubuloalveolar gland with a fibromuscular stroma that weighs about 20 g and measures 4 cm × 2 cm × 3 cm. The constituents of the prostate fluid include spermine, citric acid, cholesterol, phospholipids, fibrinolysin, fibrinogenase, zinc, acid phosphatase, and prostate-specific antigen, a 34-kDa kallikrein-like serine protease. Fluid is also added to the seminal plasma by the bulbourethral (Cowper) glands and urethral (Littre) glands during its transit through the penile urethra.
Physiology of the Male Reproductive System
Figure 12–2
Pathways for testicular androgen and estrogen biosynthesis. Heavy arrows indicate major pathways. Circled numbers represent enzymes as follows: ①, CYP11A1 (20,22-desmolase; P450scc); ②, 3β-hydroxysteroid dehydrogenase and Δ5,Δ4-isomerase II (3βHSDII); ③, CYP17 (17α-hydroxylase; P450c17); ④, CYP17 (17,20-lyase; P450c17); ⑤, 17α-HSD3 (17α-hydroxysteroid dehydrogenase 3; 17-ketoreductase); ⑥, 5α-reductase; ⑦, CYP19 (aromatase). StAR, steroidogenic acute regulatory protein. (See also Figures 9–4, 13–4, and 14–13.)
The three steroids of primary importance in male reproductive function are testosterone, dihydrotestosterone, and estradiol. From a quantitative standpoint, the most important androgen is testosterone. Over 95% of the testosterone is secreted by the testicular Leydig cells. In addition to testosterone, the testes secrete small amounts of the potent androgen dihydrotestosterone and the weak androgens dehydroepiandrosterone (DHEA) and androstenedione. The Leydig cells also secrete small quantities of estradiol, estrone, pregnenolone, progesterone, 17α-hydroxypregnenolone, and 17α-hydroxyprogesterone. The steps in testicular androgen biosynthesis are illustrated in Figure 12–2.
Dihydrotestosterone and estradiol are derived not only by direct secretion from the testes but also by conversion in peripheral tissues of androgen and estrogen precursors secreted by both the testes and the adrenals. Thus, about 80% of the circulating concentration of these two steroids is derived from such peripheral conversion. Table 12–1 summarizes the approximate contributions of the testes, adrenals, and peripheral tissues to the circulating levels of several sex steroid hormones in men.
Hormone | Testicular Secretion | Adrenal Secretion | Peripheral Conversion of Precursors |
---|---|---|---|
Testosterone | 95 | <1 | <5 |
Dihydrotestosterone | <20 | <1 | 80 |
Estradiol | <20 | <1 | 80 |
Estrone | <2 | <1 | 98 |
DHEA sulfate | <10 | 90 | — |
In the blood, androgens and estrogens exist in either a free (unbound) state or as bound to serum proteins. Although about 38% of testosterone is weakly bound to albumin, the major binding protein is sex hormone–binding globulin (SHBG), a high affinity, low capacity binding protein, which binds 60% of the testosterone. This glycosylated dimeric protein is homologous to, yet distinct from, the androgen-binding protein secreted by the Sertoli cells. SHBG is synthesized in the liver, with the gene located on the short arm of chromosome 17. The serum concentrations of this protein are increased by estrogen, tamoxifen, phenytoin, or thyroid hormone administration and by hyperthyroidism and cirrhosis and are decreased by exogenous androgens, glucocorticoids, or growth hormone and by hypothyroidism, acromegaly, obesity, and hyperinsulinemic states. About 2% of the circulating testosterone is not bound to serum proteins and is able to enter cells and exert its metabolic effects. In addition, some of the albumin-bound testosterone may dissociate from the protein and enter target tissues; thus, the amount of bioavailable testosterone may be greater than just the amount of non–protein-bound testosterone.
As noted later, testosterone may be converted to dihydrotestosterone within specific androgen target tissues. Most circulating testosterone is converted primarily by the liver into various metabolites such as androsterone and etiocholanolone, which, after conjugation with glucuronic or sulfuric acid, are excreted in the urine as 17-ketosteroids. However, it should be noted that only 20% to 30% of the urinary 17-ketosteroids are derived from testosterone metabolism. The majority of the 17-ketosteroids are formed from the metabolism of adrenal steroids. Therefore, 17-ketosteroid determinations do not reliably reflect testicular steroid secretion.
