Pulmonary symptoms from advanced-stage lung cancer often require palliative treatments for compassionate patient care. Although many of these symptoms can result from complications of advanced lung cancer treatment regimens (ie, radiation/chemotherapy-induced lung toxicity) or the patient’s underlying comorbid conditions and poor constitution, a significant number of patients have symptoms that originate from the primary tumor itself or from locoregional metastases within the thoracic cavity. These complications from advanced-stage lung cancer can be a serious threat to life and require appropriate intervention.
Airway obstruction
Central airway obstruction refers to significant obstruction of the trachea and main bronchi. Patients with advanced-stage lung cancer who have this complication are likely to have significant dyspnea and hemoptysis, which can become life threatening, because the onset of stridor implies impending airway obstruction and requires immediate attention. Obstructive symptoms can be caused by intraluminal tumor obstruction, extraluminal tumor compression, or both; airway obstruction by the tumor can also produce aggravating factors due to excessive mucous production and plugging, postobstructive pneumonia, and hemoptysis. Appropriate therapeutic strategies must be anticipated to provide palliation and symptomatic relief.
Bronchoscopy remains the single most important diagnostic and therapeutic tool for managing airway obstruction. Several different bronchoscopic interventions are available to palliate airway obstruction in advanced-stage lung cancer. Choosing one bronchoscopic intervention over another is more often determined as much by physician bias as by the cause and anatomic location of the obstruction and patient comorbidities. It is very important to recognize that there are some patients with locally advanced lung cancer involving the central airways who may be amenable to a curative-intent resection involving a tracheoplastic or bronchoplastic procedure. Treatment decisions to offer only palliative endobronchial management in locally advanced lung cancer should be rigorously avoided when potential definitive tracheobronchial resection and reconstruction could be otherwise considered with complete resection. Patients with advanced non–small cell lung carcinoma (NSCLC) with malignant central airway obstruction who undergo local treatment by therapeutic bronchoscopy do not have worse survival than those with advanced-stage lung cancer without airway obstruction. Therefore, therapeutic bronchoscopy should be offered to those patients with advanced-stage NSCLC and airway obstruction as an effective palliative measure.
Patients with symptoms from central airway compromise can be offered several different bronchoscopic options. Tumors that invade into the airway can be treated by mechanical core-out, laser ablation, electrocautery, and dilatation and stent placement. Each of these approaches has the benefit of reliable and immediate improvement in appropriately selected patients. Cryotherapy, photodynamic therapy (PDT), and brachytherapy also are effective for treating endoluminal tumors but usually require several days to achieve a tumor response and so have a more limited role in the management of patients who are acutely symptomatic due to central airway obstruction. Extraluminal compression of central airways by tumor causing severe symptoms is not usually palliated well by techniques other than dilatation and stenting. When therapeutic bronchoscopy is unavailable for patients with impending death from airway compromise, endotracheal intubation passing the tube beyond the area of stenosis with the aid of flexible bronchoscopy can be temporarily lifesaving before the patient is transferred to a referral center.
Bronchoscopic debridement of intraluminal tumor obstruction can quickly and effectively palliate airway obstruction and relieve dyspnea. Rigid bronchoscopy is the preferred technique and is a safe effective method to evaluate and stabilize a patient with airway compromise from tumor invasion or compression. The rigid bronchoscope is much quicker and more effective in accomplishing this task than the flexible bronchoscope. Rigid bronchoscopes are available in different diameters; by maneuvering the bronchoscope beyond the area of obstruction, the patient can be ventilated during the intervention, and further evaluation of the nature of the obstruction can be done concomitantly. Flexible bronchoscopy can be performed through the rigid scope, which allows distal airway evaluation and treatment. In many situations, the act of performing a rigid bronchoscopy dilates the area of obstruction; however, the benefit of this method is brief in cases of obstruction from lung cancer, in which obstruction occurs predominantly from extrinsic compression of the airway. In situations in which there is an endoluminal component to airway obstruction, the rigid bronchoscope is used to core out the obstructing lesion with the beveled tip of the rigid bronchoscope applied at the base of the tumor. Retrieving the pieces with biopsy forceps piecemeal using the biopsy forceps through the rigid bronchoscope can be helpful. The benefit of such a core-out procedure is its technical simplicity and the lack of need for additional equipment beyond the standard instruments used in rigid bronchoscopy. Rigid bronchoscopy also allows access for additional therapies to palliate and alleviate the acute symptoms caused by central airway obstruction from lung cancer and has been shown to be an effective technique to permit immediate discontinuation of mechanical ventilation in patients who had required intubation for airway compromise from lung cancer.
