The role of the microenvironment during the initiation and progression of malignancy is appreciated to be of critical importance for improved molecular diagnostics and therapeutics. The tumor microenvironment is the product of a crosstalk between different cells types. Active contribution of tumor-associated stromal cells to cancer progression has been recognized. Stromal elements consist of the extracellular matrix, fibroblasts of various phenotypes, and a scaffold comprised of immune and inflammatory cells, blood and lymph vessels, and nerves. This review focuses on therapeutic targets in the microenvironment related to tumor endothelium, tumor associated fibroblasts, and the extracellular matrix.
Key points
- •
The role of the microenvironment during the initiation and progression of malignancy is appreciated to be of critical importance for improved molecular diagnostics and therapeutics.
- •
The tumor microenvironment is the product of a crosstalk between different cells types. Critical elements in the microenvironment include tumor-associated fibroblasts, which provide an essential communication network via secretion of growth factors and chemokines, inducing an altered extracellular matrix, thereby providing additional oncogenic signals that enhance cancer-cell proliferation and invasion.
- •
Therapeutic targeting of angiogenesis factors, tumor associated fibroblasts, and cell adhesion molecules compose are an active area of clinical trial investigation to determine efficacy of these approaches.
- •
Some angiogenesis inhibitors are approved by the Food and Drug Administration for cancer, yet further research is needed to improve efficacy in resistant tumors.
- •
Clinical trials targeting integrins and tumor-activated fibroblasts are being conducted.
Tumor microenvironment
The role of the microenvironment during the initiation and progression of malignancy is appreciated to be of critical importance for improved molecular diagnostics and therapeutics. The tumor microenvironment is the product of crosstalk between different cells types. For instance, in epithelial tumors, these cells include the invasive carcinoma and its stromal elements. Critical elements in the microenvironment include tumor-associated fibroblasts, which provide an essential communication network via secretion of growth factors and chemokines, inducing an altered extracellular matrix (ECM), thereby providing additional oncogenic signals that enhance cancer-cell proliferation and invasion. Active contribution of tumor-associated stromal cells to cancer progression has been recognized. Stromal elements consist of the ECM, fibroblasts of various phenotypes, and a scaffold comprised of immune and inflammatory cells, blood and lymph vessels, and nerves. This review focuses on therapeutic targets in the microenvironment related to tumor endothelium, tumor-associated fibroblasts, and the ECM, whereas the immune targets are covered in the immunotherapy article in this issue.
Tumor microenvironment
The role of the microenvironment during the initiation and progression of malignancy is appreciated to be of critical importance for improved molecular diagnostics and therapeutics. The tumor microenvironment is the product of crosstalk between different cells types. For instance, in epithelial tumors, these cells include the invasive carcinoma and its stromal elements. Critical elements in the microenvironment include tumor-associated fibroblasts, which provide an essential communication network via secretion of growth factors and chemokines, inducing an altered extracellular matrix (ECM), thereby providing additional oncogenic signals that enhance cancer-cell proliferation and invasion. Active contribution of tumor-associated stromal cells to cancer progression has been recognized. Stromal elements consist of the ECM, fibroblasts of various phenotypes, and a scaffold comprised of immune and inflammatory cells, blood and lymph vessels, and nerves. This review focuses on therapeutic targets in the microenvironment related to tumor endothelium, tumor-associated fibroblasts, and the ECM, whereas the immune targets are covered in the immunotherapy article in this issue.
Angiogenesis is necessary for tumor growth
The initial evidence that angiogenesis is necessary for tumor growth came from studies transplanting cancer cells into the avascular corneas of rabbits. In these studies, tumors did not grow in rabbit corneas before sprouting vessels were able to grow to connect to the tumor. Additionally, inhibiting blood vessel formation prevented tumor growth beyond 0.4 mm 2 . Similar studies found that tumors placed in chicken embryo chorioallantoic membranes shrank during the first 3 days after placement. However, new vessel formation was seen to form from existing vessels after the tumors were placed. When these new vessels connected to the tumor, tumor growth resumed. These studies not only identified a significant role of vessel formation in tumor progression but they also identified that tumors elicited the growth of vessels from existing vessels. This finding suggested that tumors released diffusible factors that initiate angiogenesis to continue and maintain their growth.
