Fig. 19.1
Motor fMRI. A low grade glioma was located in the right superior frontal gyrus, anteriorly to the precentral sulcus. Presurgical fMRI shows primary sensorimotor activation of the left fingers in red, located along the precentral gyrus, posteriorly to the tumor (a–c). Left toes movements in green elicited activation in the paracentral lobule, posteriorly and medially to the tumor (c). SMA, adjacent to the medial aspect of the tumor was activated with an anteroposterior distribution, the activation related to hand movement was located anteriorly to those of the foot movements. A complete tumoral resection was performed, including SMA, and with respect to precentral gyrus. After surgery, a SMA syndrome was observed with a transient motor impairment of the left hemibody that recovered in a week
In addition to primary sensorimotor cortex, secondary motor cortex involved in motor planning and motor onset such as the supplementary motor area (SMA) and the lateral premotor area are usually identified using simple paced movements. These activities are rather bilateral with predominance in the areas contralateral to the movements. In the SMA, a rostro-caudal somatotopy is also reported with representation the lips close to the vertical line passing through the anterior commissure and orthogonal to anterior commissure—posterior commissure plane. Foot representation is located posteriorly, adjacent to anterior aspect of the paracentral gyrus. Hand representation is located in between lips and foot representations [18] (Fig. 19.1). Again, conditional subtraction using mental imagery, modulation of movement preparation or complexity, could help to better distinguish primary from secondary motor areas. However, compromises are advocated to maximize fMRI feasibility in clinical practice.
These data were validated using several techniques. Similar results were obtained when compared to others techniques such as PET [19, 20] and magnetoencephalography [21, 22]. Confrontation with intraoperative electrical cortical stimulations demonstrated an accuracy of fMRI data within a 10–15 mm range [23, 24]. These slight differences are mainly explained by the sensory involvement using simple tasks contrasting paced movements versus rest, and the venous contribution of the BOLD signal. Additional validation was also obtained from surgical resection. Indeed, the resection of the SMA activation was followed by a transient contralateral motor deficit that recovers within several days, the so-called SMA syndrome [25, 26].
While acute lesions of the primary sensorimotor areas are at risk of permanent neurological deficit, slow growing low grade gliomas are prone to elicit loco-regional plasticity, involving adjacent areas and homologous contralesional areas, especially lateral premotor area and SMA able to facilitate recovery [26]. Considering rigorous quality check, well-known methodological and technical limitations of BOLD fMRI, cortical mapping of primary sensorimotor areas and SMA provided by BOLD fMRI may be helpful for treatment strategy and presurgical planning [27–29].
19.2.4.2 Language System
Lesion-based and electrical stimulation mapping (ESM) studies demonstrated that the language system relies on a widespread cortico-subcortical network connected by subcortical fibers bundles.
The classical model inherited from the nineteenth century emphasized on an anterior motor area (Broca’s area including posterior part of the inferior frontal gyrus and anterior opercular area) and a posterior sensitive area (Wernicke’s area including posterior part of the superior temporal gyrus and posterior opercular area), connected by the arcuate fasciculus. This organization has a strong left hemispheric dominance in the general population. Even though his simple model can explain some cases in clinical practice, fMRI may show additional area, such as the preSMA, especially using production task (Fig. 19.2). Indeed, surgical resection of activation located in the preSMA in the dominant hemisphere is associated with the occurrence of transient mutism [30].


Fig. 19.2
Language fMRI: Plasticity and reproducibility. A low-grade glioma of the left middle frontal gyrus was revealed by seizure with speech impairment in a right-handed patient. Language fMRI using a covert sentence generation task repeated twice (activation maps using same statistical threshold are displayed in red (1st task), in green (2nd task); in yellow (overlap) shows reproducible anterior activations in both inferior frontal gyri (a, b), both middle frontal gyri (c–e), preSMA (d, e). Reproducible posterior activation was detected in the posterior part of the right superior temporal sulcus (a). The examination shows a right-hemisphere predominance for language with an activation of the left middle frontal gyrus close to the posterior-inferior aspect of the tumor. A complete tumoral resection was obtained with awake surgery and intraoperative electrostimulation mapping. No speech impairment was reported during and after surgery
The reliability of BOLD fMRI to determine hemispheric dominance, based on the calculation of a laterality index [31], was estimated by comparison with the selective intracarotid injection of sodium amobarbital, the WADA test that temporarily disables the hemisphere downstream [24, 32, 33]. This agreement argued to prone fMRI as a non-invasive technique able to determine hemispheric dominance for language instead of WADA test because of its greater comfort, lack of morbidity, lower cost, and higher availability.