Laser resection, electrocautery, and stenting are therapeutic bronchoscopic techniques that can provide immediate relief from endoluminal airway obstruction and can be used independently or in conjunction with bronchoscopic debridement. Laser resection can be performed via rigid bronchoscopy or flexible bronchoscopy and essentially has the same indications as mechanical core-out procedures for treatment of malignant airway obstruction. Laser resection is not effective in cases of dominant external compression, and a relative contraindication is hypoxemia because the risk of fire limits the inspired oxygen fraction to no greater than 40% fraction of inspired oxygen. When done using flexible bronchoscopy, laser resection using the Nd:YAG laser can be performed under topical anesthetic and is able to reach the lobar orifices more easily than mechanical debridement by rigid bronchoscopy. The main goal of laser resection is palliation of symptoms in patients with inoperable lung cancer with endobronchial manifestations, and, as such, there is little improvement in ventilation with laser resection of obstruction of the segmental bronchi. Laser resection is a substantially longer process than mechanical debridement, requires significant additional equipment and cost, and incurs the additional risks of airway fire, perforation, and fistula formation. In general, there is little practical benefit of laser resection alone over mechanical core-out procedures in cases of malignant endoluminal obstruction, but it is often combined with other treatment modalities, especially as a means of assuring hemostasis.
Electrocautery refers to application of electrical current to produce tissue heating and coagulation as electrons are conducted to the tissue because of voltage differences between the electrocautery probe and the target tissue. Argon plasma coagulation (APC) is also a mode of electrocoagulation. In APC, the ionized argon gas jet flow conducts electrons, allowing a noncontact mode of treatment. Both methods result in coagulative necrosis of the tumor lesion. The therapeutic goal of electrocautery/APC in advanced-stage lung cancer is the same as laser resection and mechanical debridement: to achieve immediate debulking of endobronchial lesions. In contrast to laser resection, electrocautery has a more superficial effect and does not produce deep tissue necrosis, which is preferable if major vessels are in the vicinity. In addition, electrocautery/APC does not have the logistical concerns of laser, such as, special eye protection is not necessary, and it can be performed by flexible bronchoscopy. From a cost standpoint, electrocautery/APC is less expensive to perform than laser resection. The success rate of this technique is comparable to other bronchoscopic debulking techniques, and the technique is on the order of 70% to 80% effective.
PDT, brachytherapy, and cryotherapy have also been used as palliative treatment in patients with advanced-stage lung cancer and obstructive central lesions. However, these techniques have a delay in treatment response and therefore are not suitable in patients with acute obstructive symptoms. Cryotherapy is more limited in use than either PDT or brachytherapy in bronchoscopic palliation of primary lung cancer. All 3 modalities, like the previously discussed interventions, are applicable only to tumors that have an endoluminal component requiring treatment.
