Keywords
Targeted anti-angiogenic gene therapy, nonviral vector, viral vector, tumor-specific promoter, antibody conjugation, peptide conjugation, adeno-associated virus phage, tumor necrosis factor-α
Introduction
The microenvironment in which a tumor originates plays a critical role in its initiation and progression. In tissues experiencing tumor growth, the stroma and stromal cells, including fibroblasts and vascular endothelial cells, create a supportive microenvironment for tumor growth, metastasis, and angiogenesis. Tumor angiogenesis is defined as the proliferation of a network of blood vessels used to supply the tumor with oxygen and nutrients. This area presents an exciting and innovative target for novel therapies, which may overcome the limitations of current modalities. The molecular and cellular aberrations that lead to the upregulation of angiogenesis associated with tumor growth present a unique target for the utilization of gene therapy through the direct delivery of antivascular agents to the endothelial cells of the tumor vasculature . This presents an attractive area to target for gene therapy, which has resulted in the revitalization of efforts to develop a novel vascular-targeting agent, as well as improve the specific delivery modalities that can be used to achieve this goal. This chapter reviews the current status of targeted cancer gene therapy and outlines the challenges for the next generation of gene transfer technology. Furthermore, the chapter summarizes the results of preclinical anti-angiogenic gene therapy investigations, which utilize targeted tumor necrosis factor-α (TNF-α) treatment, and discusses potential clinical applications of this treatment strategy.
Angiogenesis and its Role in Tumor Biology
Angiogenesis is the development of new capillary growth from a previously established vasculature. Several studies performed in the early 1900s noted abnormalities in the tumor-associated blood vessels, which further suggested that tumors might produce substances that promote blood vessel growth and permeability . The isolation of such substances by Folkman was followed by the discovery of numerous tumor-derived pro-angiogenic cytokines, including the vascular endothelial growth factor (VEGF) and fibroblast growth factor (FGF) families . A key advancement in the practicability of anti-angiogenic therapy as a treatment for cancer was the demonstration that tumors depend on angiogenesis for sustained growth . Because angiogenesis is essential for tumor growth and metastasis, controlling tumor-associated angiogenesis is a promising tactic in limiting cancer progression. Therefore, considerable efforts have been directed toward anti-angiogenic therapy as a new modality in the treatment of human cancers.
Anti-Angiogenic Gene Therapy
Recently, anti-angiogenic therapies have received widespread attention. Gene therapy represents an attractive alternative to recombinant protein administration for multiple reasons, such as the ability to achieve direct in vivo delivery of a gene of interest to a target tissue. Indeed, gene therapy holds promise as a strategy for single-enzyme deficiency disorders but also as a powerful tool in the treatment of cancer. However, a major challenge is the design of an efficient delivery method of the gene of interest to the target tissue. Of note, a variety of strategies have been attempted to combat this challenge. In this section, we group the most recently described strategies of anti-angiogenic gene therapy into three broad categories: vector strategy, tissue-specific targeted gene therapy, and a developed targeted adeno-associated virus phage (AAVP) delivery system for use in anti-angiogenic gene therapy.
Vector Strategies for Anti-Angiogenic Gene Therapy
The preclinical works performed in anti-angiogenic gene therapy demonstrated that blocking angiogenesis allowed for the potential control of tumor growth. A variety of nonviral and viral vectors have been used in gene therapy. This section summarizes the most recent vector strategies that have been utilized.
Nonviral Vectors
Nonviral vectors possess several advantages, such as their safety and easy modifiability, but they have a lower transfection efficiency than viral vectors. However, in the past several years, advancements in the field have improved the efficiency of these vectors. A number of different nonviral vectors have been used for anti-angiogenic gene therapy, including nanoparticles, cationic liposomes, and low-voltage electroporation ( Table 2.1 ).
