The elucidation of the heat shock response (HSR) as a mediator of cellular stress has created a framework for understanding how these processes may promote tumorigenesis. Furthermore, the identification of specific components of the HSR and how they are co-opted by cancer cells has led to the discovery of new therapeutic targets. A wide range of small molecule inhibitors of the HSR are in various stages of development for clinical application in patients with cancer. The introduction of these novel small molecule inhibitors offers the opportunity for synergy with existing therapies and the potential for highly targeted treatments.
Key points
- •
Components of the heat shock response (HSR) have become increasingly well defined.
- •
The HSR is active in all phases of tumorigenesis.
- •
Targeting the substrates of the HSR is an attractive and novel means of cancer therapy.
- •
Agents that target the HSR are in various stages of development for clinical use in a variety of cancer types.
- •
The ability of these new agents to synergize with current therapies may be significant.
Introduction
The heat shock response (HSR) is a highly conserved, precisely orchestrated, and internally regulated series of genetic and biochemical events that is triggered by the exposure of eukaryotic cells to various forms of stress. The response was first reported in 1962 by Ritossa, who observed the appearance of a new pattern of chromatin puffs on the salivary gland chromosomes of the fruit fly, Drosophila bucksii , in response to exposure to increased temperatures. Subsequent studies revealed that this response could be induced by a variety of other stresses such as exposure to heavy metals and oxidative stress. In 1973, Tissières and colleagues further defined this response at a molecular level, describing a brisk and dramatic increase in the synthesis of a small, interrelated family of proteins that came to be known as the heat shock proteins (HSPs). This transcriptional response to stress was then discovered to be mediated by a family of heat shock transcription factors (HSFs) that are negatively regulated and activated by the initiation of specific cellular events.
Cell stress is a defining event and efficient use of the stress response can be the difference between cell survival and extinction. The HSR serves a primarily cytoprotective function and is autoregulated so that it is just as promptly switched off, either when the stress has been overcome and homeostasis restored or when the cell has been overwhelmed and cell death ensues. The seamless coordination of these complex interactions involves multilevel crosstalk between the HSR and multiple, diverse, proliferative, antiapoptotic, and death receptor pathways. If these environmental or physiologic stresses are prolonged, then aberrations of the HSR can result in defective development and the establishment of a wide variety of disorders associated with a broad spectrum of disease states ranging from neurodegenerative diseases to ischemia-reperfusion injury.
Given their role in proteostasis, and therefore cytoprotection, increased expression of molecular chaperones is noted in several pathologic disease states that are characterized by increased synthesis and accumulation of aberrant proteins, notable among which is cancer. Demonstration of the increased expression of HSPs in cancer has led to the hypothesis that transformed cells co-opt the HSR to perpetuate the malignant phenotype. This increased expression has been noted in several different cancers: solid organ, gastrointestinal, and hematopoietic. Further, increased expression correlates with aggressiveness and resistance to therapy, which has resulted in extensive research in the past decade to elucidate mechanisms whereby transformed cells might exploit the stress machinery. Increased understanding of these interactions has sparked an interest in developing modulators of the HSR as a means of treatment.
In this article, the various components and mechanisms driving the HSR are detailed with special emphasis on its importance in fostering and maintaining the malignant phenotype. Current research, clinical applications, and future directions in the area of pharmacotherapy targeting this ubiquitous cellular response to stress are discussed.