PDT is a 2-stage treatment process that produces tumor necrosis by excitation of a chemical photosensitizing agent (eg, porfimer sodium) by a specific light wavelength that matches the absorption band of the agent. The first stage is photosensitization of the bronchial tumor lesion, which is then followed by the illumination stage with exposure of the agent to the light of specific wavelength. At present, Photofrin (Axcan Pharma Inc) is licensed for use in advanced-stage lung cancer by the Food and Drug Administration and is the most commonly used photosensitizer with a long-standing safety record. The recommended dose of the drug is 2 mg/kg body weight and is administered intravenously 24 to 72 hours before the illumination stage. The light that activates the porphyrin-based photosensitizer is within the red region of the light spectrum (630 nm), and different light sources have been used, including argon dye lasers and diode lasers. Because the photosensitizer is only activated in the presence of the laser light source, specific areas for PDT can be targeted. However, indiscriminate illumination could result in collateral damage to normal tissue. The light generated by the laser is delivered to the tumor by optical fibers that are introduced via either the rigid or fiber-optic bronchoscope. The delivery fibers can be either a cylindrical diffuser, which distributes light circumferentially to be used for interstitial treatment when placed within a tumor mass or a microlens delivery fiber, which is designed for surface application. Tissue necrosis and sloughing of tissue occurs within a few days, and a follow-up rigid and flexible bronchoscopy is necessary for removal of the necrotic debris and tissue and for airway clearance, particularly infected secretions collected beyond the bronchial obstruction. Repeat treatment can also be performed after debridement but needs to be performed within the prescribed time (within 7 days of Photofrin injection) to achieve additional tumoricidal effect. Several studies have confirmed the efficacy and safety of PDT procedures for cancer, and, in those with advanced-stage cancer and endoluminal bronchial obstruction, PDT is capable of providing significant palliative improvement and may have a more durable benefit than mechanical debridement or laser resection because of its wider radial effect of tumor necrosis, which may delay tumor regrowth and obstruction. In many cases of NSCLC, patients with inoperable cancer are referred for PDT following recurrence of tumor after chemotherapy/radiotherapy. Although PDT may be an effective palliative therapy, its response is slow and should not be used as the primary intervention for treatment of obstructing central airway lesions with a threatened airway. Also, PDT is associated with considerably greater complexity than the other approaches, which must be considered when PDT is performed in fragile patients with limited life spans. Complications specific to light sensitivity, notably skin burn, can occur, and this skin photosensitivity can last for 4 to 6 weeks during which patients must avoid exposure to sunlight, a significant negative factor for quality-of-life considerations in patients being palliated for cancer.
Endoluminal brachytherapy remains one of the oldest techniques of interventional bronchoscopy, and, like PDT, its effect on tumor debulking is delayed; therefore this technique is not indicated in situations in which immediate tumor debulking for airway obstruction is required. However, brachytherapy can be used to treat airway obstruction caused by extraluminal cancer located immediately adjacent to the airway. The radiation source, iridium 192, is delivered through endobronchial catheters positioned under flexible bronchoscopy, and the therapy sessions can be performed on an outpatient basis with minimal short-term complications. Brachytherapy, therefore, is of benefit, particularly in patients with reduced performance status. The effects of high–dose rate brachytherapy can be initially observed after 1 week, and the peak effect is seen usually after 3 weeks. This technique has the benefit of having a longer-lasting tumoricidal effect than the other described bronchoscopic therapies and can also destroy tumor beyond the bronchial wall. However, brachytherapy only has an effective treatment range of 1 to 2 cm from the endoluminal source and so has no significant impact on bulky tumors involving the airway. Therefore, brachytherapy should not be used to replace standard external beam radiation therapy in most patients. Brachytherapy is primarily used in patients who have already received maximal doses of external beam radiation, and current evidence supports its role as a complementary therapy to palliate patients with airway obstruction rather than as primary therapy alone. The major contraindication for this therapy is the presence or imminent danger of fistula formation between the treated bronchi and other adjacent structures. Complications associated with brachytherapy include radiation bronchitis and stenosis and can occur days or weeks after therapy. The most important adverse effect as mentioned earlier is fatal hemoptysis and bronchovascular fistula formation, which has an overall reported prevalence of 10%.