Gene | Vector | Findings | Reference |
---|---|---|---|
HIF-α | Nanoparticle | Systemic delivery of MNP (siHIF) effectively inhibited tumor growth, suppressed cell migration, and disturbed angiogenesis in a PC3 prostate cancer xenograft murine model without activating innate immune responses. | |
P53 | Nanoparticle | NP-mediated p53 decreased tumor angiogenic activity and thus reduced tumor growth and improved animal survival in xenografts of p53 mutant tumors. | |
VEGF | Nanoparticle | Intravenous and intratumoral administration of the PEG–VEGF siRNA micelles significantly inhibited VEGF expression at the tumor tissue and suppressed tumor growth in mouse tumor model without showing any detectable inflammatory responses in mice. | |
sFlt-1 | Nanoparticle | Inhibition of tumor growth in BxPC3 pancreatic adenocarcinoma tumor-bearing mice. | |
VEGF | Nanoparticle | Inhibition of tumor growth and angiogenesis in mouse tumor model. | |
Argonaute-2 | Cationic liposome | Systemic injection with the lipoplex containing siRNA for the Argonaute-2 gene (apoptosis-inducible siRNA) resulted in significant antitumor effect without severe side effects in mice with Lewis lung carcinoma. | |
Survivin | Cationic liposome | Inhibited cell proliferation and tumor angiogenesis and increased apoptosis in hepatoma tumor xenografts. | |
Angiostatin and endostatin | Low-voltage electroporation | Decreased microvessel density and inhibited tumor growth |
Nanoparticles
Polymeric drug carriers are used in the delivery of low-molecular-mass drugs, nucleotides, and peptides. Liu et al . reported that a cationic mixed micellar nanoparticle (MNP) consisting of amphiphilic block copolymers poly(ε-caprolactone)-block–poly(2-aminoethylethylene phosphate) (PCL(29)-b–PPEEA(21)) carrier for HIF-1α siRNA inhibited HIF-1α expression and resulted in the disruption of angiogenesis and therefore suppressed tumor cell proliferation . Prabha et al . also developed a polymeric nanoparticle (NP) for p53 gene delivery in the inhibition of tumor angiogenesis and growth . Polyethylenimine (PEI) has a high cationic charge density and can bind condensed siRNA and plasmid, which protects the siRNA against nucleases and reduces its renal clearance. siRNA and plasmid–PEG/PEI polyelectrolyte complexes were formulated using polyethylene glycol (PEG) and PEI, and the modified siRNA and plasmid were successfully applied . A small RNA delivery system using PEI–PEG–APRPG polymer for targeting VEGF has been observed to effectively deliver siRNA to tumors overexpressing VEGF and thereby inhibiting angiogenesis and tumor growth . Utilizing an α ν β 3 integrin–ligand coupled nanoparticle approach, Salehi-Had et al . developed an anti-angiogenic drug for imaging targeted gene therapy for the treatment of choroidal neovascularization . These findings indicate that nanoparticles could eventually prove to be a useful and safe delivery system for anti-angiogenic gene therapy.
Cationic Liposome
Liposomes are microscopic spherical vesicles of phospholipids and cholesterol. Liposomes have been evaluated as delivery systems for a great variety of molecules, including small drug molecules, nucleotide, proteins, and even plasmids. Cationic liposome shows promise as a carrier for nucleic acids because it can selectively bind to the endothelial cells of blood vessels in tumor tissues . The main advantage of this strategy is the relatively high gene transfer efficiency compared to that of other nonviral transfection strategies. It also has the potential for broad application due to its ability to work with many genes of interest. A successful case of angiogenesis inhibition resulted in significant antitumor effect without severe side effects in the in vivo model of lung carcinoma using Argonaute-2 siRNA carried by a cationic liposome . In addition, siRNA/cationic liposome has been shown to inhibit proliferation, induce apoptosis, and enhance radiosensitivity in human hepatoma cells in in vitro and in vivo models . These findings further indicate that cationic liposome-mediated systemic delivery of a drug has the potential to achieve anti-angiogenic gene therapy, resulting in the suppression of tumor growth.
Low-Voltage Electroporation
Electroporation gene therapy is a strategy that aims to disrupt cellular membranes with high-voltage electrical currents, resulting in the formation of pores through which applied naked DNA can traverse . The advantages of this strategy are similar to those of lipofection in that a vast array of cell types could theoretically be targeted in a relatively simple manner with this technique. Uesato et al . successfully demonstrated the antitumor effect of two anti-angiogenic genes, angiostatin and endostatin, which are delivered to the tumor cells by low-voltage electroporation . This finding suggests that in vivo delivery of anti-angiogenic genes with low-voltage electroporation could be a possible therapeutic strategy for established solid tumors when both genes are applied in combination.