In fact, ESM and numerous observations suggested that language organization was more complex, advocating for the necessity to update the classical model. Indeed, a dual stream of language is now proposed [34]. Inspired by the dual stream processing in the vision system, language system would rely on a dorsal stream for sensorimotor integration in the dominant hemisphere, and on a ventral stream for speech comprehension rather bilateral. Both streams would process neural inputs from sensory integration given by the Wernicke’s area. The ventral stream would involve the ventral and anterior parts of both temporal lobes to perform speech recognition and representation of lexical concepts. The dorsal stream would consist in two pathways involved in driving phonological information to articulatory motor representations. The posterior part of the inferior and middle frontal gyri and the perisylvian temporo-parietal area would have a hemispheric predominance, left-sided in most cases [34].
Such a broader distribution of the language network is also supported by fMRI that provides multiple activation foci. In clinical practice, a set of block-designed paradigms is recommended to better identify anterior and posterior activation. This set may consist in sentence completion, word generation, speech comprehension [29, 30, 35, 36]. However, numerous discrepancies with ESM were reported [34, 37–39]. ESM remains the gold standard method using various tasks such as spontaneous speech, picture naming, and counting. As an alternative method, language fMRI mapping was obtained using several tasks involving speech production and comprehension. Language fMRI sensitivity ranged from 37 to 100% and specificity from 60 to 100%. fMRI false-negative rate, that decreased sensitivity and may lead to permanent deficit, would rather be associated with high-grade gliomas in line with data collected from motor tasks [40]. fMRI false-positive rate, that decreased specificity and may lead to an incomplete resection, would rather be associated with low-grade gliomas [38]. These differences might be explained by differences between covert tasks commonly used in fMRI to avoid head movement and overt tasks used in ESM, and by difference in spatial selectivity and functional sensitivity of each technique.
As a consequence of these considerations, fMRI results in language preoperative mapping remain questionable regarding presurgical goals with the needs to provide exhaustive mapping of critical areas, including spatial delineation with tumoral margins. Indeed, the variability of the fMRI maps remains significant despite of methodological attempts of standardization, cortical mapping does not provide information of essential subcortical structures to be preserved during surgery, fMRI is hardly able to distinguish critical areas from less essential areas regarding functional prognosis and oncological achievement.
In practice, fMRI of language system remains often limited to determine hemispheric dominance based on the sums of left and right-sided clusters that allow to calculate the laterality index, a way use to match the binary representation of language based on WADA test. This simplification barely reflects the multiple cortical involvement detected by fMRI using various tasks. Despite limitations, confrontations between fMRI and ESM will provide further insights in language processing.
19.2.5 Key Points in fMRI Interpretation
Despite recent works using advanced imaging techniques; numerous limitations maintain BOLD fMRI far from being able to map directly neural activity in clinical practice. Besides reserves on the physiological basis of the BOLD signal and the cognitive subtraction approach, imaging resolutions are far out of the neural scales. Indeed, a standard voxel of 55 mm3 (3–5 mm in each dimension) contains 5.5 million neurons, 2.2–5.5 × 1010 synapses, 22 km of dendrites, and 220 km of axons Additionally, a spatial smoothing twice larger than the voxel size is commonly applied [2]. The temporal resolution of 2–3 s is also far from having the neural millisecond scale, and multiple repetitions must be performed and averaged over time to reach a sufficient contrast between cognitive conditions.
Before addressing the most common issues in clinical fMRI interpretation, expected results and potential methodological and physiological confounds should be clear in mind. The whole procedure of data acquisition and analysis must be tested and validated prior to start clinical practice.
19.2.5.1 Image Quality
Functional MRI is an imaging technique. Checking image quality sounds trivial. Contrary to conventional MRI, checking for the quality of the whole dataset is hard to perform in clinical practice. Indeed, a standard fMRI examination may, for instance, include 4 tasks acquired in 4 separate sessions of 100 volumes containing 40 slices, giving a total of 16,000 images to screen for artifacts.