Cryotherapy offers another modality of treating endoluminal lesions and is based on the cytotoxic effects of cold on living tissue. Cryotherapy tumor destruction relies on the formation of intracellular and extracellular ice crystals, which damage intracellular organelles and cause cellular dehydration. An additional vascular effect occurs: the cold induces an initial tissue vasoconstriction followed by a delayed vasodilation. A zone of local infarct results 6 to 12 hours later. With a 3 mm diameter cryoprobe, the area of destruction is approximately 1 cm in diameter with a depth of 3 mm. Like PDT and brachytherapy, cryotherapy has a delayed effect for tumor destruction, but it differs in that it does not induce collagen damage or risk bronchial wall perforation because collagen and cartilage are quite cryoresistant. Nonhemorrhagic necrosis of the treated lesion occurs 1 to 2 weeks after the procedure, and, generally, the necrotic tissue is expectorated or removed with follow-up bronchoscopy. Flexible cryoprobes are available and can be performed by flexible bronchoscopy; however, the cryoprobes used with rigid bronchoscopy are more powerful and less time consuming to perform. Cryotherapy has been reported to have significant palliative improvement in patients with obstructive airway symptoms from advanced-stage lung cancer, with symptomatic improvement in cough, dyspnea, chest pain, and hemoptysis as well as performance status. Although the application of cryotherapy is less widely applied, it seems as effective as the other palliative methods that use thermal energy, such as laser and electrocautery. The major disadvantage of cryotherapy is that it does require repeated applications for treatment effectiveness and therefore is not ideal for relieving acute dyspnea from airway obstruction.
None of the therapies thus far described are effective for symptomatic airway obstruction caused by extraluminal compression, which represents a high percentage of patients with advanced lung cancer. These patients often have predominant extrinsic airway compression from the disease or have endoluminal tumor obstruction with significant extrinsic compression, and, under these conditions, airway stenting is the only immediate treatment that can provide prompt symptomatic relief. The goals of tracheobronchial stenting in palliation of advanced-stage malignancy are to relieve dyspnea and improve drainage of the obstructed distal lung. Indications for airway stenting in palliation of malignant airway obstruction include the following conditions: (1) extensive tracheal or bronchial compression by the primary lung tumor or central lymph node metastasis without other treatment options, (2) airway stabilization in the acutely symptomatic or compromised patient while the tumor is treated with chemotherapy or radiation therapy, and (3) airway maintenance following tumor debulking by the various therapeutic endoluminal bronchoscopic techniques described earlier. The benefit of stenting is that it provides immediate relief of obstruction, but stenting frequently requires debulking or dilatation of the tumor lesion in order to place the largest-diameter stent possible. The primary disadvantage of airway stenting is impairment of the innate mucociliary clearance mechanisms, which can result in airway obstruction from inspissated secretions, and also obstruction from granulation tissue or tumor ingrowth can become problematic, depending on the type of stent that is deployed. Stent migration and displacement occur but are usually less problematic if an appropriate-sized stent is placed.
There are a variety of commercially available tracheobronchial stents, and a full description of the various types is beyond the scope of this review. However, the selection of the optimal stent should be based on the knowledge of the advantages and disadvantages of the individual types of stent and of their method of deployment and on the anatomic location of the lesion. In general, there are 2 types of airway stents, silicone and expandable metal stents, which can be covered or uncovered. The Dumon or Hood stents are the most widely used silicone stents and come in different diameters and lengths as well as Y configuration for placement in the lower tracheal and carinal region. These stents have external silicone studs or flanges to decrease stent migration and require rigid bronchoscopy and general anesthesia for placement. Intrinsically, silicone stents have smaller inner diameter to outer diameter ratios than metal stents, but silicone stents are relatively less expensive and have minimal tissue reactivity, allowing them to be more easily removed or repositioned.
Self-expanding metal stents have gained in popularity over the years because of their ease of insertion because they can be deployed by flexible bronchoscopy with fluoroscopic guidance and under local anesthesia. Other advantages of self-expanding metal stents include their radiopaqueness, which allows radiographic visualization; the greater airway cross-sectional diameter area due to thinner walls; and the ability of these stents to conform to tortuous airways with minimal migration as the radial expansion force is distributed more uniformly across the stent. The major disadvantage of metal stents is that they are very difficult to reposition or remove and may become secondarily obstructed by tumor ingrowth or granulation tissue. Although the covered metal stents have fewer problems with tumor ingrowth or granulation, it can still occur at the bare metal ends of these stents. Third-generation stents are now available that combine much of the advantages of both expandable and solid silicone stents. These stents are expandable and easier to place yet are completely covered, allowing the option of repositioning or removal (at the cost of more dislodgement) and avoiding the complication of tumor or granulation tissue ingrowth and obstruction.