Viral Vectors
The use of viral vectors in gene therapy is an approach that has the potential for achieving direct, targeted in vivo delivery of the gene payload. The desired characteristics of a viral vector for gene therapy are its easy purification into high titers to mediate targeted gene delivery and its prolonged gene expression with minimal side effects. Four main classes of viral vectors in preclinical studies with clinical application for anti-angiogenic gene therapy are adenoviruses, adeno-associated viruses (AAVs), retroviruses, and lentiviruses. The main difference between these vectors is that retroviruses and lentiviruses can integrate themselves into the genome, whereas the other two classes predominantly persist as extrachromosomal episomes .
Retroviral Vectors
Retroviruses are a class of enveloped viruses that contain a single-stranded RNA genome. They are able to efficiently integrate permanently into the human genome, where they provide the basis for permanent expression of up to 8 or 9 kb of foreign DNA. This vector strategy is highlighted by several major advantages, including the integration of the gene of interest into chromosomes, stable expression, and the unique life cycle of the virus causing no immunogenicity by virtue of using a complementary packaging cell line to generate retroviral infectious particles. However, these advantages come at a cost. Namely, the chromosomal integration is random, alluding to the potential for insertional mutagenesis. Furthermore, this strategy is limited by its inability to transduce quiescent cells. Tsai et al . developed a γ-retrovirus vector modified from Moloney murine leukemia virus carrying the interleukin-2 (IL-2) gene for bladder cancer therapy . Animal studies showed that these envelope-modified γ-retroviruses carrying the IL-12 gene exerted higher antitumor activity in terms of retarding tumor growth and prolonging the survival of tumor-bearing mice than unmodified ones. This was associated with enhanced tumor cell apoptosis, as well as increased intratumoral levels of IL-12. Rocha’s group also developed an endostain and IL-2-expressing replication-competent retrovirus bicistronic vector for anti-angiogenic gene therapy of metastatic renal cell carcinoma . The first strategy behind the use of a retrovirus bicistronic vector is that it uses separated promoters to drive the expression of different genes in the same vector. The second strategy of its use is the coexpression of different genes, separated by an internal ribosome entry site (IRES) sequence expressed as a single transcriptional cassette under the control of a common upstream promoter. Therefore, IRES enables vectors to produce multiple products from a single transcriptional unit, thereby eliminating the loss of gene expression as a result of promoter competition or counterselection. The studies showed that retroviral bicistronic gene transfer led to the secretion of functional endostain and IL-2 that were sufficiently active to inhibit tumor angiogenesis and tumor cell proliferation. A number of studies utilizing a retroviral vector for the delivery of anti-angiogenic gene therapy are listed in Table 2.2 .
Gene | Vector | Findings | Reference |
---|---|---|---|
IL-2 | Retrovirus | Antitumor activity and prolonged the survival of tumor-bearing mice. | |
Endostatin and IL-2 | Retrovirus | (1) Inhibited tumor angiogenesis and tumor cell proliferation and (2) increased the infiltration of immune cells. | |
Metastasis-associated protein 1 | Retrovirus | Suppressed the invasion and angiogenic activity of the cells in vitro and delayed tumor formation and development in prostate cancer mouse xenografts. | |
Endostatin | Retrovirus | Microvascular density and proliferation of tumor cell were significantly decreased in mice bearing renal cell carcinoma. | |
PEDF | Retrovirus | Decreased microvessel density and caused significant inhibition of tumor growth in pancreatic cancer mouse xenografts. | |
VEGF | Retrovirus | Reduced the vascularization and abolished the tumor growth in glioblastoma mouse xenografts. | |
VEGF-receptor 2 | Retrovirus | Inhibiting tumor angiogenesis and proliferation in mice bearing human breast cancer cells. | |
bFGF | Retrovirus | Inhibition of B16 melanoma growth in vivo. | |
Matrilysin | Retrovirus | Low invasiveness and slow tumor growth. |