Testosterone leaves the circulation and rapidly traverses the cell membrane (Figure 12–3). In most androgen target cells, testosterone is enzymatically converted to the more potent androgen dihydrotestosterone by the microsomal isoenzyme 5α-reductase-2, which has a pH optimum of 5.5. Another isoenzyme, 5α-reductase-1, has a pH optimum near 8.0 and may involve androgen action in the skin, but it is not active in the urogenital tract. Dihydrotestosterone as well as testosterone then binds to the same specific intracellular receptor protein (Rc in Figure 12–3) that is distinct from both androgen-binding protein and SHBG. The genes that encode for this protein are located on the X chromosome. The androgen receptor is a member of the steroid-thyroid hormone nuclear superfamily. It is synthesized in the cytoplasm and is associated with several heat shock proteins and immunophilins. When testosterone or dihydrotestosterone binds to the receptor, it dissociates from the multiprotein complex, and conformational changes take place that allow it to form a homodimer with another hormone-androgen receptor molecule and to be translocated into the nucleus through binding to importins (Rn in Figure 12–3). In the nucleus, the androgen–androgen receptor complex binds to androgen response elements in DNA through the DNA-binding domain of the receptor and interacts with protein coactivators, which allows the polymorphic transactivating domain of the receptor to initiate transcriptional activity. This results in the synthesis of messenger RNA (mRNA), which is eventually transported to the cytoplasm, where it directs new protein synthesis and other changes that together constitute androgen action. In addition to this classical genomic pathway of androgen action, which brings about action in hours to days, androgens may exhibit a rapid effect through nonclassical signaling pathways involving cell surface actions that alter cellular calcium influx.
A variety of biologic effects of androgens have been defined in males. As discussed in Chapter 14, they are essential for appropriate differentiation of the internal and external male genital system during fetal development. During puberty, androgen-mediated growth of the scrotum, epididymis, vas deferens, seminal vesicles, prostate, and penis occurs. The functional integrity of these organs requires androgens. Androgens stimulate skeletal muscle growth and growth of the larynx, which results in deepening of the voice, and of the epiphysial cartilaginous plates, which results in the pubertal growth spurt. Both ambisexual (pubic and axillary) hair growth and sexual (beard, mustache, chest, abdomen, and back) hair growth are stimulated, as is sebaceous gland activity. Other effects include stimulation of erythropoiesis and social behavioral changes.
The hypothalamus synthesizes a decapeptide, gonadotropin–releasing hormone (GnRH), and secretes it in pulses every 30 to 120 minutes into the hypothalamohypophysial portal blood. After reaching the anterior pituitary, GnRH binds to the gonadotrophs and stimulates the release of both luteinizing hormone (LH) and, to a lesser extent, FSH into the general circulation. LH is taken up by the Leydig cells, where it binds to specific membrane receptors. The LH receptor is a G protein–coupled receptor containing seven transmembrane domains with a serine- and threonine-rich cytoplasmic region containing a phosphorylation site and a 350- to 400 amino acid extracellular hormone-binding domain. The binding of LH to the receptor leads to activation of adenylyl cyclase and generation of cAMP and other messengers. It also stimulates the synthesis of a steroidogenic acute regulatory protein. This enhances the transfer of cholesterol into the inner mitochondrial membrane, leading to the synthesis and secretion of androgens. In turn, the elevation of androgens inhibits the secretion of LH from the anterior pituitary through a direct action on the pituitary and an inhibitory effect at the hypothalamic level. Both the hypothalamus and the pituitary have androgen and estrogen receptors. Experimentally, pure androgens such as dihydrotestosterone (DHT) reduce LH pulse frequency, whereas estradiol reduces LH pulse amplitude. However, the major inhibitory effect of androgen on the hypothalamus appears to be mediated principally by estradiol, which may be derived locally through the aromatization of testosterone. Leydig cells also secrete small quantities of cytokines and other peptides, which may be important for paracrine regulation of testicular function.
(See Figure 12–4)
After stimulation by GnRH, the gonadotrophs secrete FSH into the systemic circulation. This glycoprotein hormone binds to specific receptors in the Sertoli cells and stimulates the production of androgen-binding protein. FSH is necessary for the initiation of spermatogenesis. However, full maturation of the spermatozoa appears to require not only an FSH effect but also testosterone. Indeed, the major action of FSH on spermatogenesis may be via the stimulation of androgen-binding protein production, which allows a high intratubular concentration of testosterone to be maintained.
In addition to androgen-binding protein, the Sertoli cell secretes several other substances, including insulin-like growth factor-I, transferrin, Müllerian duct inhibitory factor, and inhibin. At least three genes have been found to direct inhibin synthesis. Although two forms of inhibin have been identified, only inhibin B has been found in males. This 32-kDa protein, which is composed of an alpha and a beta subunit, selectively inhibits FSH release from the pituitary without affecting LH release. FSH directly stimulates the Sertoli cells to secrete inhibin B. Serum inhibin B concentrations reflect Sertoli cell number and sperm production. They correlate with testicular size. There is a reciprocal relationship between serum inhibin B and FSH levels, and inhibin B is therefore probably a physiologic regulator of pituitary FSH secretion, possibly together with the gonadal steroids. Inhibin levels decline with advancing age.