There are 4 known strategies by which tumors can augment their blood supply. They can stimulate angiogenesis, use existing vessels directly, induce vasculogenesis, or form vasculogenic networks without vascular cells. The secretion of proangiogenic factors and inhibition of antiangiogenic factors induce vascular sprouting from preexisting capillaries and venules, which constitutes the process of angiogenesis. This mechanism is the most common way tumors gain access to the vasculature. Tumors may also use existing vessels by growing alongside them, as is the case in astrocytomas. The strategy of vasculogenesis involves the formation of blood vessels from bone marrow precursors. This strategy differs markedly from angiogenesis in that the sources of the cells that make up the vessels are from the bone marrow and not from preexisting vessels. However, many of the soluble mediators that initiate vasculogenesis, notably vascular endothelial growth factor (VEGF), parallel those found in angiogenesis. Lastly, tumors themselves can form lumens that can be used to transport blood but lack endothelial cells or other vascular components. Multiple strategies may be in play in a particular tumor, depending on their tumor type or stage. In this review, the authors focus on the major pathways of angiogenesis because its contribution has been applicable to therapeutic intervention.
Growth factors/receptor tyrosine kinases
VEGF Family
The VEGF family is an essential mediator of angiogenesis, which consists of 5 family members of secreted proteins (VEGFA, VEGFB, VEGFC, VEGFD, VEGFE, and platelet-derived growth factor [PDGF]) that bind and activate 3 receptor tyrosine kinases (VEGF receptor [VEGFR]-1, -2 and -3). VEGFA promotes endothelial cell migration, proliferation, vascular permeability, and tube formation. VEGFB was identified as an endothelial cell growth factor expressed in skeletal muscle and heart ; however, its role as an angiogenesis factor is not clearly defined. VEGFC and VEGFD play critical roles in lymphangiogenesis, and their expression has been correlated with the development of lymph node metastases. Placental growth factor (PGF) promotes the survival of endothelial cells and regulates the activity of VEGF signaling.
Regulation of VEGF family gene expression is under the control of stresses, such as hypoxia, acidity, and hypoglycemia, which stimulate transcription and increase mRNA stability, resulting in increased protein expression. Under normoxic conditions, prolyl residues in hypoxia inducible factors (HIF) proteins are hydroxylated by prolyl hydroxylase in a reaction that uses molecular oxygen. Hydroxylation of HIF proteins targets them for ubiquitin-mediated proteolysis. Decreased oxygen concentration reduces the efficiency of this process: HIF proteins are stabilized and become available to bind to hypoxia-response elements in the promoters of target genes, thereby activating transcription.
VEGF proteins bind to receptor tyrosine kinases (VEGFR-1, -2 and -3) that mediate cell signaling resulting in their biologic effects. VEGFR-1 (Flt-1) binds 3 of the VEGF family ligands: VEGFA, VEGFB, and PGF. Activation of VEGFR-1 results in hematopoiesis, embryonic vessel development, macrophage chemotaxis, and recruitment of endothelial progenitor cells to tumor blood vessels from the bone marrow. VEGFR-2 (Flk-1/KDR) is the central mediator of VEGF-stimulated tumor angiogenesis and is essential in embryonic vascular development. When VEGF binds to VEGFR-2, the receptor is phosphorylated and activates downstream signaling molecules, including protein kinase C, phospholipase C, mitogen-activated protein kinase (MAPK), Raf, PI3K, and focal adhesion kinase (FAK) pathways, resulting in endothelial cell migration, proliferation, tube formation, and antiapoptosis. VEGFR-3 binds VEGFC and VEGFD and is involved in the formation of lymphatics in tumors and normal tissue.