Adenovirus
Adenovirus is a double-stranded DNA virus that binds initially to the target cell through a viral fiber protein. The adenovirus has been used more recently as an effective vector for gene delivery because it can be produced in high titers and can efficiently deliver the therapeutic gene . With advancing recombinant DNA technology, the adenovirus vectors can accommodate up to 7.5 kb of foreign DNA while targeting not only actively dividing cells but also quiescent cells . This is achieved via the interaction of the adenoviral fiber monomers with the coxsackievirus and adenovirus receptor with subsequent receptor-mediated endocytosis via interaction with integrins expressed on the cell surface . Su et al . developed a targeted CDglyTK gene delivery system by adenovirus vector for the anti-angiogenic gene therapy of breast cancer . Furthermore, Yao et al . generated a tumor vascular tissue-specific gene therapy system using adenovirus conjugated to PEG . The study showed that systemic administration of Adv–PEG (CGKRK) resulted in an approximately 500-fold higher transgene expression in tumor-associated vasculature than that of unmodified Adv vector . The latest generation of adenoviral vector is the gutless adenovirus. It is an attractive agent for gene therapy because of its impressive safety profile due to the reduction of in vivo immune response and long-term sustained gene expression. Ghulam’s group in Los Angeles developed a gutless adenovirus vector for gene therapy . Analysis of the efficacy, safety, and toxicity of this gutless adenovirus in the animal model strongly supports further clinical testing in the treatment of glioma . These results indicate that adenovirus vector has a suitable efficacy and safety for systemic anti-angiogenic gene therapy. As with viral vectors, a larger number of studies investigating the use of adenovirus vectors for the delivery of anti-angiogenic gene therapy have been conducted and are listed in Table 2.3 .
Gene | Vector | Findings | Reference |
---|---|---|---|
CDglyTK | Adenoviral | Tumor cell proliferation and microvessel density of tumor tissue were significantly reduced in breast cancer mouse xenografts. | |
HSVtk | Adenoviral | Inhibition of tumor angiogenesis with negligible side effect in liver cancer mouse xenografts. | |
P16 | Adenoviral | Exhibited multiple antitumor functions by simultaneously suppressing in vitro growth and in vivo angiogenesis of breast cancer cells, blocking cell division, as well as inducing senescence and apoptosis. | |
Vasohibin-1 | Adenoviral | Significantly reduced aberrant angiogenesis in the fibro-obliterative tissue in a murine lung cancer model. | |
VEGF receptor | Adenoviral | Inhibition of the tumor growth and angiogenesis on nude mice model of colorectal, gastric, and liver cancers. | |
CALR and MAGE-A3 | Adenoviral | Intratumoral injection of Ad-CALR/MAGE-A3 suppressed tumor growth and angiogenesis in the in vivo model of glioblastoma. | |
IL-24 | Adenoviral | Inhibition of tumor angiogenesis in a xenograft mouse model for melanoma. | |
Endostatin | Adenoviral | Inhibition of tumor angiogenesis in a xenograft mouse model for head and neck squamous cell carcinoma. |
Adeno-Associated Virus
AAV vectors have the advantage of a broad host range with a low level of immune response and longevity of gene expression. Eukaryotic AAV is an example of a chimeric vector containing parvovirus and adenovirus. This chimeric vector is able to not only transfect mitotic and quiescent cells but also stably integrate its DNA at predictable locations within chromosome 19. Moreover, major advantages of this vector strategy are the lack of immunogenicity and pathogenicity in humans. For gene therapy using AAP vector, recent studies have focused on anti-angiogenic therapy ( Table 2.4 ). Pihlmann et al . reported that an AAV-delivered microRNA clusters system efficiently downregulated VEGF expression and resulted in the inhibition of angiogenesis in a preclinical study . Wu et al . also reported that recombinant AAV-mediated PEDF successfully inhibits tumor angiogenesis and metastasis in the colorectal peritoneal carcinomatosis (CRPC) mouse model . As with viral vectors, a number of studies investigating the use of AAV vectors for the delivery of anti-angiogenic gene therapy have been conducted and are listed in Table 2.4 .