Magnetic susceptibility artifacts mostly due to hemorrhage, calcifications, metal, and previous surgical procedures are common in neuro-oncology [41]. Beside these lesion-related artifacts, constitutional magnetic susceptibility artifacts due to important signal changes between air, bone, and tissue are detected near the skull base. Thus, fMRI of orbito-frontal, temporo-polar, and temporo-basal regions is difficult to perform using GRE-T2*. Such artifacts could be reduced using spin-echo T2-weighted images [42].
When present, these artifacts might be responsible of both false negative and false positive results. On the one hand, no reliable BOLD signal changes related to the activation paradigm can be extracted within a steady and homogeneous “black hole”. On the other hand, false activations are commonly observed on the margins of these artifacts due to subtle movements synchronous to the paradigm.
Recent advances in MR processing with real-time fMRI packages offer online reconstruction, to display native images and to superimpose activation maps. In such situation, it provides a better comprehension of the results during the examination, instead of suggesting another cause of abnormal results such as abnormal performance or movement that would require performing the task again.
19.2.5.2 Image Position
In clinical practice, statistical maps calculated using BOLD images are usually overlaid onto an anatomical image to better depict the spatial relationships of the activations with the surrounding sulci or a lesion. To be valid, the superimposition is performed between datasets with similar geometric parameters or using software able to interpret potential change of position, angle and dimensions across volumes, appropriately. Coregistration can be easily checked by superimposing BOLD native images onto the anatomical image. Right-left orientation has also to be carefully checked. When necessary, manual coregistration must be conducted. Despite extensive care for spatial coregistration, a brain shift might occur during surgery and the removal of a space-occupying lesion. Thus, intraoperative fMRI has been proposed in addition to shift simulation implemented within the navigation system [43].
19.2.5.3 Individual Performance
As other functional neuroimaging techniques, poor performance is a trivial cause of poor results. Indeed, the assessment of task-related neural activity relies on the appropriate perception of the stimuli and the execution of the task when required. The attention has also to be controlled across conditions and sessions. Indeed attention and fatigue modulate BOLD contrast [44]. Overly long paradigm should be avoided. In patients, experimental setup and design must be simple and feasible, limiting fatigue, task difficulty and speed. Thus, preliminary tests are necessary to estimate the appropriateness and the feasibility of the tasks.
Before fMRI, a full explanation of the tasks and of the fMRI time course must be given and sufficient training must be performed. During the examination, instructions ought to be re-explained and repeated before each run. A dedicated software is useful to send stimuli at specific onsets. Tasks execution should be monitored, at least visually for motor tasks. However, changes in force, amplitude, frequency, or preparation of the movement influence the results. In cognitive tasks, behavioral data can be recorded, using dedicated device to obtain specific answers. To study the hemispheric dominance for language, production tasks are robust and simple. Covert tasks are usually conducted because overt paradigms increase task-related movements and their control “non-language” condition is difficult to perform overtly. However, monitoring a covert task is particularly difficult. Again, real-time analyses are useful to estimate results, to repeat a task when necessary, and for positive reinforcement. To better estimate the relationship between the task execution and signal changes, recorded performances can be tested as a statistical regressor.
19.2.5.4 Movements
Because of the brief duration of each image acquisition (<100 ms), movements artifacts within the image are unlikely. However in awake subjects, subtle movements are always identified across images along time. The motion amplitude and its spatial coherence and synchrony with the paradigm may be responsible of significant signal changes [45]. In case of movements, most important signal changes are commonly detected along the parenchymal borders in voxels containing both brain tissue and cerebrospinal fluid. When these changes are synchronous with the task execution, long strips of false-positive activation are detected along the margins of the brain and ventricles. The borders of foci of magnetic susceptibility, such as hemorrhage or calcification with voxels containing both deep hypointensity and parenchymal hyperintensity, might also be associated with peripheral false-positive activation.
