(1)
The Comprehensive Cancer Center of Drum-Tower Hospital, Medical School of Nanjing University & Clinical Cancer Institute of Nanjing University, Nanjing, 210008, People’s Republic of China
14.1 Physiological Drug Resistance (PDR) and the Role of Copolymeric Nanoparticles in Its Reversion
Copolymeric nanoparticles (NPs) have been proven to be effective carriers for the delivery of antitumor agents with some of them have come into clinical use [1–3]. The effectiveness of NPs resides in their enhanced permeability and retention (EPR) effects, rather than on an active targeting strategy [4, 5]. Ultimately, this results in sustained release of drugs from their NP vectors [6]. In this section, we will introduce another important mechanism inherent to copolymeric NPs: their ability to reverse physiological drug resistance (PDR).
Although genetic and epigenetic changes in cancer cells have been a major research focus, the tumor microenvironment is also a vital factor that has attracted increasing attention in recent years. This is because solid tumors are three-dimensional structures composed of both cancer and stromal cells in addition to matrix and vascular network [7]. The physicochemical factors in the tumor microenvironment are quite different from those found in normal tissue. These altered physicochemical factors are significant for the treatment of cancer because they can influence the efficacy of anticancer agents; for instance, the pH of tumor tissue is classic example. The extracellular pH of cancer cells is more acidic than that of normal tissue, whereas the intracellular pH of tumor is nearly equivalent [8]. As to the weakly basic agents that have an acid dissociation constant (Pka) in the range of 7.5–9.5, this unusual intracellular-extracellular pH gradient can influence the effect of these agents. In this scenario, such drugs would be protonated at the extracellular tumor pH value [7]. Since the protonated forms of these agents become much less membrane permeable, they would accumulate predominantly outside of the cell. As such, the intracellular environment would have a low concentration of such weakly basic drugs, leading to a markedly reduced ability to kill cancer cells. Raghunand et al. has defined this phenomenon as “physiological drug resistance”, which is different from the “biochemical drug resistance” (e.g. MDR or drug resistance) that is caused by the changes of signaling pathways and/or protein expression [9].
However, pH is not the solely physiochemical factor influencing drug distribution in tumor tissue. The disorganized vascular network and the absence of a functional lymphatic system of tumor tissue causes increased interstitial fluid pressure (IFP). Moreover, the composition and structure of the extracellular matrix (ECM) can passively influence the movement of molecules within the tumor [10, 11]. Given these additional features of the tumor microenvironment, the delivery of anticancer drugs often fails to reach cells that are distal from functioning blood vessels [12].
With this in mind, the following section will define “physiological drug resistance” (PDR) as any and all kinds of tumor resistance to anticancer drugs that are caused by the physiochemical factors of the tumor itself. This kind of drug resistance is usually composed of two subtypes: [1] “pH-induced physiological drug resistance” (PIPDR), which is caused by the unusual tumor intracellular-extracellular pH gradient and [2] “penetration-defect related physiological drug resistance” (PDPDR), which is caused by the impaired penetration of drugs into the tumor tissue (Fig. 14.1).


Fig. 14.1
Types of drug resistance caused by tumors
PDR plays a significant role in chemotherapy and targeted therapy failure since it is a phenomenon that occurs in most tumors [13]. These different physicochemical factors exist widely in most tumor types, irrelevant to their genetic background. As such, they can influence the effectiveness of a large number of drugs regardless of their mechanism of action. Given this, reversion of PDR would certainly improve drug effectiveness in nearly all kinds of tumors. Unfortunately, very little attention has been paid to this kind of drug resistance. In the following section, we will discuss the two kinds of PDR—PIPDR and PDPDR—in addition to the role copolymeric nanoparticles can play in the reversion of PDR.
14.1.1 PIPDR
Among the various micro environmental factors, pH is of the greatest importance. This is for two reasons: firstly, the lower extracellular pH values are detected in most tumor tissues [13]; secondly, most of the present-day chemotherapeutics for gastric cancer are weakly basic drugs such as doxorubicin, epirubicin, paclitaxel, and docetaxel [14]. Several studies have proven that the cytotoxicity of these drugs is hindered at lower extracellular tumor pH values [9, 14–16].