Airway stenting has been demonstrated to be beneficial in patients with severe symptomatic airway obstruction from advanced lung cancer. Stenting is commonly followed by adjuvant therapy such as radiation and chemotherapy and has been associated with improved median survival. However, airway stenting should also be considered as primary palliative treatment in patients with obstruction of the central airways who lack other treatment options. In one study from the Netherlands, tracheobronchial stenting as part of the palliative care of patients with terminal cancer was associated with immediate symptomatic relief and quality-of-life improvement, which was found to be worthwhile even in these patients who had very limited life expectancies.
In general, a combination of all the earlier-mentioned palliative techniques can be used in a given patient. The choice and sequence of techniques used depend on availability, surgeon expertise, and longevity. Patients are often treated with one modality, receive therapy and stabilize, and then later on develop further symptoms requiring alternative techniques.
Malignant Pleural Effusion
The onset of malignant pleural effusion (MPE) in patients with NSCLC represents a dismal prognosis, with median survival after diagnosis of MPE on the order of 4 to 6 months. The International Association for the Study of Lung Cancer reclassified MPE to the M1a descriptor, recognizing its prediction for poor long-term survival with an overall 5-year survival rate of 7%. Once patients are diagnosed with MPE, treatment strategies for NSCLC shift to a palliative intent, including relief of effusion-related symptoms. It becomes imperative that management strategies are aligned with the patient’s informed preferences, which preferably are conducted by a multidisciplinary team that is well versed in all the different palliative modalities that can be offered to these patients.
An important consideration in managing a patient with NSCLC who develops a pleural effusion is that not all effusions are necessarily malignant. Suspected malignant effusions should be confirmed by cytologic tests or pleural biopsy unless there is other compelling evidence of unresectable stage IV disease. Patients with lung cancer and a nonmalignant effusion may still be candidates for curative-intent therapy. However, once diagnosed with an MPE, management should be guided by individualization of care that takes into account clinical factors, including the patient’s functional status and estimated life expectancy, the severity of MPE-related symptoms, and the patient’s preferences regarding the acceptable invasiveness of management options. Several different options are available for treatment of MPE-related symptoms and range from observation with periodic thoracocentesis to pleurodesis to various indwelling drainage catheter techniques.
MPEs from NSCLC generally respond poorly to chemotherapy and radiation therapy and require intervention for management of its related symptoms. MPE from NSCLC generally are associated with shorter survival compared with MPE related to other cancer causes; however, estimated length of survival for individual patients with MPE varies. Prognostic factors that are often used include pleural fluid volume, pleural imaging features, and performance level. High metabolic activity in the pleural fluid determined by fludeoxyglucose F 18 positron emission tomography has been shown to portend poor survival. Performance level has also been correlated with overall survival in patients with MPE and may guide patients to undergo less invasive procedures for management of the pleural effusion. An Eastern Cooperative Oncology Group Performance Status of 2 or 3 has been shown to predict high inhospital mortality in patients who undergo surgical pleurodesis.
Several options exist for management of patients with MPE-related symptoms, and familiarity with the various benefits, risks, and costs associated with different options is necessary to choose the appropriate treatment for each individual patient. Therapeutic large-volume thoracentesis is a useful initial approach for managing lung cancer patients with symptomatic MPEs. Cytologic analysis of the pleural fluid in patients with diagnosed lung cancer can confirm the M1a staging of these patients and therefore alter treatment options. The use of ultrasound guidance is preferred to guide thoracocentesis. Using ultrasound guidance, one can decrease inadvertent injury by localizing the pleural fluid, and this technique can be used to evaluate the degree of lung reexpansion with fluid removal. Therapeutic thoracocentesis is also useful in determining if a patient’s respiratory complaints can be attributed to the effusion: improvement of a patient’s symptoms following large-volume thoracocentesis suggests that more invasive interventions to palliate anticipated recurrence of the MPE can improve the patient’s quality of life. However, if the patient’s respiratory symptoms fail to improve significantly after large-volume thoracocentesis, other causes for the patient’s complaints should be sought and excluded before proceeding with more invasive options to treat the MPE. Generally, we recommend repeat therapeutic thoracentesis of patients with recurrent symptomatic MPEs to individuals with very-poor short-term survival and those in whom pleurodesis or chronic indwelling drainage catheters are not tolerated well.