To minimize motion, subject must feel comfortable with the head maintained within the coil by adapted cushions. Paradigms must be tested to avoid excessive task-related movements and the overall duration of fMRI must be limited. Again, preliminary evaluation, explanation, training, dialog, and real-time analyses are precious to avoid excessive motion. After image acquisition, motion correction could be performed using spatial realignment. Statistical analyses might also estimate the confounding effect of head motion.
19.2.5.5 Physiological Confounds
As previously mentioned, BOLD contrast relies on task-related changes in neural activity, perfusion, and blood oxygenation. This complex mechanism is modulated by basal conditions and physiological properties such as resting neural activity, oxygenation, neurovascular coupling, perfusion, and vasomotricity. Age, medication, pathology, capnia, nicotine or caffeine influence BOLD contrast [1, 5, 15, 46, 47]. These confounds need to be controlled in comparative studies across populations. Individually, brain lesions may influence locally BOLD contrast and may lead to inappropriate interpretation [1, 40, 48–57]. For instance, the vicinity of a tumor decreases adjacent activation even for a distance greater than 10 mm [40, 51, 53, 54, 57]. In preoperative fMRI, BOLD signal is impaired in patients with higher grade gliomas and meningiomas [40, 48, 50, 53, 54, 56]. However, inconstant impairment was reported close to low grade gliomas [40, 58].
As BOLD contrast impairment might underestimate the local neural activity, discordance in language lateralization has been reported when compared to the Wada test [44, 55, 57]. In fact, interhemispheric comparisons are flawed when unilateral lesion or vascular impairment is detected. In such cases, BOLD contrast should be at least tested [40, 52, 59, 60].
Besides oxygenation disorders near brain lesions, impaired vasomotor responses have been previously proposed to explain BOLD discrepancies [46, 47]. Schematically, pathophysiological alterations might be secondary to changes in: (1) the functional mechanisms that link a specific stimulus and a vasomotor response (neurovascular coupling in response to neural activity, vasoreactivity to circulating gases, and autoregulation to perfusion pressure); and (2) the quality of the hemodynamic responses that might be affected by loco-regional changes in basal perfusion and structural abnormalities of the vasculature.
A hemodynamic hypothesis would be supported by regional perfusion changes either due to the lesional vascularization, or to structural alterations of the surrounding brain vessels. In line with experimental data in healthy subjects showing that BOLD signal may decrease as CBV increases [5], local changes in basal perfusion have been advocated to explain BOLD contrast variations in patients with tumor [40, 51, 54, 58].
In brain-lesioned patients, quantitative fMRI using the calibrated-BOLD method seems to be a promising technique to better estimate the underlying neural activity. However, this method remains difficult to conduct in clinical practice. An alternative could be to estimate the quality of the BOLD contrast using hypercapnic challenge [40, 61]. Indeed, imaging of cerebral vascular reactivity (CVR) to CO2 change using BOLD signal has been tested, especially in patients with vascular disorders and false negative fMRI results [40, 60]. This approach provides BOLD maps that overlap 95% of the functional activation. In patients with stroke and tumors, regional asymmetries in eloquent areas detected on CVR maps were the best predictors for impaired motor activation [40, 52].
As BOLD fMRI aggregates evoked blood oxygenation and functional changes in brain perfusion, fMRI interpretation might remain difficult, especially in case of focal lesions that modify these parameters. Multimodal advanced imaging, including DTI to better detect peritumoral infiltration and edema, perfusion study with permeability and vessel size imaging, functional imaging of the perfusion using arterial spin labeling during vasomotor challenge, oxygenation imaging using MRI or NIRS, could be proposed to better interpret fMRI data in patients, and to better understand structural and functional changes near brain lesions.
19.2.6 Conclusions on Task-Based fMRI
BOLD fMRI is regularly performed in clinical practice to map cortical activity of functional areas at risk of deficit before surgical resection of low grade gliomas. This technique relies on a solid biophysical framework. However, fMRI provides indirect evidence of cortical activity. Indeed, BOLD signals reveals tasks-related changes in oxygenation and perfusion. Interpretation must consider several constraints. As any imaging technique, the quality of native images must be sufficient to detect signal changes. Artifacts are common close to the skull base in the frontal and temporal regions, and in case of calcification and hemorrhage before and after surgery of brain tumors. The spatial and temporal resolutions of BOLD fMRI is far out of the range of neural scales. Activation foci with a centimetric interval of confidence should be considered. Repetition of simple tasks with a conditional approach is mandatory to reach significant and reliable signal changes, limiting fMRI to a non-ecologic investigation of brain activity. As any functional imaging technique, stimuli perception and tasks execution must be monitored. Real-time fMRI is helpful to provide immediate maps and to estimate overall quality check. Finally, changes in basal perfusion and in perfusion properties should be identified especially when false negative results are suspected close to the lesion. Considering rigorous quality check, well-known methodological and technical limitations, BOLD fMRI may remain useful for treatment strategy and presurgical planning.