The HSR
The rapid and prolific transcriptional activation of the HSP genes is under the control of HSFs. Four HSFs are known to exist, of which HSF-1, HSF-2 and HSF-4 are found in humans. HSF-1 is the primary activator of the inducible stress response in human tissues. At baseline conditions of homeostasis, HSF-1 exists in a repressed state in the cytoplasm where it is bound to the complex of HSP40/HSP70 and HSP90 as an inert monomer. On activation, it undergoes hyperphosphorylation at serine residues, forms homotrimers that have DNA-binding capacity, and translocates to the nucleus. There it binds to promoter regions on the 5′ end of the HSP genes, located at variable distances upstream from the site of transcription initiation. These promoter regions contain specific DNA sequence motifs that constitute the stress-responsive heat shock elements (HSEs). It is widely thought that the appearance of many nonnative and/or nascent polypeptides within the cell as a result of environmental or physiologic stress is the primary activator of HSF-1. HSF-1 activity is turned off via internally regulated and autoregulated mechanisms. Acting as a feedback loop, HSP70, which is elaborated from HSF-1 activity, binds to the transactivation domain of HSF-1 and represses further transcription of heat shock genes.
The HSR
The rapid and prolific transcriptional activation of the HSP genes is under the control of HSFs. Four HSFs are known to exist, of which HSF-1, HSF-2 and HSF-4 are found in humans. HSF-1 is the primary activator of the inducible stress response in human tissues. At baseline conditions of homeostasis, HSF-1 exists in a repressed state in the cytoplasm where it is bound to the complex of HSP40/HSP70 and HSP90 as an inert monomer. On activation, it undergoes hyperphosphorylation at serine residues, forms homotrimers that have DNA-binding capacity, and translocates to the nucleus. There it binds to promoter regions on the 5′ end of the HSP genes, located at variable distances upstream from the site of transcription initiation. These promoter regions contain specific DNA sequence motifs that constitute the stress-responsive heat shock elements (HSEs). It is widely thought that the appearance of many nonnative and/or nascent polypeptides within the cell as a result of environmental or physiologic stress is the primary activator of HSF-1. HSF-1 activity is turned off via internally regulated and autoregulated mechanisms. Acting as a feedback loop, HSP70, which is elaborated from HSF-1 activity, binds to the transactivation domain of HSF-1 and represses further transcription of heat shock genes.
HSPs
At a molecular level, the hallmark of the HSR is the increased synthesis of the HSP family of proteins. HSPs constitute subsets within at least 2 larger, well-characterized families of proteins, namely molecular chaperones and proteases. Their presumed primary function is to combat the proteotoxic state induced by various forms of cell stress. By virtue of their structure and function, proteins are particularly susceptible to physiologic stress. Increased temperatures, oxidative agents, heavy metals, and low pH can all lead to unfolding of proteins, thus exposing their hydrophobic core motifs and making them prone to misfolding. Misfolding may promote the aggregation and precipitation of unstable intermediaries, which eventually threatens cell survival. Molecular chaperones perform the task of protein assembly, folding, refolding of intermediates, membrane translocation, and sequestration in subcellular compartments. In situations in which the exposure is of a lethal intensity, irreversibly damaged proteins are processed by a related family of proteins, the proteases, to ensure prompt degradation and removal.
HSPs were initially classified into 6 major families, based on approximate molecular weight: HSP100, HSP90, HSP70, HSP60, HSP50, and small HSPs. More recently, the nomenclature was revised by Kampinga and colleagues to align with that of the Human Genome Organisation (HUGO) Gene Nomenclature Committee and thereby promote consistent reporting. The new nomenclature classifies the HSPs into the following groups: HSPH (HSP110), HSPC (HSP90), HSPA (HSP70), DNAJ (HSP40), and HSPB (small HSPs). This article discuss in further detail 2 of the most widely studied and targeted families of HSPs.
HSPA
Members of this subclass of HSPs are major molecular chaperones and cytokine chaperokines found in the cytoplasm, nucleus, mitochondria, and endoplasmic reticulum. There are at least 13 homologous proteins in the human HSPA chaperone family and they seem to be highly conserved in eukaryotes. These proteins can be either stress-inducible (HSPA1) or constitutively expressed (HSPA8). They have the ability to interact with newly synthesized polypeptides as they emerge from ribosomes or to bind to nonnative or damaged proteins that result from exposure to stress. In addition, they assist in trafficking proteins into cell organelles, and they also seem to play a role in dissociation of aggregated proteins. These multiple, diverse functions underline the significance of HSPA to the maintenance of protein homeostasis during normal biochemical cellular processes as well as in the stressed state.