Raghunand et al. [8, 9, 15, 16] have demonstrated the existence of PIPDR in chemotherapeutics more than 10 years ago. Since its discovery, several attempts have been made to overcome PIPDR. For instance, Lee et al. [17] treated cancer cells with the combination of doxorubicin and either chloroquine (a competing base) or omeprazole (an H+-pump inhibitor). In the study reported by Raghunand et al. [16], the in vivo effectiveness of doxorubicin was enhanced by adding sodium bicarbonate to the drinking water of mice. However, these methods rely on the use of additional agents, or they alter normal homeostasis. Either way, severe side effects may result. Accordingly, most of these treatments failed to attain clinical use because of their inherent or potential toxicity or the likelihood of side effects [17, 18]. The only in vivo study on the reversion of PIPDR was reported by Raghunand et al. [16] in which bicarbonate-induced extracellular alkalinization led to significantly improve the therapeutic effectiveness of doxorubicin against MCF-7 xenografts. However, the potential for side effects is significant since the increase in extracellular pH occurs nonspecifically.
Polymeric NPs are better candidates to overcome PIPDR than other approaches since they are more effective and present with fewer inherent toxicities. NPs enter cells via endocytic uptake, thereby allowing for the intracellular release of their drug cargo [19, 20]. When weakly basic drugs are incorporated into NPs, the drug will be able to effectively enter the cancer cell via endocytosis. The intracellular pH is physiologically normal (around 7. 4), meaning that the basic drugs released in the cytoplasm would keep their active, uncharged forms. Thus, this approach would lead to more effective termination of cancer cells (Fig. 14.2).
Previous work [21] from our lab used a murine model for the study of NP-driven reversion of PIPDR. In this model, tetrandrine (Tet, Pka 7.80), an alkaloid isolated from traditional Chinese medicine, was incorporated into the diblock copolymer methoxy poly(ethylene glycol)-polycaprolactone (mPEG-PCL). In vitro cytotoxicity studies showed that the cytotoxicity of free Tet was significantly decreased (p < 0.05) when the extracellular pH decreased from 7.4 to 6.8. Importantly, the cytotoxicity of Tet-loaded nanoparticles (Tet-NPs) was not significantly influenced by this same change in pH. Our evaluation of the antitumor effects of free Tet versus Tet-NPs in a murine model confirmed the successful reversion of PIPDR by Tet-NPs. Moreover, the NPs reversed in vivo PIPDR more efficiently than other existing methods with meaningfully fewer side effects. Collectively, these results revealed a new mechanism for copolymeric NPs and expanded our understanding of copolymeric drug carriers into an area where cancer microenvironmental factors must be considered.
14.1.2 PDPDR
PDPDR is caused by increased interstitial fluid pressure (IFP) and altered composition and structure of the extracellular matrix (ECM). Taken together, these changes slow down the movement of molecules within the tumor. Many anticancer drugs—including chemotherapeutics and molecular-targeted agents—have limited distribution from blood vessels into solid tumors, which limits their effectiveness [7, 11, 12] (Fig. 14.3).


Fig. 14.3
In vivo distribution of doxorubicin. A section from a mouse mammary tumor showing the distribution of doxorubicin (blue) in relation to tumor blood vessels (red) and regions of hypoxia (green). It is obvious that doxorubicin is distributed around the tumor blood vessels but far away from the hypoxic region of tumors. Bar = 100 μm. Reproduced with permission from Ref. [22]
Traditional methods used to enhance drug penetration into tumor tissue are limited and most have remained at the preclinical stage. These approaches will be described in more detail below.