Two additional inhibin-related proteins that have been identified in porcine follicular fluid may also be present in the testes. These factors, designated follicle regulatory protein and activin, are composed of inhibin beta subunit dimers and can selectively stimulate pituitary FSH secretion in vitro. They are structurally similar to transforming growth factor β (TGFβ), which can also stimulate pituitary FSH release. The physiologic role, if any, that follicle regulatory protein, activin, and TGFβ have in the regulation of FSH secretion is unknown.
Evaluation of Male Gonadal Function
The clinical presentation of patients with deficient testosterone production or action depends on the age at onset of hypogonadism. Androgen deficiency during the second to third months of fetal development results in varying degrees of ambiguity of the genitalia and male pseudohermaphroditism. If the deficiency develops during the third trimester, defects in testicular descent leading to cryptorchidism as well as micropenis may occur. These topics are covered in Chapters 14 and 15.
Prepubertal androgen deficiency leads to poor secondary sexual development and eunuchoid skeletal proportions. The penis fails to enlarge, the testes remain small, and the scrotum does not develop the marked rugae characteristic of puberty. The voice remains high-pitched and the muscle mass does not develop fully, resulting in less than normal strength and endurance. The lack of appropriate stimulation of sexual hair growth results in sparse axillary and pubic hair (which receive some stimulation from adrenal androgens) and absent or very sparse facial, chest, upper abdominal, and back hair. Although the androgen-mediated pubertal growth spurt fails to take place, the epiphysial plates of the long bones continue to grow under the influence of insulin-like growth factor-I and other growth factors. Thus, the long bones of the upper and lower extremities grow out of proportion to the axial skeleton. Healthy white men have an average upper segment (crown to pubis) to lower segment (pubis to floor) ratio of greater than 1, whereas prepubertal hypogonadism results in a ratio of less than 1. Similarly, the ratio of total arm span to total height averages 0.96 in white men. Because of the relatively greater growth in the upper extremities, the arm span of eunuchoid individuals exceeds height by 5 cm or more.
If testosterone deficiency develops after puberty, the patient may complain of decreased libido, erectile dysfunction, and low energy. Patients with mild androgen deficiency or androgen deficiency of recent onset may not note a decrease in facial or body hair growth; it appears that although adult androgen levels must be achieved to stimulate male sexual hair growth, relatively low levels of androgens are required to maintain sexual hair growth. With long-standing hypogonadism, the growth of facial hair will diminish, and the frequency of shaving may also decrease (Figure 12–5). In addition, fine wrinkles may appear in the corners of the mouth and eyes and, together with the sparse beard growth, result in the classic hypogonadal facies.
Adequate assessment of the genitalia is essential in the evaluation of male hypogonadism. The examination should be performed in a warm room in order to relax the dartos muscle of the scrotum. The penis should be examined for the presence of hypospadias, epispadias, and chordee (abnormal angulation of the penis due to a fibrotic plaque), which may interfere with fertility. The fully stretched dorsal penile length should be measured in the flaccid state from the pubopenile skin junction to the tip of the glans. The normal range in adults is 12 to 16 cm (10th and 90th percentiles, respectively).
Assessment of testicular volume is also vital to the evaluation of hypogonadism. Careful measurement of the longitudinal and transverse axes of the testes may be made and testicular volume (V) calculated from the formula for a prolate spheroid: V = 0.52 × length × width2. The mean volume for an adult testis is 18.6 ± 4.8 mL. Alternatively, volume may be estimated with the Prader orchidometer, which consists of a series of plastic ellipsoids ranging in volume from 1 to 25 mL. Each testis is compared with the appropriate ellipsoid. Adults normally have volumes greater than 15 mL by this method.
Because about half of testicular volume is composed of seminiferous tubules, decrease in volume indicates lack of tubular development or regression of tubular size. The consistency of the testicle should be noted. Small, firm testes are characteristic of hyalinization or fibrosis, as may occur in Klinefelter syndrome. Small, rubbery testes are normally found in prepubertal males; in an adult, they are indicative of deficient gonadotropin stimulation. Testes of soft consistency are characteristically found in individuals with postpubertal testicular atrophy.