All HSPA family members have a multidomain organization that consists of an N-terminal nucleotide-binding domain (∼40 kDa) with ATPase activity and a C-terminal peptide-binding domain (∼25 kDa) with a short linker. The interaction of HSPA with protein substrates depends on ATP hydrolysis. In the ATP-bound state, HSPA has low binding affinity for substrates. Functional activation requires assembly into large, complex chaperone machines constituted through association with cochaperones including the DNAJ family of HSPs. DNAJ causes stimulation of ATP hydrolysis, and the resulting ADP-bound HSPA has high substrate-binding affinity. All cellular functions performed by the HSPA family depend on ATP-dependent association with, and dissociation from, substrate proteins. By regulating the configuration and affinity of HSPA within the chaperone machines, DNAJ, and other cochaperones, can modulate its substrate selectivity and therefore recruit it for a multitude of intracellular functions.
HSPC
HSPC is another example of an evolutionarily highly conserved molecular chaperone, existing from bacteria to mammals. Another ATP-dependent chaperone, it is essential in the cellular response to stress. The proteostatic functions of HSPC are even more diverse, playing an integral role in constitutive cell signaling in the nonstressed state. More than 200 client proteins are stabilized and/or activated via interaction with HSPC. Similar to HSPA, this task is accomplished through the construction, together with HSPA, DNAJ, and other cochaperones, of a dynamic, interactive complex called the HSPC chaperone machine. HSPC is abundant even in nonstressed cells, and a multitude of client proteins rely on interactions with the chaperone machinery to acquire their active conformations.
The molecular mechanisms underlying the function of HSPCs remain less well understood compared with other chaperone proteins. The reasons for this are multifactorial. The complexes formed between HSPC and its client proteins are dynamic and short lived, complicated further by the abundant and variable participation of cochaperones. HSPC interacts with more than 20 cochaperones, and each of them plays a part in modulating substrate affinity, specificity, and activity. Via abundant expression and numerous interactions with a variety of proteins, HSPC creates a dynamic reservoir that serves to buffer proteotoxic stress. However, under extreme conditions this reservoir is prone to rapid depletion, leaving the cell susceptible to the altered structure and function of a plethora of HSPC client proteins.
A broad classification of the functions of molecular chaperones has traditionally been entertained, defined as protein holding and protein folding. Both HSPA and HSPC are involved mainly in protein holding. HSPA primarily interacts with nascent polypeptides, safeguarding their refolding potential and preventing the formation of misfolded, insoluble intermediates, and then guides them back to productive, on-pathway folding. Protein folding takes place once the substrate has been released and is regulated primarily by HSPD. However, these distinctions are not absolute and there is overlap and redundancy. For example, HSPA can cooperate with other ATP-dependent chaperones (including HSPC) and chaperonins to mediate folding of certain substrates. In deciding the fate of damaged proteins, HSPA and HSPC seem to have divergent functions: HSPA promotes substrate ubiquitination leading to elimination, whereas HSPC favors protein preservation.
The HSR and tumorigenesis
Essential Steps in Tumorigenesis
Hanahan and Weinberg examined the molecular, biochemical, and cellular traits displayed by most human cancers. In a landmark review, they set forth the principles that govern the transformation of normal cells to malignant tumors. There is now almost universal agreement that the evolution of the malignant phenotype requires these key alterations in cell physiology: self-sufficiency in growth signals, insensitivity to growth-inhibitory (antigrowth) signals, evasion of programmed cell death (apoptosis), limitless replicative potential, sustained angiogenesis, and tissue invasion and metastasis. Via their ability to hold a large pool of proteins in their active conformations, HSPs modulate the function of several important signaling proteins and are therefore involved in many of these steps on the pathway to tumor progression, as summarized in Table 1 .