14.1.2.1 Angiogenesis Inhibitors
Anti-angiogenesis agents (e.g. bevacizumab) directly inhibit vascular endothelial growth factor (VEGF). They have been shown to enhance the clinical effects of chemotherapeutics in several kinds of tumors. This result may appear paradoxical, since the inhibition of angiogenesis would lead to decreases in blood vessel density, thus impairing drug penetration and delivery to cancer cells. However, recent studies have revealed that the mechanisms of angiogenesis therapy act to normalize tumor vascular architecture, thereby improving blood flow to cancer cells and reducing IFP [23–25]. As a result, drug penetration would be improved. It is possible that this is a neglected mechanism for the synergistic effect between chemotherapeutics and angiogenetic agents [26].
14.1.2.2 ECM Modification
The ECM in the tumor microenvironment comprises a diverse network of structural and instructional molecules that is closely linked to the tumor cells [27]. An abnormal extracellular matrix (ECM) is a major factor leading to impaired drug penetration in solid tumors. To this end, a series of approaches have been reported to partially degrade or downregulate the expression of ECM proteins in order to improve drug penetration [28]. The agents used to degrade ECM include collagenase [29, 30], hyaluronidase [29, 31], and matrix metalloproteinase-8 (MMP-8) [32], to name a few. However, these strategies will likely present a double-edged sword, as both MMPs and proteinases play an important role in promoting tumor invasion and metastasis.
Numerous studies have shown that nanoparticles enhance the penetration of their loaded drug agents [33–36], whether alone or in combination with other treatments such as hyperthermia [37], anti-angiogenic agents, or tumor-penetrating peptides. However, nanoparticle penetration is still influenced by the particle size and tumor environment, meaning that multiple biobarriers will need to be overcome before nanomedicines are clinically capable of being delivered to the target site.
Local administration of a nanoparticle-based delivery system results in high drug concentrations and retention times at the tumor site. The clinical benefits of intraperitoneal chemotherapy in advanced stage cancer patients was verified in work that showed local regional chemotherapy improved their clinical outcomes. However, chemotherapeutic efficacy also depends on the accessibility and retention of the delivered drug to tumor tissue. To this end, Saltzman et al. [38] studied the pharmacokinetic and tissue distribution of local polymer implants in the rat brain. They showed that at the end of the first day, therapeutic agent penetration was 5 mm from the site of implantation. From days 3 to 14, therapeutic penetration was reduced to 1 mm. According to rapid in vitro release kinetics, 84% of the drug was cumulatively released from this delivery system during the first 24 h. In the first few days after implantation, the penetration distance of the polymeric drug was reduced since the drug diffusion gradient was significantly diminished. It is possible that intraoperative administration could cause acute injury and enhance drug penetration via convection of interstitial fluid. This phenomenon might also be the reason for the rapid drug elimination seen after day three. It should be noted that the authors did not take into account the effect of interstitial fluid convection to tissue penetration.
After reaching the target site, the cell membrane is an additional barrier to cross in order for efficient delivery of the loaded drug in nanodrug delivery systems (NDDSs) into specific organelles within the cytoplasm of cancer cells. Various strategies have been tried to stabilize lysosomal membrane and prevent lysosomal, such as targeting ligands, antibodies, as well as cell-penetrating peptides (CPPs). Suitable nanoparticle size also influences the penetration property of nanomedicines and affects their cellular uptake. It was found that 30-nm nanoparticles could more easily extravasate and penetrate into tumor tissue when compared with larger size nanoparticles. Moreover, the penetration advantages of smaller nanoparticles exhibited distinct therapeutic effects.
Cell-penetrating peptides (CPPs) have been shown to help polymeric nanoparticles permeate cellular membranes and internalize into cancer cells. For instance, TATp, as a pegylated CPPs, has been used to modify liposomes. The prepared micelles could be efficiently taken up by cancer cells and provided for high transfection productivity in cell nuclei. As a result, TATp improved cytoplasmic drug levels and overcame drug resistance in tumor-bearing mice.