The epididymis and vas deferens should also be examined. One of the most important parts of the examination is evaluation for the presence of varicocele resulting from incompetence of the internal spermatic vein. As will be discussed later, this is an important and potentially correctable cause of male infertility. The patient should be examined in the upright position while performing the Valsalva maneuver. The examiner should carefully palpate the spermatic cords above the testes. A varicocele can be felt as an impulse along the posterior portion of the cord. About 85% of varicoceles are located on the left side, and 15% are bilateral.
With some exceptions, a normal semen analysis excludes gonadal dysfunction. However, a single abnormal semen analysis is not a sufficient basis for a diagnosis of disturbance of testicular function, because marked variations in several of the parameters may be seen in normal individuals: at least three semen samples should be examined over a 2- to 3-month interval in order to evaluate this facet of male gonadal function. As noted earlier, approximately 3 months are required for completion of the spermatogenic cycle and movement of the mature spermatozoa through the ductal system. Therefore, when an abnormal semen sample is produced, one must question the patient about prior fever, trauma, drug exposure, and other factors that may temporarily damage spermatogenesis.
The semen should be collected by masturbation after 2 to 7 days of sexual abstinence and examined within 1 hour after collection. Normal semen has a volume of more than 2 to 4 mL, with 20 × 106 or more sperms per milliliter. Over half of the spermatozoa should exhibit progressive motility, and 30% or more should have normal morphology.
Each of the gonadal steroids may be measured by specific assays. Although single determinations may distinguish between normal individuals and patients with severe hypogonadism, mild defects in androgen production may be missed. In normal individuals, there are frequent, rapid pulsatile changes in serum testosterone concentration as well as a slight early morning elevation. Therefore, at least three separate blood samples should be collected at 20- to 40-minute intervals during the morning for testosterone measurement. The testosterone may be measured in each of the serum samples, or equal aliquots of each of the three serum samples may be combined, mixed, and subjected to testosterone analysis. The latter procedure provides a savings in cost as well as a mean serum testosterone concentration that takes into account the pulsatile release of testosterone.
Androgen and estrogen immunoassays measure total serum steroid concentrations. This is the sum of the free, biologically active hormone and the protein-bound moiety. There is a great deal of variability with different testosterone immunoassays, especially in the lower ranges. The most reliable methods are specific immunoassays following extraction of serum or gas chromatography (GC)- or liquid chromatography (LC)-tandem mass spectroscopy. Although in most circumstances it is not necessary to determine the actual quantity of free steroid hormones, in some situations alterations in the binding protein concentration may occur. Lowered concentrations of SHBG are seen in patients with hypothyroidism, obesity, and acromegaly. In these circumstances, the free testosterone concentration should be measured, because it may be normal when the total serum testosterone level is decreased. The normal male serum concentrations of gonadal steroids collected in the basal state are given in Table 12–2.
Hormone | Ranges |
---|---|
Testosterone, total | 260-1000 ng/dL (9.0-34.7 nmol/L) |
Testosterone, free | 50-210 pg/mL (173-729 pmol/L) |
Dihydrotestosterone | 27-75 ng/dL (0.9-2.6 nmol/L) |
Androstenedione | 50-250 ng/dL (1.7-8.5 nmol/L) |
Estradiol | 10-50 pg/mL (3.67-18.35 pmol/L) |
Estrone | 15-65 pg/mL (55.5-240 pmol/L) |
FSH | 1.6-8 mIU/mL (1.6 8 IU/L) |
LH | 1.5-9.3 mIU/mL (1. 5-9.3 IU/L) |
PRL | 2-18 ng/mL (87-780 nmol/L) |
LH and, to a lesser extent, FSH are released in pulsatile fashion throughout the day. In addition, there is a diurnal variation with higher levels in the morning than in the evening. Therefore, as with testosterone, at least three blood samples should be obtained at 20- to 40-minute intervals during the morning. FSH and LH may be measured in each of the samples or in a single pooled specimen. Although many laboratories give a numerical value for the lower limits of normal for gonadotropins, some normal males have undetectable concentrations of FSH and LH by presently available immunoassay techniques. Furthermore, the concentrations of gonadotropins measured in one laboratory may not be directly comparable to those measured in another because of differences in the reference preparations used. The primary use of basal FSH and LH concentrations is to distinguish between hypergonadotropic hypogonadism, in which either or both of the gonadotropins are elevated, and hypogonadotropic hypogonadism, in which the gonadotropins are low or inappropriately normal in the presence of decreased androgen production.
Elevations of serum prolactin (PRL) inhibit the normal release of pituitary gonadotropins (shown by a reduced LH pulse frequency), probably through an effect on the hypothalamus. Thus, serum PRL measurements should be performed in any patient with hypogonadotropic hypogonadism. Serum PRL concentrations are generally stable throughout the day; therefore, measurement of this hormone in a single sample is usually sufficient. However, the patient should abstain from eating for 3 hours before the blood sample is obtained, because a protein meal may acutely stimulate the release of PRL from the pituitary. The normal ranges for serum PRL and gonadotropins are shown in Table 12–2.