19.3 Resting-State fMRI in DLGG
19.3.1 An Introduction to Resting-State fMRI
As mentioned above, most of functional neuroimaging studies focus on brain’s response to a task or stimulus. However, the majority of brain’s energy consumption arises from its activity at rest (95%) [62]. Spontaneous BOLD signal low frequency fluctuations are thought to arise from that baseline activity. Resting-state fMRI (RS-fMRI) focuses on spontaneous low frequency fluctuations (about 0.1 Hz) in the BOLD signal. An analysis of the frequency spectrum of RS-fMRI data demonstrated that low frequency fluctuations (defined as <0.1 Hz) contributed to more than 90% of the correlation coefficient between regions of the same resting-state network [63].
The functional significance of these fluctuations was first presented by Biswal et al. [64]. In this study, subjects were told not to perform any task. The authors identified a seed region in the left somatosensory cortex on the basis of block design fMRI. After determining the correlation between the BOLD time course of the seed region and that of all other areas in the brain, the authors found that the left somatosensory cortex was highly correlated with homologous areas in the contralateral hemisphere. The existence of synchronous spontaneous fluctuations between primary somatosensory and higher order areas was further confirmed by later studies [65, 66].
Several distinct networks have been identified at rest using BOLD signal temporal correlations defined as resting state networks (RSN). One of the most consistent RSN is the default mode network (DMN) [67], first identified from positron emission tomography (PET) data by Raichle et al. In this study, the authors found that consistent regions of the brain were activated at rest but decreased their activity when cognitive tasks were performed. Greicius et al. [68] identified the DMN using fMRI. Further studies have hypothesized that there are two opposing systems in the brain, one including the DMN and the other composed of attentional or task-based systems [69]. Those two systems have an anti-correlated functional connectivity.
Several other RSNs have been identified. The somatosensory network, studied first by Biswal et al., [64] includes primary and higher order motor as well as sensory areas. The visual network is highly consistent across various studies and spans much of the occipital cortex [63, 70]. An auditory network consisting of the Heschl gyrus, the superior temporal gyrus, and the posterior insula has been identified [66]. A language network that includes the so-called Broca’s area and Wernicke’s area, but also that extends to prefrontal, temporal, parietal, and subcortical regions, has been described by using RS-fMRI [71].
RSNs involved in attentional modulation and cognitive control have also been identified. Two networks identified by using both RS-fMRI and task-based fMRI include the dorsal and ventral attention networks [72]. The dorsal attention network includes the intraparietal sulcus and the frontal eye field and is involved in the executive control of attention. The ventral attention network, which includes the temporoparietal junction and ventral frontal cortex, is involved in the detection of salient cues (those that stand out from their environment) [72]. The frontoparietal control network, which includes the lateral prefrontal cortex and the inferior parietal lobule, is thought to be involved in decision-making processes [73].
19.3.2 Reliability of RS-fMRI
FMRI appears to be the ideal neuroimaging technique for the investigation of resting-state network characteristics. The spatial resolution is superior to other methodologies such as electroencephalography and magnetoencephalography, allowing for localization and separation of the various resting-state networks simultaneously. Significant correlations between variations in the power of electrophysiological activity in higher frequency bands (e.g. alpha and beta) and RS-fMRI signals have been demonstrated [74].
