Primary concerns in the clinical utilization of CAR-modified T cells
I. T lymphocyte population selection and culture
(a) Mechanism of T cell activation (OKT3, CD3-CD28 beads)
(b) Cytokines or small molecules included in culture and expansion protocol
(c) Selection of optimal T cell phenotype (Tcm, Tem, Tscm)
II. Gene vector design
(a) Selection of target antigen (both at the epitope and tissue expression levels)
(b) Creation of scFv binding domain
(c) Inclusion of other T cell activation motifs beyond CD3-zeta (CD137, OX40, CD28)
(d) Transient versus permanent gene transduction methodology
(e) Evaluation of the need for a “safety switch” feature
(f) Inclusion of other immunomodulatory transcripts in the vector (co-stim, cytokine)
12.5 Concluding Remarks
The current state of the art in CAR-modified T cell therapy in the clinic is focused on CD19-specific second-generation vectors that encode a 4-1BB (CD137) and CD3ζ-chain signaling package (see NCT02030847 and NCT01626495 at clinicaltrials.gov). Interestingly, because of the high activity of anti-CD19 CARs, the CD28 and CD3ζ-chain signaling package is still highly effective against disease and may be entirely suitable, especially if the patient goes on to HSCT (see NCT01593696, NCT00586391, and NCT00924326). The combination of CAR-based therapy with lymphodepletion or immune checkpoint blockade (such as anti-PD-1 or anti-CTLA4 antibody) demonstrates that we are in a rapidly changing clinical study environment in which new insights towards the effective use of CAR-T cells against hematologic malignancies will continue to develop. In scenarios where immune activity is potentiated, a less active CAR (at least as defined in the laboratory) may be more desirable. Given the rapid translation of CAR-T cell therapy into the clinic, where are the next breakthroughs going to come from? First will be with regard to the viral vector technology. Currently lentivirus-based approaches are state of the art. However, this represents a cost and developmental bottleneck; thus, new transfection-based approaches are awaiting development. Second, the ability to define the most effective CAR-T cell populations with regard to phenotype and the ability to direct their developmental state through cytokines or modification of signal transduction pathways (such as with mTOR inhibitors) will continue to refine current culture techniques and approaches. The goal would be the ability to more rapidly define or create T cell populations that could be infused at lower doses (thus requiring less laboratory effort) while retaining high antileukemic activity. Finally, the demonstration of an effective CAR-based therapy against a solid tumor awaits clinical confirmation. The high degree of normal tissue damage that has been seen in some trials indicates that tissue destruction is indeed possible. However, we do not yet know if it is a paucity of truly tumor-specific cell surface targets or if it the tumor microenvironment that prohibits clinical antitumor effectiveness. The recent opening of a trial featuring a third-generation CAR specific for the pediatric tumor-associated antigen GD2 is of interest in this regard. The retroviral vector used in this trial expresses a GD2-specific binding motif and a combination of CD28, CD3ζ, and OX40 signaling motifs (see NCT01822652). This signaling combination is thought to perform similar to the 4-1BB second-generation vectors, where the anti-apoptotic properties of a TNF-receptor superfamily member (OX40, TNFRSF4, or CD137, TNFRSF9) may enhance survival of the transduced cells once they are infused. This vector also encodes an iCaspase-9 safety gene. If this credentialed tumor-specific anti-GD2 scFv fails to make an impact on disease in a CAR setting, this indicates that engineered T cells alone cannot overcome the solid tumor microenvironment and future successes will hinge on altering this milieu. If the GD2-specific CAR is effective, we will have turned an important first corner in treating solid tumors with engineered T cells.
References
1.
2.
3.
Topalian SL, Hodi FS, Brahmer JR, Gettinger SN, Smith DC, McDermott DF, et al. Safety, activity, and immune correlates of anti-PD-1 antibody in cancer. N Engl J Med. 2012;366(26):2443–54.PubMedCentralPubMedCrossRef
4.
Hodi FS, O’Day SJ, McDermott DF, Weber RW, Sosman JA, Haanen JB, et al. Improved survival with ipilimumab in patients with metastatic melanoma. N Engl J Med. 2010;363(8):711–23.PubMedCentralPubMedCrossRef
5.
Bar M, Sandmaier BM, Inamoto Y, Bruno B, Hari P, Chauncey T, et al. Donor lymphocyte infusion for relapsed hematological malignancies after allogeneic hematopoietic cell transplantation: prognostic relevance of the initial CD3 T cell dose. Biol Blood Marrow Transplant. 2013;19(6):949–57.PubMedCrossRef
6.