Human chorionic gonadotropin (hCG) is a glycoprotein hormone with biologic actions similar to those of LH. Following an injection of chorionic gonadotropin, this hormone binds to the LH receptors on the Leydig cells and stimulates the synthesis and secretion of testicular steroids. Therefore, the Leydig cells may be directly assessed by the intramuscular injection of 4000 IU of chorionic gonadotropin daily for 4 days. A normal response is a doubling of the testosterone level following the last injection. Alternatively, a single intramuscular dose of chorionic gonadotropin (5000 IU/1.7 m2 in adults or 100 IU/kg in children) may be given, with blood samples taken for testosterone measurements 72 and 96 hours later. Patients with primary gonadal disease have a diminished response following administration of chorionic gonadotropin, whereas patients with Leydig cell failure secondary to pituitary or hypothalamic disease have a qualitatively normal response.
Testicular biopsy in hypogonadal men is primarily indicated in patients with normal-sized testes and azoospermia in order to distinguish between spermatogenic failure and ductal obstruction. Although germinal aplasia, hypoplasia, maturation arrest, and other abnormalities of spermatogenesis may be diagnosed by examination of testicular tissue in oligospermic males, knowledge of the type of defect does not alter therapy. Therefore, testicular biopsy is not usually indicated for evaluation of mild to moderate oligospermia. If a testicular biopsy is carried out, testicular sperm extraction for possible intracytoplasmic sperm injection (ICSI) into ova may be performed at the same time.
Figure 12–6 outlines an approach to the diagnosis of male gonadal disorders. Semen analysis and determination of the basal concentrations of testosterone, FSH, and LH allow the clinician to distinguish patients with primary gonadal failure who have poor semen characteristics, low or normal testosterone, and elevated FSH or LH from those with secondary gonadal failure and abnormal semen analysis, decreased testosterone, and low or inappropriately normal gonadotropins.
In patients with elevations of gonadotropins resulting from primary testicular disease, chromosomal analysis helps to differentiate between genetic abnormalities and acquired testicular defects. Because no therapy exists that restores spermatogenesis in an individual with severe testicular damage, androgen replacement is the treatment of choice. Patients with isolated seminiferous tubule failure may have normal or elevated FSH concentrations in association with normal LH and testosterone levels and usually severe oligospermia. Patients with azoospermia require evaluation for the possible presence of ductal obstruction, because this defect may be surgically correctable. Fructose is added to seminal plasma by the seminal vesicles, and an absence of fructose indicates that the seminal vesicles are absent or bilaterally obstructed. The combination of a poor semen analysis with low testosterone, FSH, and LH is indicative of a hypothalamic or pituitary defect. Such patients need further evaluation of anterior and posterior pituitary gland function with appropriate pituitary function tests, as well as neuroradiologic and neuro-ophthalmologic studies (Chapter 4).
Pharmacology of Drugs Used to Treat Male Gonadal Disorders
A variety of drugs are available for the treatment of androgen deficiency. Preparations for sublingual or oral administration such as methyltestosterone, oxymetholone, and fluoxymesterone have the advantage of ease of administration but the disadvantage of erratic absorption, potential for cholestatic jaundice, and decreased effectiveness when compared to the intramuscular preparations. Testosterone propionate is a short-acting androgen. Its main use is in initiating therapy in older men, whose prostate glands may be exquisitely sensitive to testosterone. A dose of 50 mg two or three times per week is adequate. Obstructive symptoms due to benign prostatic hypertrophy following therapy with this androgen usually resolve rapidly because of its short duration of action.
Androgen deficiency may be treated with testosterone enanthate or cyclopentylpropionate (cypionate) given intramuscularly. Unlike the oral androgen preparations, both of these agents are capable of completely virilizing the patients. Therapy may be initiated with 50 mg intramuscularly every 4 weeks and gradually increased to a maintenance dose of 75 to 100 mg every week or 150 to 200 mg every 2 weeks. Testosterone undecanoate, a long-acting form of testosterone given intramuscularly every 3 months, is available in many countries, but is not yet approved by the FDA for use in the United States. Testosterone pellets may be implanted subcutaneously for a longer duration of effect. However, this therapy has not enjoyed much popularity. Transdermal delivery via membranes impregnated with testosterone (Androderm) is another method of replacement therapy. The patches can be placed on the skin of the back, shoulder, or abdomen and provide normal androgen concentrations. A testosterone gel (AndroGel 1%; Testim 1%) that is applied daily to the abdomen, shoulders, or upper arms also results in physiologic concentrations of testosterone. A buccal preparation (Striant) applied twice daily under the upper lip also is available, but is not widely used due to gum irritation.