Hallmarks of Cancer | HSPs Implicated | Molecules | References, Year |
---|---|---|---|
Self-sufficiency in growth signals | HSP70 | Cyclin D1 | Diehl et al, 2003 |
HSP90 | HER-2, c-Src, c- Raf , ERK1, Akt, Bcr-Abl, EGFR, BRAF, HCK, Cdk4, and eIF-2α | Taipale et al, 2010 | |
Insensitivity to growth inhibition | HSP90 | CDK4, CDK6 | Bishop et al, 2007 |
Evasion of programmed cell death | HSP27 | Bid, cytochrome C | Beere et al, 2001 Calderwood et al, 2006 |
HSP70 | Caspases, Bax, AIF, APAF-1, JNK, Akt | ||
HSP90 | Caspases, APAF-1, RIP1, AKT | ||
Limitless replicative potential | HSP72 | Hdm2, p53 and Cdc2 | Yaglom et al, 2007 |
HSP70 | p53 | Sherman et al, 2007 | |
HSP90 | Telomerase (h-TERT) | Holt et al, 1999 | |
Sustained angiogenesis | HSP27 | VEGF | Keezer et al, 2003 |
HSP70 | HIF1α | Zhou et al, 2004 | |
HSP90 | HIF1α, VEGF, NOS | Garcia-Cardena et al, 1998 | |
Tissue invasion and metastasis | HSP90 | MMP2 | Eustace et al, 2004 |
Levels of HSPs are increased in a wide variety of cancers. Despite various hypotheses, the mechanism by which this increased expression is effected remains elusive. A general hypothesis proposes that stress stimuli encountered within the tumor microenvironment (hypoglycemia, hypoxia, and low pH) cause canonical induction of the HSR. However, Tang and colleagues showed that although overexpression of HSPs was preserved from tumor samples to cells grown in culture, expression was subsequently downregulated on the establishment of tumor xenografts in vivo using the cultured cells. The mechanisms for HSP overexpression are likely to be complex and multifactorial. Multiple alterations in protein homeostasis occur during the stepwise progression to carcinogenesis that could lead to induction, or derepression, of HSP genes. This process includes the early stages such as infection by oncogenic viruses or exposure to environmental carcinogens; progressive expression of mutated proteins; changes in the immune and endocrine systems; and also cell exposure to a multitude of stresses including hypoxia, starvation, and other proteotoxic stressors.
Coupling of HSF-1 Activation to Malignant Cell Signal Transduction
The activation of HSF-1 during stress has evolved to be a brisk response. Therefore, it does not rely on an increase in transcription or translation, but relies solely on the posttranslational modification of existing levels of HSF-1. However, activation of HSF-1 in cancers is associated with an increase in the detected overall level of the protein. Although the mechanism for this increase is unclear, it has been theorized to involve an increase in transcription/translation or certain epigenetic changes.
Addiction to Chaperones
Genetic mutations and increased protein expression are key events in tumor progression. Transformation is therefore accompanied by an exponential increase in protein load, with many of those proteins being mutated and therefore being inherently unstable. Maintenance of proteostasis within this mutated phenotype causes an enhanced dependence on the chaperoning function of HSPs. Chaperones stabilize oncoproteins and permit, or even enhance, their tumorigenic potential. Therefore, tumor cells show an addiction to chaperones and this may be the cause of their increased HSP expression.
The ability of HSPs to hold large amounts of altered proteins, including protein kinases and transcription factors, may significantly affect vital biologic processes such as signal transduction, chromatin remodeling and epigenetic regulation, development, and morphologic evolution has earned them the nickname capacitors of evolution. Cancer cells co-opt this capacitance machinery to protect an array of mutated and overexpressed oncoproteins from misfolding and therefore from proteasomal degradation. These oncoproteins have a host of different functions that affect each of the physiologic changes that must occur in the pathway to transformation.