A humanized version of a monoclonal antibody to VEGFA, bevacizumab, became the first Food and Drug Administration (FDA)–approved antiangiogenic drug in the United States in 2004. It was approved as a first-line treatment agent for metastatic colorectal cancer, in combination with 5-fluorouracil, and was later approved for the treatment of metastatic non–squamous-cell lung cancer, breast cancer, and glioblastoma multiforme.
Additional FDA-approved drugs that block VEGF signaling are sunitinib and sorafenib, both receptor tyrosine kinase inhibitors that are administered orally. In addition to blocking VEGFR signaling, sorafenib also blocks PDGFRB, FLT3, and KIT signaling. Similarly, sunitinib blocks signaling from VEGFR1-3, PDGFRA, PDGFRB, FLT3, and RET. Sorafenib has been approved for unresectable hepatocellular carcinoma, and advanced renal cell carcinoma, whereas sunitinib has been approved for metastatic renal cell carcinoma, gastrointestinal stromal tumors, and neuroendocrine tumors.
Transforming Growth Factor–β
Transforming growth factor–β (TGF-β) is a paracrine polypeptide with 3 homologous forms (TGFβ1, TGFβ2, and TGFβ3). TGF-β is produced in latent form as a zymogen; after secretion, a latency-associated peptide is proteolytically cleaved to release active TGF-β. Active TGF-β binds to constitutively active type 2 receptors (TGFBR2) to activate type 1 receptors (TGFBR1) in a heteromeric complex that controls transcription through the action of a family of SMAD proteins. TGF-β is a proangiogenic agent despite the fact that, in vitro, TGF-β causes apoptosis and growth arrest of endothelial cells. This paradoxic behavior may be explained by the fact that TGF-β activates the secretion of fibroblast growth factor 2, which acts to stimulate the expression of VEGF. VEGF in turn acts in an autocrine manner through its receptor VEGFR2 to activate the MAPK pathway. However, TGF-β will reverse the protective action of VEGF, promoting apoptosis, which occurs in the pruning process, to form the final vascular network. Therapeutic approaches for targeting TGF-β signaling include antagonism of TGF-β ligand binding to the heteromeric receptor complex with isoform-selective antibodies, such as lerdelimumab (TGF-β 2 ) and metelimumab (TGF-β 1 ) or the pan-neutralizing antibody GC-1008, and intracellular inhibition of the type I TGF-β receptor kinase with small-molecule inhibitors, such as LY550410, SB-505124, or SD-208.
Fibroblast Growth Factor
Fibroblast growth factors (FGFs) maintain endothelial cell function. FGF1 and FGF2 promote endothelial cell proliferation and migration and stimulate angiogenesis. FGFs produce their biologic effects by binding to transmembrane tyrosine kinase receptors, FGFR1 through FGFR4. FGFR can activate Phospholipase C-gamma (PLC-γ), thereby stimulating the production of diacylglycerol and inositol 1,4,5-trisphosphate. This stimulation, in turn, releases intracellular calcium and activates Ca 2+ -dependent PKCs. The activation of the PI3K-Akt cell survival pathway is one of the important biologic responses induced by FGF2 in endothelial cells.
There are several inhibitors of FGF signaling that are undergoing clinical trials. FP-1039 (FGFR1:Fc) is a soluble fusion protein consisting of the extracellular domain of human FGF receptor 1c (FGFR1) linked to the Fc portion of human IgG1. FP-1039 prevents FGFR1 ligands from binding to any of their related receptors within the family of 7 FGF receptors and may mediate both antitumor and antiangiogenic effects. E-3810 and TKI258 are dual VEGFR and FGFR tyrosine kinase inhibitors in clinical trial.