Androgens, both oral and intramuscular, have been used (illegally) by some athletes to increase muscle mass and strength. Although this may achieve the anticipated result in some individuals, adverse effects include oligospermia and testicular atrophy—in addition to some of the complications noted below.
Androgen therapy is currently contraindicated in patients with prostatic carcinoma. About 1% to 2% of patients receiving oral methyltestosterone or fluoxymesterone develop intrahepatic cholestatic jaundice that resolves when the drug is discontinued. Rarely, these methylated or halogenated androgens have been associated with benign and malignant hepatocellular tumors.
Androgen therapy also may cause premature fusion of the epiphyses in an adolescent, and this may result in some loss of potential height. Therefore, androgen therapy is usually withheld until a hypogonadal male reaches 13 years of age. Sodium and water retention may induce hypertension or congestive heart failure in susceptible individuals. Because androgens stimulate erythropoietin production, erythrocytosis may occur during therapy. This is not usually clinically significant. Inhibition of spermatogenesis is mediated through suppression of gonadotropins by the androgens. Gynecomastia may develop during initiation of androgen therapy but usually resolves with continued administration of the drug. Sleep apnea may be precipitated. Priapism, acne, and aggressive behavior are dose-related adverse effects and generally disappear after reduction of dosage. Androgens decrease the production of thyroxine-binding globulin and corticosteroid-binding globulin by the liver. Therefore, total serum thyroxine and cortisol concentrations may be decreased, although the free hormone concentrations remain normal. High-density lipoprotein concentrations may also be reduced with oral androgens.
In patients with hypogonadism due to inadequate gonadotropin secretion, spermatogenesis and virilization may be induced by exogenous gonadotropin injections. Because the gonadotropins are proteins with short half-lives, they must be administered parenterally two or three times a week.
The expense and inconvenience of this type of therapy preclude its routine use for the treatment of androgen deficiency. The two major indications for exogenous gonadotropins are treatment of cryptorchidism (see later) and induction of spermatogenesis in hypogonadal males who wish to father children.
To induce spermatogenesis, 2000 IU of chorionic gonadotropin may be given intramuscularly three times a week for 9 to 12 months. In some individuals with partial gonadotropin deficiencies, this may induce adequate spermatogenesis. In patients with more severe deficiencies, menotropins, available in vials containing 75 IU each of FSH and LH, or highly purified urinary FSH (urofollitropin) or FSH produced by recombinant DNA technology (follitropin beta), each containing 75 IU of FSH, is added to chorionic gonadotropin therapy after 9 to 12 months and is administered in a dosage of one vial intramuscularly three times a week.
Adverse reactions with such therapy are minimal. Acne, gynecomastia, or prostatic enlargement may be noted as a result of excessive Leydig cell stimulation. Reduction of the chorionic gonadotropin dosage or a decrease in the frequency of chorionic gonadotropin injections generally results in resolution of the problem.
GnRH (gonadorelin acetate), administered in pulses every 60 to 120 minutes by portable infusion pumps, effectively stimulates the endogenous release of LH and FSH in hypogonadotropic hypogonadal patients. This therapy does not currently appear to offer any major advantage over the use of exogenous gonadotropins for induction of spermatogenesis or the use of testosterone enanthate or cypionate for virilization, and is no longer available in the United States. A long-acting analog of GnRH, leuprolide acetate, is available for the treatment of advanced prostatic carcinoma. Daily subcutaneous administration of 1 mg or monthly intramuscular injections of 7.5 mg of a depot preparation—22.5 mg for 3 months or 30 mg for 4 months—results in desensitization of the pituitary GnRH receptors, which reduces LH and FSH levels and so ultimately testosterone concentrations. Similar results are produced with a subcutaneous injection of the depot form of the GnRH analog goserelin or monthly intramuscular injections of triptorelin. With these therapies, initial remission rates for prostatic carcinoma are similar to those found with orchiectomy or treatment with diethylstilbestrol (about 70%). A long-acting GnRH antagonist, abarelix, also is an effective means of inducing medical castration by reducing LH, FSH, and testosterone secretion. Although GnRH agonists may produce an initial rise in LH, FSH, and testosterone levels, no such rise is seen with GnRH antagonists, making them better agents to use in the presence of metastasis in sensitive areas such as the spinal cord.