One weakness of RS-fMRI lies in an important difference between the analysis of spontaneous fluctuations and more traditional studies of task-evoked BOLD responses. In the latter, the timing and intensity of the task is known a priori and the responses of many trials are combined together to eliminate noise and to increase statistical significance [4, 75]. However, in RS-fMRI, functional connectivity is determined by measuring the temporal similarity of the BOLD time series in voxels using some metric, commonly the correlation coefficient. For example, in the original Biswal paper [64], voxels whose correlation coefficient passed a statistical threshold were deemed to be functionally connected, thus revealing common spontaneous fluctuations between left and right motor cortices. Since the two time series are measured simultaneously, any non-neural activity-related process that affects one or both time series will affect the measure of functional connectivity, thus yielding a spurious result. These RS-fMRI confounds can not only increase the apparent functional connectivity by introducing spurious similarities between the time series but they can also reduce the connectivity metric if differential confounds between regions are introduced. RS-fMRI confounds encompass the following physiological parameters: motion, cardiac and respiratory physiological noise, arterial CO2 concentration, blood pressure, cerebral autoregulation and vasomotion [76]. In order to control those physiological confounds one can use two different RS-fMRI cleanup techniques: those utilizing external recordings of physiology and data-based cleanup methods that only use the RS-fMRI data itself [76].
Important issues to consider with regard to RS-fMRI are the test-retest reproducibility and inter-subject variability. Studies suggest that RSNs can be detected reliably across imaging sessions [77] and across different subjects [78] though there may be some loci of variability between subjects [79].
19.3.3 Functional Connectivity Maps: Technical Aspects
The simplest technique is to extract the BOLD time course from a region of interest (called a seed region) and determine the temporal correlation between this extracted signal and the time course from all other brain voxels. This approach is widely used owing to its inherent simplicity, sensitivity and ease of interpretation [64]. However, it has some disadvantages. The results are dependent on the a priori definition of a seed region, multiple systems cannot be studied simultaneously and the extracted waveform may not be a true independent variable when assessing statistical significance. In response to these limitations other more sophisticated techniques for analyzing spontaneous BOLD data have been proposed.
Hierarchical clustering still requires a priori definition of seed regions [80]. However, instead of extracting the time course from just one seed region, the time courses from many seed regions are obtained and a correlation matrix is constructed. A clustering algorithm is then used to determine which regions are most closely related and which regions are more distantly related. This technique is useful to visualize the interaction between a large number of regions.
Independent component analysis (ICA) is perhaps the second most popular technique for analyzing spontaneous BOLD data [65]. This approach does not require a priori definition of seed regions. Instead, sophisticated algorithms analyze the entire BOLD data set and decompose it into components that are maximally independent in a statistical sense. Each component is associated with a spatial map. Some maps reflect noise components whereas others reflect neuro-anatomical systems. Because this technique is data driven and automatically isolates sources of noise, it holds tremendous promise and its use is increasing. However, there are still several challenges. First, results are highly dependent on the number of components one asks the algorithm to produce. Second, the user must determine which components reflect noise and which components look like neuro-anatomical systems, introducing a priori criteria for system selection.
19.3.4 Resting State fMRI and Low Grade Glioma Surgical Planning
The use of resting state fMRI for the evaluation of spontaneous fluctuations in the BOLD signal has several benefits over traditional task-based fMRI. One important advantage of this method is that it can be performed even when the patient is unable to cooperate with the functional task. This will enable us to perform fMRI mapping on many populations previously excluded from traditional task-based techniques such as young children, patients with cognitive impairments, and patients that are paralyzed, aphasic, or hard of hearing. Spontaneous fluctuations have been shown to persist under conditions of sleep [81] and different levels of anesthesia [82], thus a second advantage of this technique is that is can be performed in agitated patients and in young children under sedation. A third advantage is that one data acquisition can be used to study many different brain networks, thus possibly reducing the acquisition time when many systems are evaluated. This is in contrast to task activations which require dedicated data acquisitions for each function one is attempting to localize.