The goal of pleurodesis is to obliterate the pleural space by producing adhesions between the parietal and visceral pleurae and thereby prevent pleural fluid reaccumulation. A variety of different pleurodesis protocols exist that reflect provider preferences and can range between differences in instillation of sclerosants to differences in surgical approach. No large-scale multicenter trials have compared the efficacy of different pleurodesis protocols. The most common chemical sclerosants used are talc, doxycycline, and bleomycin. Systematic reviews of primary studies indicate that talc is the most effective sclerosant in preventing nonrecurrence of effusions. Concerns regarding the adverse effects from talc limit its use in patients with benign disease, but talc is not generally considered a limiting factor in patients with a malignant effusion. Reported complications include fever, chest pain, empyema, and acute lung injury leading to acute respiratory distress syndrome. Talc-related acute lung injury is thought to be caused by talc’s small particle size, which can be systemically absorbed by vascular beds and thereby cause an inflammatory reaction beyond the pleural space. Studies have reported lung injury from talc instillation in 3% to 8% of patients and increased oxygen requirements in up to 30% of patients. Although the chemical sclerosant for pleurodesis can be instilled at the bedside via tube thoracostomy or by image-guided small-bore catheters, our preference is to perform pleurodesis by video-assisted thoracoscopic (VAT) talc insufflation. The VAT approach has been demonstrated to achieve higher rates of successful pleurodesis when compared with medical pleurodesis at the bedside via a chest tube and has not been associated with increased mortality. Thoracoscopy also allows the surgeon to assess whether there is a chance of reexpansion and pleural apposition. Thoracoscopic talc pleurodesis has been reported to have an overall success rate of approximately 96%.
Although medical pleurodesis is quicker, simpler to perform, and less costly than VAT pleurodesis, we believe that VAT offers significant advantages by allowing more complete pleural fluid drainage, disrupts and lyses pleural adhesions, and ensures even distribution of the talc throughout the pleural surface. Most importantly, VAT allows the surgeon to thoroughly assess the completeness of lung reexpansion before proceeding with pleurodesis. The lung’s ability to fully reexpand and achieve complete pleural apposition is a critical factor in predicting the effectiveness of pleurodesis. In general, we reserve bedside pleurodesis in patients who are unwilling or unable to tolerate general anesthesia and single lung ventilation.
Chronic tunneled indwelling catheters offer an alternative strategy for management of symptomatic MPEs. These catheters can be inserted in the outpatient setting with or without the use of radiologic guidance or as part of the VAT procedure when the underlying lung does not reexpand completely or extensive tumor interposition in the pleural space makes pleurodesis unlikely to be successful. The patient can then intermittently drain pleural fluid in the home setting, and this management strategy offers significant improvement of symptoms. Most patients with these catheters do not require subsequent procedures. The complications associated with these catheters include catheter infection, cellulitis, empyema, catheter obstruction, and reports of tumor tracking along the catheter track. The use of chronic indwelling catheters has traditionally been used in patients with large symptomatic pleural effusions with trapped lungs, those who could not tolerate pleurodesis, or those who had failed previous pleurodesis attempts. However, spontaneous pleurodesis with the use of tunneled catheters has been reported to occur in patients with MPEs and has prompted some providers to offer chronic indwelling catheters as primary therapy for managing MPEs over pleurodesis, citing the multiple benefits of catheters with ease of placement, decreased or lack of need for hospitalization, and immediate relief of symptoms. There are limited data on the cost-effectiveness of treatment of MPEs by indwelling catheters versus talc pleurodesis. One cost-effective analysis model suggested that the use of placement of a chronic indwelling catheter became more cost effective than talc pleurodesis if the life expectancy was 6 weeks or less.
Again, use of throacoscopy can allow an assessment of the potential for reexpansion. If the lung reexpands adequately, then pleurodesis may be preferred. If on the other hand the lung is trapped, the port site can be used as the entry point for placement of an indwelling catheter.