7.
Marijt WA, Heemskerk MH, Kloosterboer FM, Goulmy E, Kester MG, van der Hoorn MA, et al. Hematopoiesis-restricted minor histocompatibility antigens HA-1- or HA-2-specific T cells can induce complete remissions of relapsed leukemia. Proc Natl Acad Sci U S A. 2003;100(5):2742–7.PubMedCentralPubMedCrossRef
8.
Nicholls S, Piper KP, Mohammed F, Dafforn TR, Tenzer S, Salim M, et al. Secondary anchor polymorphism in the HA-1 minor histocompatibility antigen critically affects MHC stability and TCR recognition. Proc Natl Acad Sci U S A. 2009;106(10):3889–94.PubMedCentralPubMedCrossRef
9.
Griffioen M, van der Meijden ED, Slager EH, Honders MW, Rutten CE, van Luxemburg-Heijs SA, et al. Identification of phosphatidylinositol 4-kinase type II beta as HLA class II-restricted target in graft versus leukemia reactivity. Proc Natl Acad Sci U S A. 2008;105(10):3837–42.PubMedCentralPubMedCrossRef
10.
11.
12.
Gerdemann U, Katari U, Christin AS, Cruz CR, Tripic T, Rousseau A, et al. Cytotoxic T lymphocytes simultaneously targeting multiple tumor-associated antigens to treat EBV negative lymphoma. Mol Ther. 2011;19(12):2258–68.PubMedCentralPubMedCrossRef
13.
Rosenberg SA, Yang JC, Sherry RM, Kammula US, Hughes MS, Phan GQ, et al. Durable complete responses in heavily pretreated patients with metastatic melanoma using T-cell transfer immunotherapy. Clin Cancer Res. 2011;17(13):4550–7.PubMedCentralPubMedCrossRef
14.
Morgan RA, Dudley ME, Wunderlich JR, Hughes MS, Yang JC, Sherry RM, et al. Cancer regression in patients after transfer of genetically engineered lymphocytes. Science. 2006;314(5796):126–9.PubMedCentralPubMedCrossRef
15.
Gross G, Waks T, Eshhar Z. Expression of immunoglobulin-T-cell receptor chimeric molecules as functional receptors with antibody-type specificity. Proc Natl Acad Sci U S A. 1989;86(24):10024–8.PubMedCentralPubMedCrossRef
16.
17.
Hwu P, Yang JC, Cowherd R, Treisman J, Shafer GE, Eshhar Z, et al. In vivo antitumor activity of T cells redirected with chimeric antibody/T-cell receptor genes. Cancer Res. 1995;55(15):3369–73.PubMed
18.
Altenschmidt U, Klundt E, Groner B. Adoptive transfer of in vitro-targeted, activated T lymphocytes results in total tumor regression. J Immunol. 1997;159(11):5509–15.PubMed
19.
20.
21.
Yun CO, Nolan KF, Beecham EJ, Reisfeld RA, Junghans RP. Targeting of T lymphocytes to melanoma cells through chimeric anti-GD3 immunoglobulin T-cell receptors. Neoplasia. 2000;2(5):449–59.PubMedCentralPubMedCrossRef
22.
23.
Stewart-Jones G, Wadle A, Hombach A, Shenderov E, Held G, Fischer E, et al. Rational development of high-affinity T-cell receptor-like antibodies. Proc Natl Acad Sci U S A. 2009;106(14):5784–8.PubMedCentralPubMedCrossRef
24.
Kershaw MH, Westwood JA, Parker LL, Wang G, Eshhar Z, Mavroukakis SA, et al. A phase I study on adoptive immunotherapy using gene-modified T cells for ovarian cancer. Clin Cancer Res. 2006;12(20 Pt 1):6106–15.PubMedCentralPubMedCrossRef
25.