Finally, iRGD is a tumor-specific penetrating peptide that can significantly enhance IP doxorubicin penetration into disseminated peritoneal tumor nodules in mice [39]. Intraperitoneal coadministration of iRGD and doxorubicin specifically labeled suppressed peritoneal metastases in a mouse model. Importantly, iRGD improved intratumoral dextran and doxorubicin concentrations up to 3 and 2.5 times, respectively. When compared with administration of just intraperitoneal doxorubicin, a combination of iRGD and doxorubicin treatment significantly inhibited the growth of peritoneal metastatic tumors and reduced systemic drug toxicity. According to their study, intraperitoneal iRGD and nano drugs were a simple and effective strategy to improve the IP therapeutic index and reduce systemic cytotoxicity for peritoneal carcinomatosis.
14.2 Nanoparticle Applications in Gastric Cancer Treatment
Nanoparticles have inherently adjustable physical properties, which include multivalent targeting abilities, high loading abilities, scalability, easy dispersibility in water, and can pass through biological barriers. As such, further exploration of nanomedical technology could potentially allow for safer and more effective radiographic imaging and detection systems, in addition to more robust early diagnosis and individualized treatments for gastric cancer [40]. More specifically, nanoparticles composed of magnetic iron oxide, magnetic fluorescence, metal, semiconductors, quantum dots, nanodiamonds, nanowires, nanometer polymer, nanocarbon, and graphene have all been used in related medical research fields.
14.2.1 Albumin Nanoparticles
Human serum albumin (HAS) has many medical advantages: good biocompatibility, low toxicity, no immunogenicity, easy preparation, targeted and controlled release, and it can increase drug stability. Given this extensive list, it has drawn considerable attention. In particular, HAS nanoparticles are good drug carriers with the ability to localize within tumor tissues. This would therefore increase the antitumor effects of the loaded drug [41, 42].
Some scholars have used disulfide-PDPH (3-(2-pyridyldithio) propionyl hydrazide) to combine hydrophobic adriamycin (ADR) with HAS. The result has been an amphiphilic adriamycin-human serum albumin nano-micelle ADR-HAS [43] with redox sensitivity. In a nude mouse transplanted tumor models established by NCI-N87 cells, the tumor inhibition rate of ADR-HSA nanoparticles was as high as 69.98%.
14.2.2 NK105
Since paclitaxel (PTX) is hydrophobic, its clinical application depends on its addition to polyoxyethylene castor oil EL. However, this combination will likely lead to allergic reactions [44]. To this end, NK105 is a type of “core-shell” poly-micelle nanoparticle that can “wrap” PTX. It can be directly used for intravenous administration without the addition of further solvents. Therefore, NK105 has a greater clinical advantage than PTX alone [45]. Importantly, Phase clinical study results have shown that the recommended dose of NK105 was 150 mg every 3 weeks, which was equivalent to PTX/m2 [46].
A Phase II clinical study was conducted to assess the efficacy and safety of NK105 in patients with advanced gastric cancer who had failed first-line chemotherapy [47]. All patients included in the study had to have measurable lesions and had to have also undergone chemotherapy without paclitaxel at least once. Every 3 weeks, patients received a 30-min NK105 intravenous infusion (150 mg equivalent to PTX/m2), without allergy-free pretreatment. These infusions occurred until a given patient’s condition improved, until he/she could no longer tolerate the side effects, or he/she refused. The primary end point event was overall response rate (ORR) after baseline. The secondary end point events were progression-free survival (PFS), time to treatment failure (TTF), and overall survival (OS).
From November 2007 to July 2009, 57 patients were included in the study, and 56 of them were evaluated. Two of them achieved complete remission, 12 achieved partial, and the ORR was 25%. The meso-position PFS was 3.0 months, meso-position TTF 2.8 months, and meso-position OS 14.4 months. Drug-associated toxicity went from light to severe, with the following percent of patients reporting the following: neutropenia (64.0%), leukopenia (17.5%), lymphopenia (8.8%), abnormal nerve sensory (1.8%), fatigue (3.5%), and oral cavity inflammation (1.8%). There were no treatment-related deaths.