Growth Signaling and Anti-apoptosis
HSPs are vital for maintaining signaling proteins in active conformations so as to enable an expeditious response to growth signals. HSPC, along with HSPA and HSPB, organized within high-molecular-weight chaperone machinery, is required for the stability and activity of various significant growth-promoting oncoproteins. Prominent among these is HER-2 and its downstream effectors including Akt, c-Src, and Raf-1. In addition, it has also been shown that HSP90 association is essential for the function and stability of the androgen receptor in prostate cancer cells.
Activation of several oncogenes, notably c-Myc and Ras, also induces pathways of programmed cell death or apoptosis. Therefore, several of the apoptotic pathways must be subverted during the progression to transformation and attainment of the malignant phenotype. HSPA and HSPB, unlike HSPC, do not influence cellular proliferation. However, they do play a role in the inhibition of programmed cell death and, thereby, in the creation of a profoundly resilient state. Some of the high-profile targets of HSPA and HSPB include members of the caspase-dependent apoptotic pathways such as c-Jun kinase, Apaf-1, and caspase 8. In addition, HSPA also plays a role in the inhibition of an alternative death pathway involving digestion of cellular contents by lysosome-derived cathepsins. Inactivation or knockdown of HSPA and/or HSPB in tumor cells leads to spontaneous activation of apoptosis.
Inhibition of Replicative Senescence
All somatic cells have a built-in, preset limit on the number of cell divisions. This limit is regulated by the length of end-chromosomal telomeres, which undergo shortening with every successive cell division. At the preprogrammed point of crisis, the cell enters the pathway of senescence. In some cells, expression of p53-sensitive telomerase is sufficient to bypass crisis and escape senescence. HSPC is essential for telomerase stability. Recent data indicate that specific members of the HSPC and HSPA family are overexpressed in tumors and inhibit senescence via both a p53-dependent and p53-independent manner.
Angiogenesis
To meet increasing tissue demands for oxygen delivery, growing tumor cells use angiogenic growth factor signaling to create neocirculatory pathways at the microscopic level. The primary signal for angiogenesis is hypoxia and the most important sensor for hypoxia in tumor tissues is the hypoxia-inducible factor 1α (HIF1α). HIF1α is regulated primarily at the level of protein stability and both HSPA and HSPC are necessary for its stabilization and accumulation. Several key downstream signaling molecules such as vascular endothelial growth factor (VEGF) and nitric oxide synthase (NOS) require HSPC for their induction and stable functionality.
Invasion and Metastasis
There is evidence to support the involvement of HSPs in this final stage of tumor dissemination. For example, tumor cells that overexpress HSF-1 and HSPs show a higher propensity and capability for invasion and metastases. However, the mechanisms involved in these processes are poorly understood at this time. Existing data show that the induction in some tumor cells of an anchorage-independent state, mediated by heregulin, requires the HFS-1 gene. Other recent studies have also suggested a role for extracellular HSPC. HSPC expressed on the surface of tumor cells can bind to matrix metalloproteinase 2 (MMP2), a key protein in the development of invasion.
Implications of HSP Overexpression
The involvement of HSPs in various stages of oncogenesis, and their aberrant overexpression in a wide variety of tumor specimens, has generated interest in their role as biomarkers, both diagnostic and predictive. There is a potential for the use of circulating levels of HSPs and anti-HSP antibodies for diagnostic purposes, although this potential remains unrealized. However, there is increasing evidence that the overexpression of HSPs in tissue samples is a biomarker of carcinogenesis and the level of expression has been correlated with the degree of differentiation, response to therapy, and outcome prognostication.
Increased levels of HSP27 have been detected in breast and endometrial cancer as well as in leukemia. Moreover, there is variability of the phosphorylation patterns of HSP27 in tumor samples compared with corresponding normal tissue. The expression of this protein confers a poor prognosis in patients with gastric, liver, and prostate cancer. Likewise, expression of the HSP70 protein correlates with unfavorable outcomes in breast, endometrial, uterine cervical, and bladder carcinomas. In addition, increased expression of HSP90 has been linked with disease progression in melanoma and correlated with poor survival outcomes in cancers of the breast and lung as well as in gastrointestinal stromal tumors (GIST).