Lamers CH, Sleijfer S, Vulto AG, Kruit WH, Kliffen M, Debets R, et al. Treatment of metastatic renal cell carcinoma with autologous T-lymphocytes genetically retargeted against carbonic anhydrase IX: first clinical experience. J Clin Oncol. 2006;24(13):e20–2.PubMedCrossRef
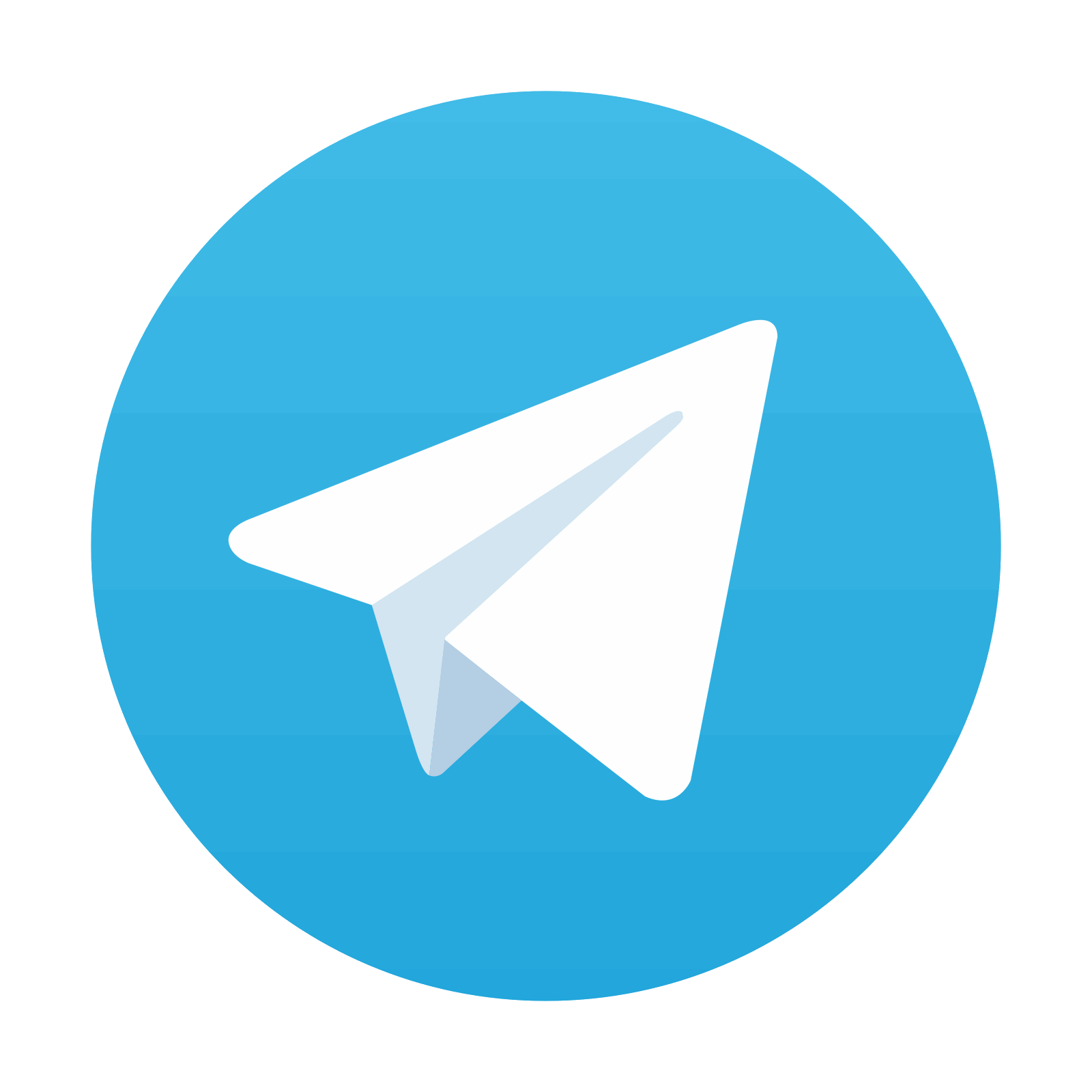
Stay updated, free articles. Join our Telegram channel

Full access? Get Clinical Tree
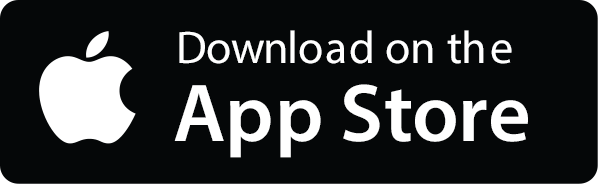
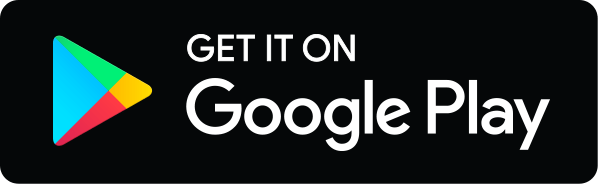