Nafarelin acetate is a GnRH agonist that is administered intranasally for the treatment of endometriosis and central precocious puberty. Central precocious puberty also may be treated with leuprolide acetate and another analog, histrelin acetate. Long-acting GnRH agonists also are used to suppress endogenous gonadal steroid production as part of the endocrine treatment of transsexual persons. Long-acting GnRH agonists combined with testosterone have been studied as a possible male contraceptive, but they do not uniformly induce azoospermia.
Hypogonadism may be subdivided into three general categories (Table 12–3). A thorough discussion of the hypothalamic–pituitary disorders that cause hypogonadism is presented in Chapters 4 and 15. The defects in androgen biosynthesis and androgen action are described in Chapter 14. The following section emphasizes the primary gonadal abnormalities.
Hypothalamic-Pituitary Disorders Panhypopituitarism Isolated LH deficiency (fertile eunuch) Isolated FSH deficiency LH and FSH deficiency a. With normal sense of smell b. With hyposmia or anosmia (Kallmann syndrome) c. With complex neurologic syndromes Prader-Willi syndrome Laurence-Moon, Bardet-Biedl syndromes Möbius syndrome Lowe syndrome Cerebellar ataxia Biologically inactive LH Hyperprolactinemia |
Gonadal Abnormalities Klinefelter syndrome Other chromosomal defects (XX male, XY/XXY, XX/XXY, XXXY, XXXXY, XXYY, XYY) Bilateral anorchia (vanishing testes syndrome) Leydig cell aplasia Cryptorchidism Noonan syndrome Myotonic dystrophy Adult seminiferous tubule failure Adult Leydig cell failure Defects in androgen biosynthesis |
Defects in Androgen Action Complete androgen insensitivity (testicular feminization) Incomplete androgen insensitivity |
Klinefelter syndrome is the most common genetic cause of male hypogonadism, occurring in one of 600 male births. An extra X chromosome is present in about 0.2% of male conceptions and 0.1% to 0.2% of live-born males. Sex chromosome surveys of mentally retarded males have revealed an extra X chromosome in 0.45% to 2.5% of such individuals. Patients with an XXY genotype have classic Klinefelter syndrome; those with an XXXY, XXXXY, or XXYY genotype or with XXY/chromosomal mosaicism are considered to have variant forms of the syndrome.
The XXY genotype is usually due to meiotic nondisjunction during parental gametogenesis, which results in an egg with two X chromosomes or a sperm with an X and a Y chromosome. After fertilization, nondisjunction during mitotic division results in mosaicism.
At birth there are generally no physical stigmas of Klinefelter syndrome, and during childhood there are no specific signs or symptoms. The chromosomal defect is expressed chiefly during puberty. As the gonadotropins increase, the seminiferous tubules do not enlarge but rather undergo fibrosis and hyalinization, which results in small, firm testes. Obliteration of the seminiferous tubules results in azoospermia.
In addition to dysgenesis of the seminiferous tubules, the Leydig cells are also abnormal. They are present in clumps and appear to be hyperplastic on initial examination of a testicular biopsy. However, the Leydig cell mass is not increased, and the apparent hyperplasia is actually due to the marked reduction in tubular volume. Despite the normal mass of tissue, the Leydig cells are functionally abnormal. The testosterone production rate is reduced, and there is a compensatory elevation in serum LH. Stimulation of the Leydig cells with exogenous chorionic gonadotropin results in a subnormal rise in testosterone. The clinical manifestations of androgen deficiency vary considerably from patient to patient and may be related to the degree of diminished testosterone production and alterations in the androgen receptor. Exon 1 of the androgen receptor gene contains a polymorphism with a variable number of CAG repeats. As the number of repeats increases, there is a progressive decrease in androgen action and more phenotypic abnormalities. Thus, some individuals have virtually no secondary sexual developmental changes, whereas others are indistinguishable from healthy individuals.
The elevated LH concentrations also stimulate the Leydig cells to secrete increased quantities of estradiol and estradiol precursors. The relatively high estradiol: testosterone ratio is responsible for the variable degrees of feminization and gynecomastia seen in these patients. The elevated estradiol also stimulates the liver to produce SHBG. This may result in total serum testosterone concentrations that are within the low-normal range for adult males. However, the free testosterone level may be lower than normal.
The pathogenesis of the eunuchoid proportions, personality, and intellectual deficits and associated medical disorders is presently unclear.
Most of the seminiferous tubules are fibrotic and hyalinized, although occasional Sertoli cells and spermatogonia may be present in some sections. Absence of elastic fibers in the tunica propria is indicative of the dysgenetic nature of the tubules. The Leydig cells are arranged in clumps and appear hyperplastic, although the total mass is normal.