14.2.3 Chitosan Nanoparticles
Chitosan is a type of natural, linear polysaccharide polymer obtained from a deacetylation chitin reaction. Chitosan has unique properties, including being a biological adhesive, having good biocompatibility and biodegradability, being nontoxic, and having low immunogenicity. As such, it has been widely used in pharmacological and biomedical domains. The existence in amidogen and carboxyl groups in the chitosan molecule makes it easy for chemical modification to occur. Importantly, such modifications can imbue chitosan with amphipathy. The resulting amphiphilic molecules can form self-assembled nanoparticles, which can carry and bring drugs to specific locations. They can then achieve good drug targeting by conjugating specific targeting molecules into the drug-loaded nanoparticles.
N-trimethyl chitosan (TMC) is a type of water-soluble, cationic polyelectrolyte that can be used for oral and intravenous administration of PTX. TMC has biocompatibility and biodegradability properties, and it can form nanoparticles with diameters of 150–200 nm using an ion gelation method [48]. Embedding PTC into TMC can effectively solve the problem of the poor water solubility exhibited by PTX and lead to more controlled release and a prolonged drug half-life. For instance, Song et al. [49] prepared spherical, paclitaxel-loaded, n-trimethyl chitosan chloride TMC-PTX nanoparticles. The loaded amount of PTX in this particle was approximately 30%. TMC-PTX treatment inhibited proliferation and induced apoptosis in NCI-N87 and SGC-7901, two strains of human gastric carcinoma cells. In addition, comparison of TMC-PTX with PTX alone revealed that the former had enhanced therapeutic effects in both NCI-N87 and SGC-7901 types of nude mouse transplanted tumor models. Importantly, there were no evident side effects.
Polyethylene glycol (PEG) is a type of nonionic, hydrophilic polymer that has beneficial characteristics including nontoxicity, no immunogenicity, long circulation times, and good biocompatibility. Therefore, PEG could be widely used in cross-linked polymer combinations. Epigallocatechin-3-gallate (EGCG) is the main component of tea polyphenols found in green tea and has both anti-inflammatory and antioxidant effects. Moreover, it can inhibit tumor growth through anti-angiogenetic effects in addition to inhibiting proliferation and inducing apoptosis [50–54]. Some researchers have made FCS/PCS/Gel/EGCG nanoparticles from fucose-chitosan (FCS), PEG-chitosan (PCS), EGCG, and gelatin [55]. It was found that oral administration of this nanoparticle could effectively reduce drug release in environments containing gastric acid. This led to significant decreases in gastric cancer activity and reductions in inflammatory reactions of the stomach and liver.
14.2.4 Calcium Carbonate Nanoparticles
Nanometer calcium carbonate is a new type of functional, inorganic, and nonmetallic mineral filler that was developed in the 1980s. It is also emerging as a viable gene transfection vector that can be medicated using inorganic nanoparticles. In addition to its easy preparation, good biocompatibility, and biodegradability, it also has a high transfection efficiency and good reproducibility. Furthermore, no buffered solution is needed during the preparative process to control pH [56].
He et al. [57] prepared calcium carbonate (CaCO3) nanoparticles to deliver vascular endothelial growth factor (VEGF) C-siRNA. The diameter of this CaCO3 nanoparticle was 58 mm, and the surface positive charge was +28.6 mV. When compared with lipidosome nanoparticles, the CaCO3 nanoparticles did not show evidence of cytotoxicity to SGC-7901 cells. Additionally, SGC-7901 cells successfully inhibited VEGF-C expression when transfected with CaCO3 nanoparticles loaded with VEGF-C siRNA. In a SGC-7901 cell subcutaneous transplantation tumor model, CaCO3 nanoparticles were also able to effectively inhibit tumor lymphangion genesis and tumor cell growth. Taken together, these results demonstrate that CaCO3 nanoparticles can effectively deliver siRNA nonviral vectors, highlighting their potential use in the genetic treatment of gastric cancer.