Gene | Vector | Findings | Reference |
---|---|---|---|
VEGF | Adeno-associated virus | Knockdown VEGF in vitro and in vivo by AAV delivery microRNA. | |
PEDF | Adeno-associated virus | Inhibited angiogenesis and induced apoptosis in tumor tissues from a murine colorectal peritoneal carcinomatosis model. | |
PEDF | Adeno-associated virus | Decreased microvessel density and increased apoptosis in the tumor tissue of lung cancer mouse model. | |
HSV-TK and endostatin | Adeno-associated virus | Inhibition of tumor growth and angiogensis in a bladder cancer mouse model. | |
Endostein | Adeno-associated virus | Controlled bladder tumor cell growth and angiogenesis in an in vivo model. | |
Endostein | Adeno-associated virus | Increased endoreduplication in endothelial cells and inhibited metastasis of breast cancer in vivo model. | |
hING4 | Adeno-associated virus | Inhibited tumor growth and angiogenesis of Panc-1 pancreatic cancer subcutaneously xenografted in vivo. | |
IP-24 | Adeno-associated virus | Inhibition of angiogenesis and tumor cell proliferation in hepatocarcinoma mouse model. | |
hSulf1 | Adeno-associated virus | Inhibition of tumor growth and angiogenesis in vitro and in vivo . | |
HGFK1 | Adeno-associated virus | Inhibited endothelial cell migration and proliferation and prolonged survival of mice with colon cancer. | |
VEGF | Adeno-associated virus | Inhibition of angiogenesis. | |
HOXD10 | Adeno-associated virus | Inhibition the growth of pituitary tumors in nude mouse model. |
Lentiviral Vectors
Lentiviral vectors represent a new vector system that can achieve permanent integration of the gene into nondividing cells. Gene transfer can be achieved in very quiescent cells, which are nondividing or terminally differentiated, such as neurons. Lentiviral vectors are especially useful in transducing cells that lack receptors for adenoviruses. A broad tissue tropism for lentivirus can be achieved using a variety