Notch
The Notch signaling pathway involves gene regulation mechanisms that control multiple cell differentiation processes during embryonic and adult life and is essential for cell-cell communication. This pathway has been directly linked to tumor angiogenesis and to the activation of dormant tumors. VEGFA induces expression of the endothelium-specific Notch ligand deltalike 4 (DLL4): when DLL4 activates the Notch signaling pathway in neighboring cells, it inhibits dorsal sprouting of endothelial tubes. When expressed in tumor cells, DLL4 can activate Notch signaling in host stromal cells, thereby improving vascular function. Inhibition of DLL4-mediated Notch signaling promotes a hyperproliferative response in endothelial cells, a process that leads to an increase in angiogenic sprouting and branching. Despite this increase in vascularity, tumors are poorly perfused, hypoxia increases, and tumor growth is inhibited. Neutralizing anti-DLL4 antibodies have been demonstrated to inhibit tumor growth in vivo. These findings point to the Notch pathway as a potential therapeutic target.
Angiopoietin/Tie Receptors
Angiopoietins belong to a family of endothelial cell-specific molecules that play an important role in endothelial growth, maintenance, and stabilization by binding to Tie receptors. There are 4 types of angiopoietins: Ang-1, -2, -3, and -4. The Tie1 receptor is highly expressed in angioblasts, embryonic vascular endothelium, and endocardium and, in adult tissues, is expressed in lung capillaries. The Tie2 receptor mediates survival signals for endothelial cells resulting in vessel maturation. Ang-2 is an autocrine antagonist that induces vascular destabilization, whereas Ang-1 is an agonist that promotes vessel stabilization in a paracrine fashion. Ang-2 is implicated in tumor-induced angiogenesis and progression and is increased during vascular remodeling. AMG 386 is an investigational peptide-Fc fusion protein that inhibits angiogenesis by preventing the interaction of Ang-1 and Ang-2 with their receptor, Tie2, and is being studied in clinical trials.
Epidermal Growth Factor
The epidermal growth factor (EGF) family consists of 11 members that bind to one of 4 epidermal growth factor receptors (EGFR). All of the receptors, except EGFR3 (HER3), contain an intracellular tyrosine kinase domain. In xenograft models, the activation of EGFR contributes to angiogenesis in addition to cellular proliferation, survival, migration, adhesion, differentiation, and tumor metastasis. The EGFR pathway is more of an indirect regulator of angiogenesis by upregulating the production of proangiogenic factors, such as VEGF. There are 3 FDA-approved EGFR inhibitors: cetuximab and panitumumab, which are monoclonal antibodies, and erlotinib, a tyrosine kinase inhibitor that specifically targets EGFR.
Insulinlike Growth Factor Pathway
The insulinlike growth factor (IGF) pathway plays a major role in cancer cell proliferation, survival, and resistance to anticancer therapies in many human tumors. IGF-1 contributes to the promotion of angiogenesis through increasing VEGF expression via HIF-1a. The 2 main strategies in the development for blocking IGF signaling as an anticancer therapeutic are receptor blockade and tyrosine kinase inhibition. Receptor blockade with the use of monoclonal antibody therapies against the IGF-1R (such as figitumumab) are being investigated. Tyrosine kinase inhibition in general will indiscriminately inhibit the kinase domains of all IGF system receptors. The exception to this is the NVP-AEW541 and NVP-ADW742, which have 15- to 30-fold increased potency for IGF-1R kinase inhibition compared with IR kinase inhibition in cellular assays.