The HSP27 gene also contains an estrogen-responsive element that might significantly alter expression in hormone receptor–positive tumors. However, this has not been shown to reliably predict response to hormonal therapy. In contrast, both HSP27 and HSP70 seem to confer resistance to chemotherapy in breast cancer. Although HSP27 correlates with poor response to chemotherapy in patients with leukemia, HSP70 predicts a favorable response to chemotherapy in osteosarcomas.
Targeting the HSR in Cancer
Via their function as molecular chaperones, HSPs aid the accumulation of mutated proteins within the cancer phenotype, thus conferring cytoprotection against otherwise lethal levels of cellular stress. In addition, via crosstalk with interconnected signal transduction pathways, HSPs influence several key steps in tumorigenesis, which makes HSPs and their protective mechanisms an attractive target for antitumor therapy.
The abundance and ubiquitous expression of HSPs in normal cells seem to render them unlikely targets for meaningful drug development secondary to a lack of selectivity. However, the addiction to chaperones prevalent in transformed cells renders them particularly vulnerable to inhibition of the activity of HSPs. Because the heat shock machinery is already being driven and used at maximal levels in the cancer cell, any decrease in the activity of HSR mechanisms can cause a dangerous imbalance favoring cell death. In addition, not only are cancer cells enriched in HSPs but studies show that there is preferential expression of a certain cancer phenotype of HSPs in tumors compared with normal cells. In the case of HSP90, the altered conformation of the proteins in tumor cells leads to a 20-fold to 200-fold higher specificity for small molecule inhibitors compared with normal cells.