of viral envelopes . Zhou et al . described a novel method that can suppress the expression of angiopoietin-2 at transcriptional and translational levels in pancreatic cancer models by the lentivirus-mediated siRNA system . Guo’s group also demonstrated that a novel targeted recombinant self-inactivating lentivirus vector expressing alphastatin had the ability to transfer genes into human umbilical vein endothelial cells and resulted in anti-angiogenic activities . Many studies utilizing lentiviral vectors for the delivery of anti-angiogenic gene therapy are listed in Table 2.5 .
Gene | Vector | Findings | Reference |
---|---|---|---|
Angiopoietin-2 | Lentiviral | Inhibited pancreatic carcinoma angiogenesis and growth by downregulating the expression of VEGF and CD34 in in vitro and in vivo models. | |
Angiopoietin-2 | Lentiviral | Suppressed metastasis in pancreatic cancer mouse models by reduced matrix metalloproteinase-2. | |
Alphastatin | Lentiviral | Inhibited VEGF and bFGF expression and decreased tumor angiogenesis in a glioma mouse model. | |
Cyclin D | Lentiviral | Suppressed the growth, invasiveness, tumorigenicity, and pro-angiogenic potential of human pancreatic cancer cells in nude mice. | |
VEGF | Lentiviral | VEGF expression and microvascular density in tumor tissues were significantly decreased in nude mice. | |
IFN-α | Lentiviral | Increased in ischemic tumor necrosis and reduced in microvessel density in an ovarian cancer mouse model. | |
Met receptor | Lentiviral | (1) Inhibited tumor cell proliferation and survival in a variety of human xenografts, (2) impaired tumor angiogenesis by preventing host vessel arborization, and (3) suppressed or prevented the formation of spontaneous metastases. |
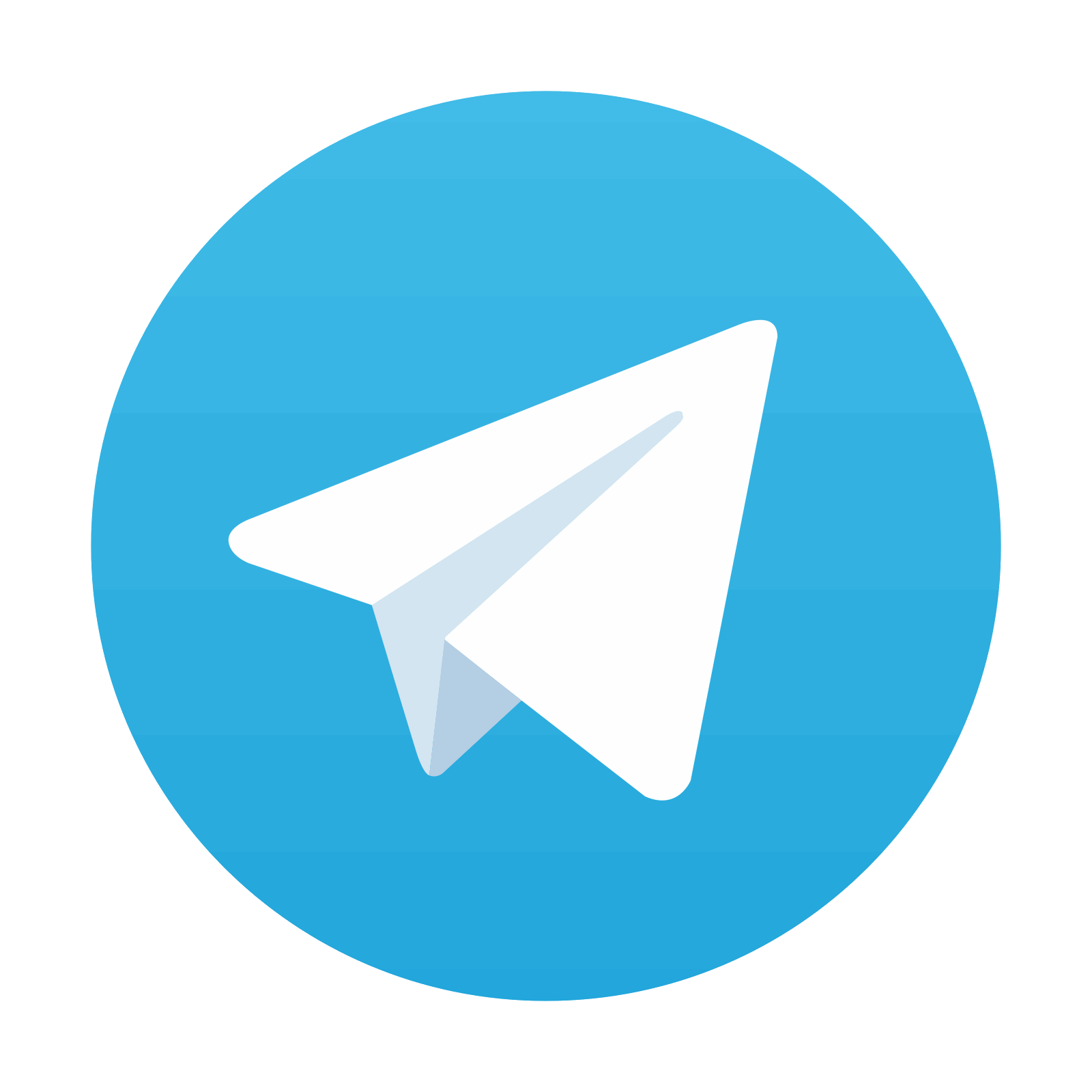
Stay updated, free articles. Join our Telegram channel

Full access? Get Clinical Tree
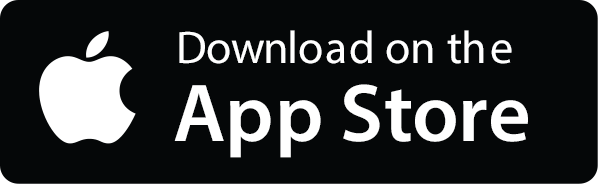
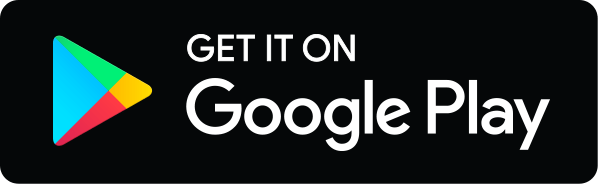