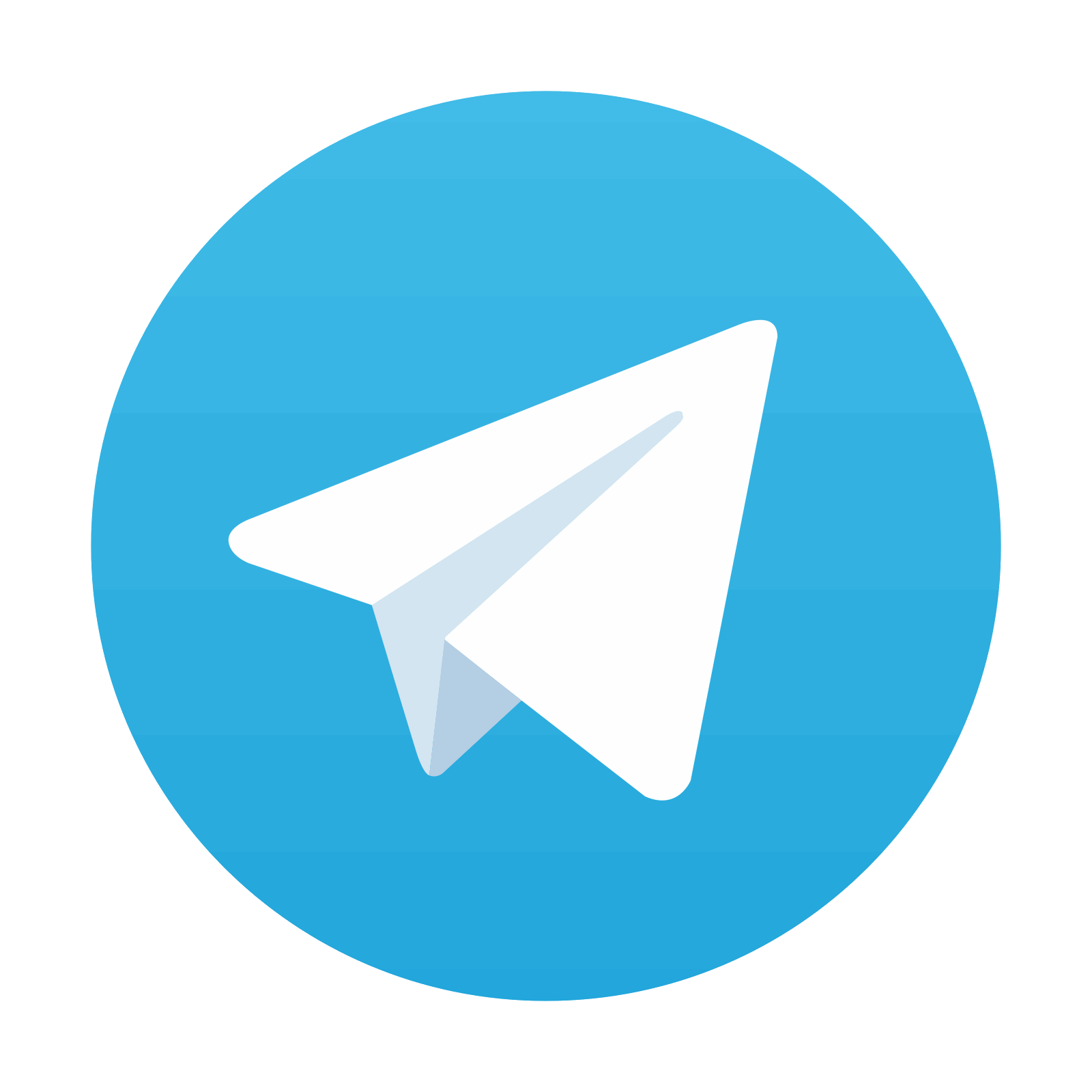
Stay updated, free articles. Join our Telegram channel

Full access? Get Clinical Tree
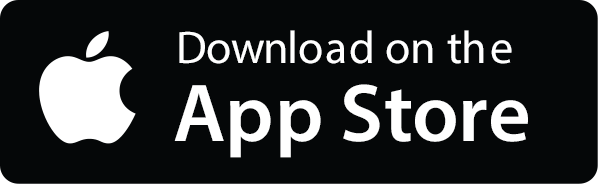
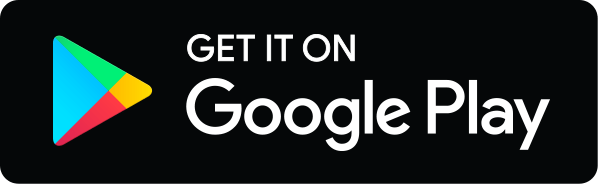