Another perceived obstacle for drug development has been that HSPs interact with substrates in a stoichiometric manner and lack catalytic activity. Therefore, researchers have used high-throughput models that provide a reliable readout of the transcriptional response to cell stress to successfully screen millions of small molecules. An ever-increasing number of molecules are currently in preclinical or clinical evaluation with some promising results.
Modulation of HSF-1
Given the central role of HSF-1 in the regulation of HSP expression in the stressed cell, it has attracted attention as a target for drug development. Drugs have been developed to both positively as well as negatively modulate HSF-1. Induction of the HSR is a useful therapeutic strategy, mainly in diseases in which the aggregation of misfolded proteins is the key underlying pathogenic defect, exemplified by neurodegenerative disorders ( Table 2 ).
HSF-1 inhibitors | — | Quercetin KNK-437 NZ28 Emunin |
HSP70 inhibitors | ABD |
|
PBD | PES | |
Aptamers | ADD70 | |
HSP90 inhibitors | Natural |
|
Synthetic |
| |
Isoform specific | NECA (GRP-94) | |
Posttranslational |
| |
Inhibitors of cochaperones | HSP40 DNAJ Hop | MAL2-11B D-peptide Pyrimidotriazinediones |
Multitargeted agents | HSF-1, NF-kB and AP-1 HSR, p53 and NF-kB | Triptolide Curaxins |
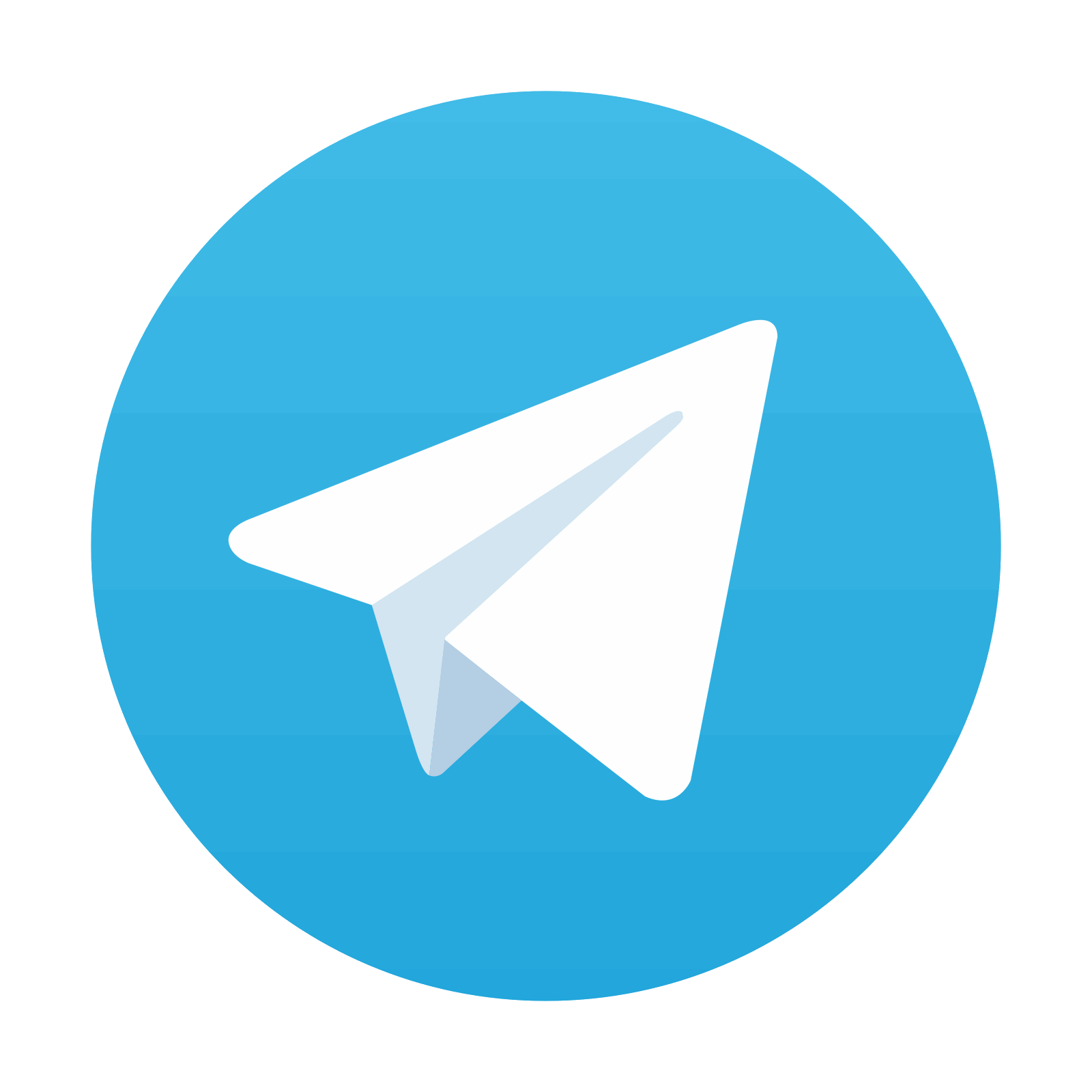
Stay updated, free articles. Join our Telegram channel

Full access? Get Clinical Tree
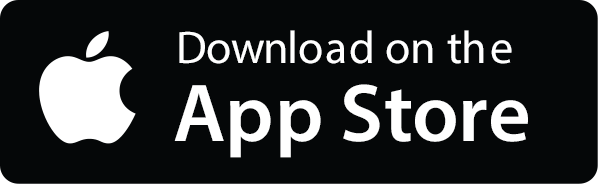
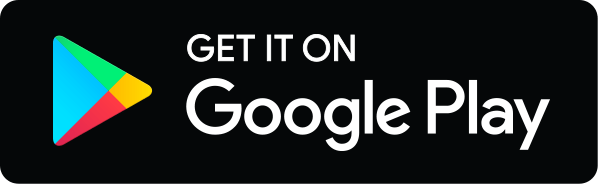