14.2.5 Gold Nanoparticles
Gold nanoparticles (GNPs) have very unique physical and chemical properties that are mainly manifested in the following three aspects: (1) gold nanoparticles are relatively safe, easy to prepare, and have good stability, (2) gold nanoparticles are basically inactive and completely nontoxic, and (3) their synthesis is relatively easy and the resulting diameter range controlled within a range of 1–150 nm. (4) They have the same small size, surface, quantum size, macroscopic quantum tunneling, and dielectric effects as those found in nanoparticles. (5) They have unique electrical catalytic, optical, magnetic, and biological affinity effects. Therefore, gold nanoparticles have wide, potential use in the biomedical field. To this end, Singh et al. prepared gold nanoparticles with stable electrical-biological properties. Gold nanoparticles to pH and demonstrated its anti-carcinoma effect inYCC-3 gastric cells [58].
14.2.6 Gelatinase-Targeted Nanoparticles
The matrix metalloproteinases (MMPs) family is an umbrella classification for endopeptidases related to extracellular matrix degradation [59]. Extensive study on MMPs has shown that they are highly expressed in all human tumors. MMP expression levels are related to tumor progression, lower patient survival rate, and tumor metastasis. Gelatinase (GEL), also known as MMP-2 and MMP-9, are components that have been studied the most. Whether in cell or animal models or in clinical observation, MMP-2 plays an important role in tumor growth as well as invading metastasis. Moreover, MMP-9 plays an important role in tumor growth [60], metastasis, and immune adjustment [61–64]. Gelatinase—especially MMP-9—is also an important factor in the tumor metastatic niche. Therefore, treatment strategies targeting MMPs like MMP-2 and MMP-9 are of great significance for the diagnosis and treatment of malignant tumors [65, 66]
To this end, miR-200c is a microRNA capable of inhibiting tumor stem cells. For instance, we [67] adopted a phthalein amine method and a twice-ring opening polymerization and synthesized the macromolecule targeted drug carrier PEG-Pep-PCL that contained a gelatinase substrate section [68]. We also prepared miR-200c single-drug nanoparticles and miR-200c/DOC enzyme-targeted double-drug nanoparticles by using a double-solvent evaporation technique. The transfection efficiency of miR-200c single-drug nanoparticles was found to be high, meaning this approach could improve long-term miR-200c cellular content, reduce TUBB3 expression, and increase sensibilization on DOC. These results showed that the antitumor effect of miR-200c/DOC double-drug nanoparticles was higher than that of DOC single-drug particles.
Work with miR-200c has shown that it can significantly increase the expression of E-cadherin and reduce the expression of the gastric cancer stem cell marker CD44. These findings led to the conclusion that the tumor’s invasive and metastatic abilities were lower than that those of either single-drug particles or Taxotere. A near-infrared live imaging experiment indicated that nanoparticles can localize in the transplanted, subcutaneous sarcoma of human gastric cancer. Laser confocal microscopy also revealed that miR-200c marked by FAM and nanoparticles marked by rhodamine-B both localized around and entered tumor tissue. miR-200c/DOC double-drug nanoparticles have also been shown to effectively inhibit tumor growth. As a result, miR-200c and E-cadherin expression in tumor tissues increased, while TUBB3 and CD44 expression decreased. The proliferative activity of cells was significantly decreased, while apoptosis increased. When the remaining tumor cells were subcutaneously transplanted in a nude mouse model, the growth rate of the second transplanted tumor in the double-drug nanoparticle group had the slowest rate in addition to longest survival time.