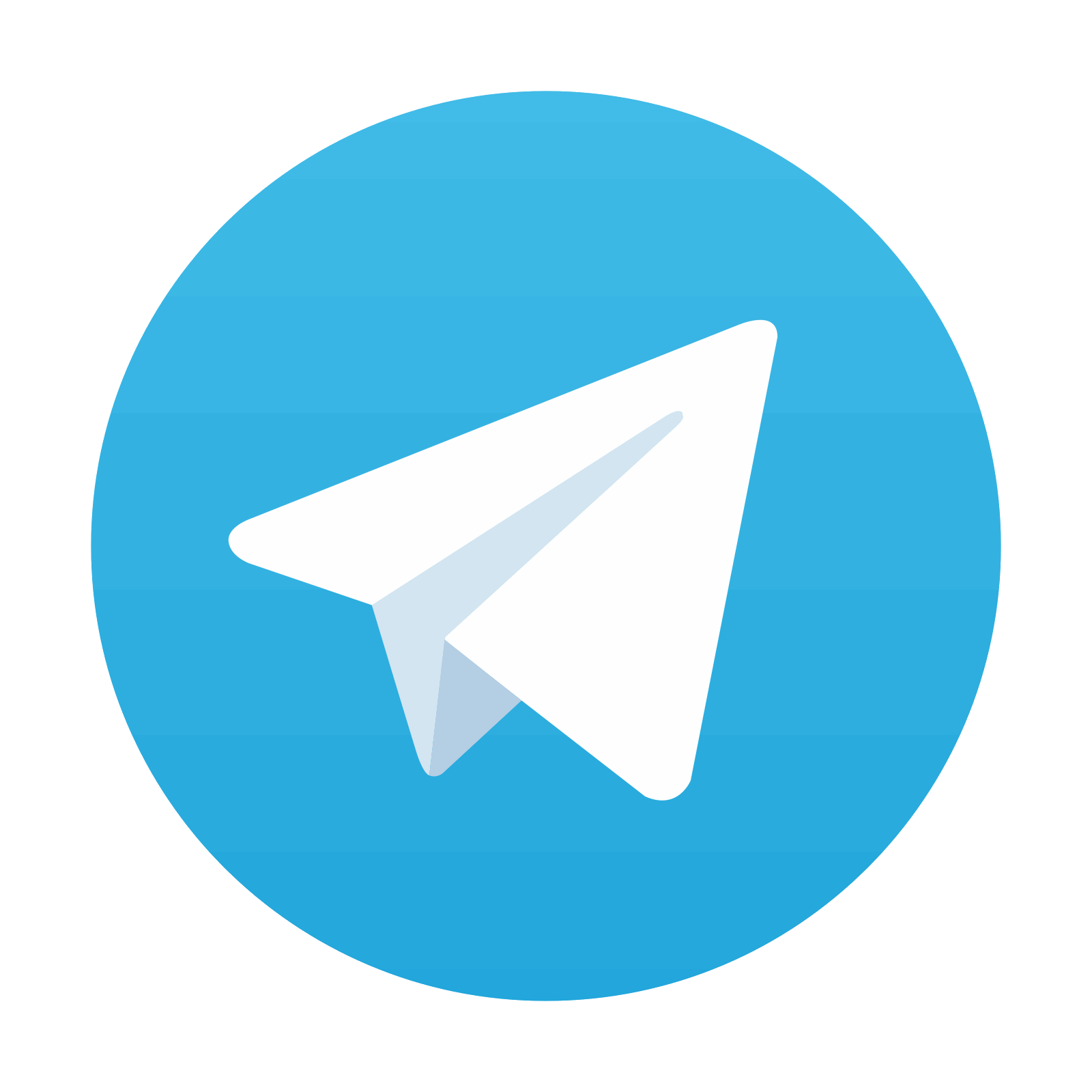
Stay updated, free articles. Join our Telegram channel

Full access? Get Clinical Tree
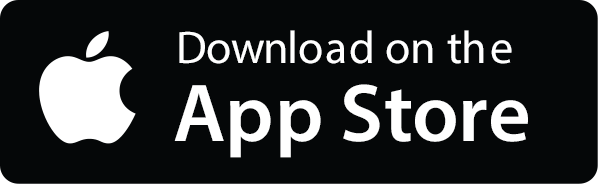
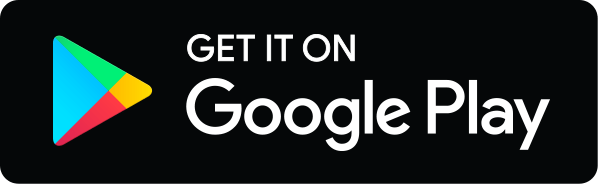