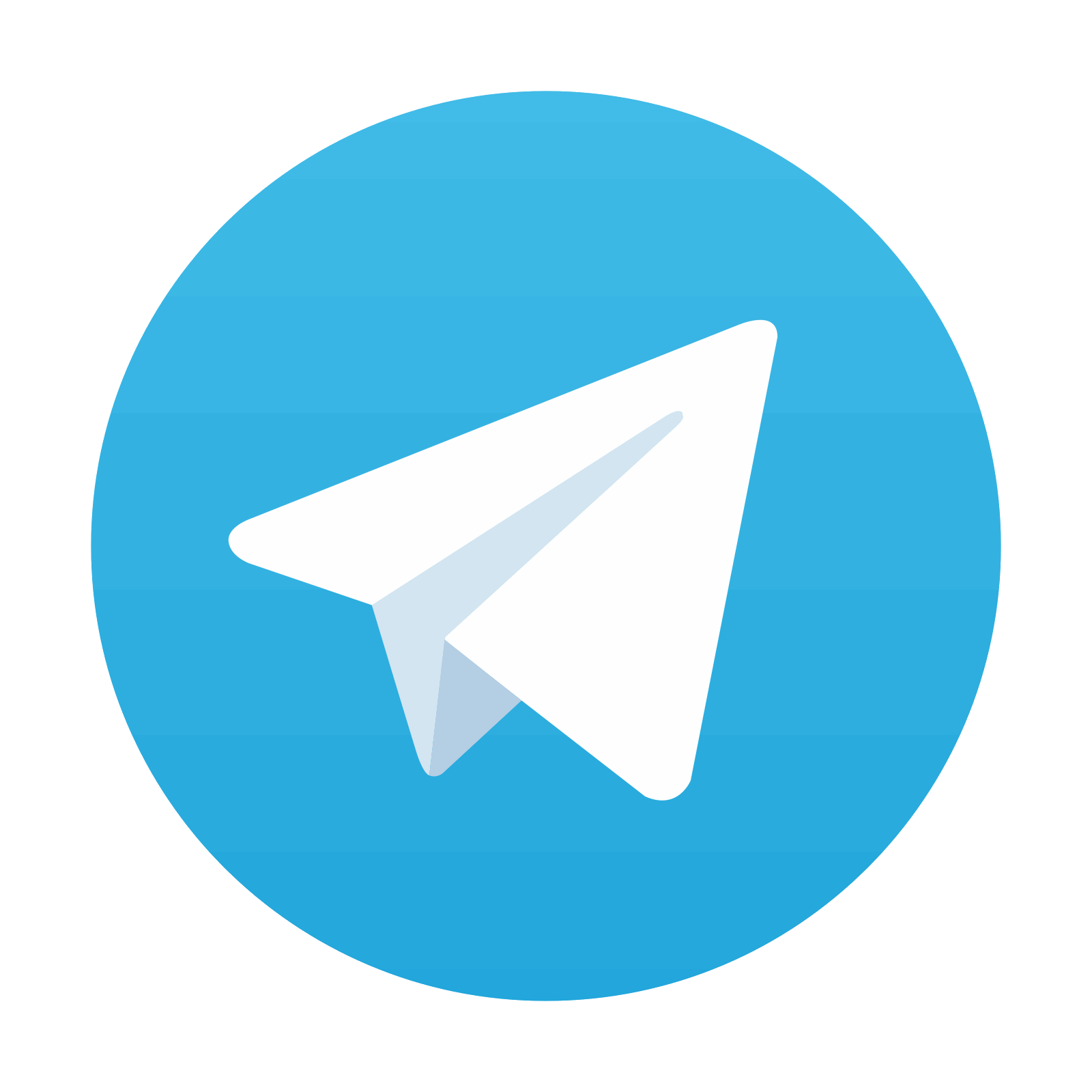
Stay updated, free articles. Join our Telegram channel

Full access? Get Clinical Tree
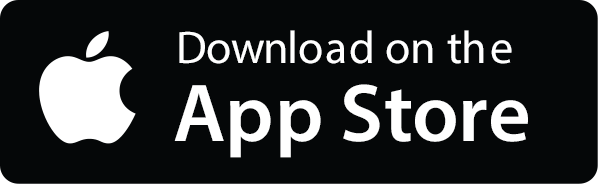
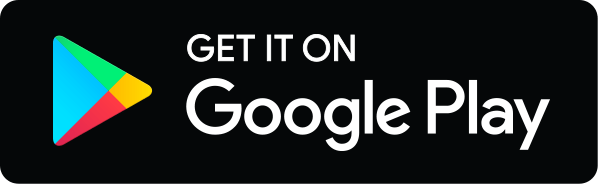