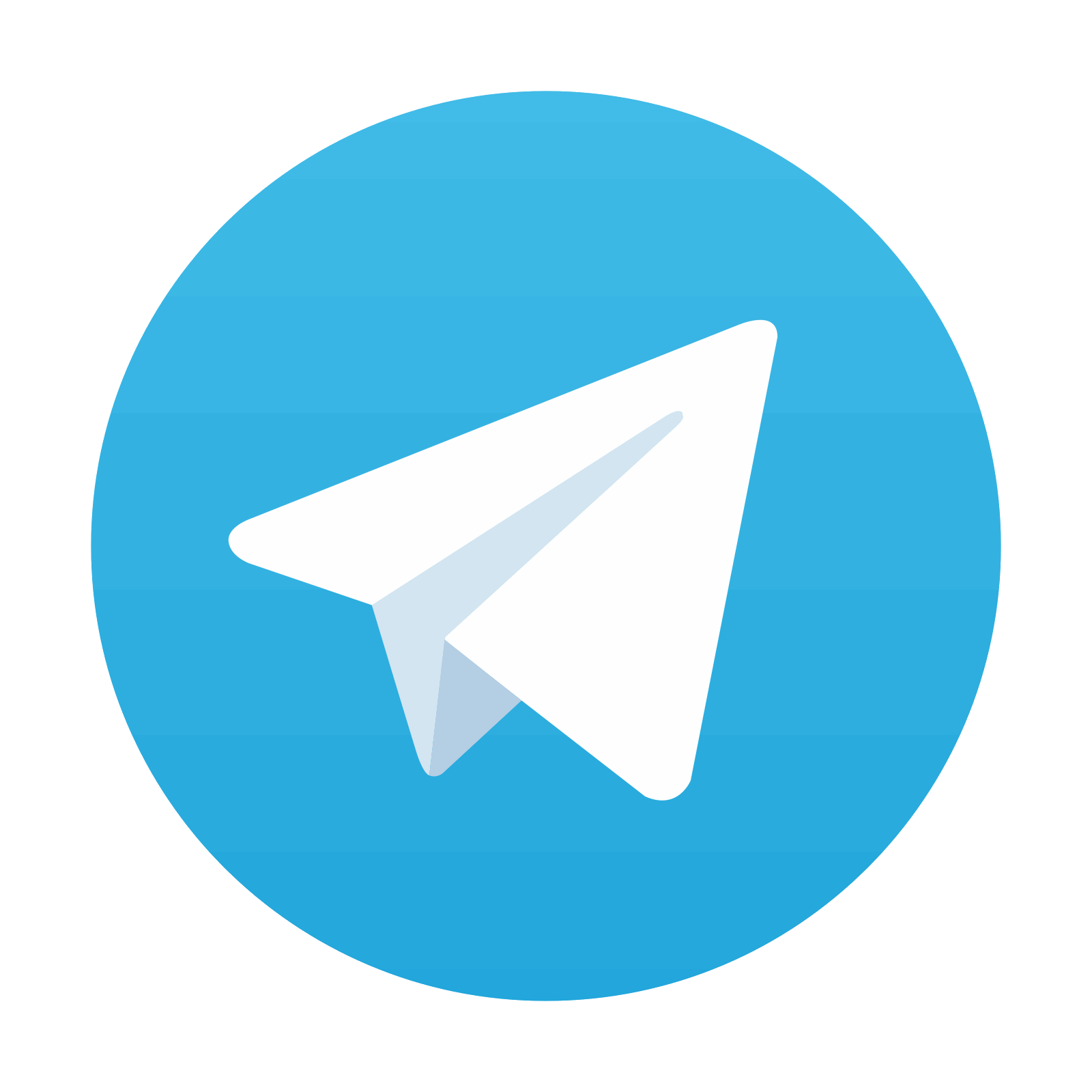
Stay updated, free articles. Join our Telegram channel

Full access? Get Clinical Tree
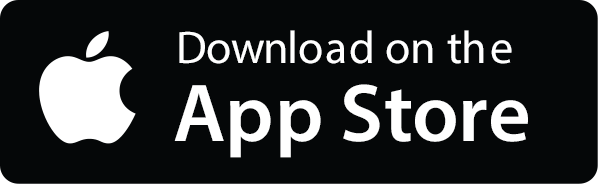
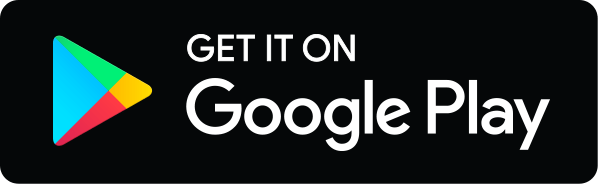