Ultimately, the choice of strategy for managing symptomatic MPEs from lung cancer, pleurodesis or chronic indwelling catheter drainage, should be individualized based on the relative benefits and drawbacks of each option while taking into account patient preferences and priorities. We have found that frequently patients are amenable to either option, and, in these circumstances, we recommend a VAT approach first. Under VAT, we are able to completely drain the pleural effusion, address loculations and adhesions, and thoroughly assess the pleural space. If the lung fully expands, we proceed with talc pleurodesis; however, if there is incomplete lung expansion, we can easily place a tunneled indwelling catheter and the patient does not require additional hospitalization.
Hemoptysis
Hemoptysis occurs in about 7% to 10% of patients who have lung cancer, which most often is mild with only blood-tinged sputum resulting from erosion of small friable mucosal blood vessels; however, hemoptysis is often frightening and anxiety provoking to the patient and family members. Hemoptysis occurs from neovascularization in and around the tumor, tumor necrosis and exfoliation with exposure of underlying blood vessels, trauma from cough or iatrogenesis, and airway-vascular fistula formation. In those patients with advanced stages of bronchogenic carcinoma, severe hemoptysis can be life threatening because of malignant invasion of central pulmonary and bronchial vessels and is associated with a very high mortality. Radiation therapy is effective in treating hemoptysis due to friable tumor and small vessel erosion but obviously is ineffective for major fistula or emergency control of life-threatening hemoptysis. Other treatment options exist for management of nonmassive hemoptysis, including the various therapeutic bronchoscopic techniques previously described, namely, laser coagulation and APC/electrocautery. However, these bronchoscopic interventions are not feasible in the setting of life-threatening hemoptysis.
The management of massive life-threatening hemoptysis takes on greater urgency because the major acute threat to the patient’s life lies in asphyxiation from blood flooding the tracheobronchial tree. The patient needs to be admitted to the critical care unit, and immediate management mandates securing the airway, isolating the bleeding lung, reversing any coagulopathy, and providing fluid resuscitation. Most often in patients with already-known advanced lung cancer, the side of bleeding is presumed, and the patient should be placed with the (tumor) bleeding side down. If the site of bleeding is uncertain, flexible bronchoscopy can be performed urgently to localize the bleeding source once the patient is intubated, preferably with a large-diameter endotracheal tube. A single-lumen cuffed endotracheal tube is generally more beneficial than a double-lumen tube, which is more difficult to place in the proper position and has lumens that are too small in diameter in general to allow adequate toilet bronchoscopy. Flexible bronchoscopy can be undertaken immediately bedside, but it is preferable to perform further bronchoscopic evaluation in the operating room for obvious reasons. One should be prepared to perform both flexible and rigid bronchoscopy. In cases of ongoing massive hemoptysis, performing a rigid bronchoscopy can facilitate suctioning and irrigation of large blood clots. The rigid scope can also be advanced into the nonbleeding side and allow ventilation and oxygenation of the patient as pulmonary toilet of the nonbleeding side is conducted.
Once the airway is secure, temporary hemorrhage control is attempted. A variety of different methods are available, which include iced-cold saline lavage in 50 mL aliquots alternating with dilute epinephrine (1 mL of 1:1000 epinephrine diluted in 10 mL of normal saline) instilled to the bleeding lung side. Topical hemostatic agents have also been applied by bronchoscopic guidance to arrest severe hemoptysis. In one study, 56 of 57 patients who continued to have persistent endobronchial bleeding despite bronchoscopic wedging with iced-cold saline lavage and instillation of vasoconstrictive agents were successfully treated by topical hemostatic tamponade using oxidized regenerated cellulose mesh. If the bronchial site of bleeding can be located, placement of an endobronchial blocker through an endotracheal tube using flexible bronchoscopy can be useful to isolate the bleeding site from further soiling the nonbleeding lung, and this blocker can be left in place in the intubated patient to allow for tamponade of the bleeding. There also has been a successful case report of endoscopic stenting to tamponade bleeding in a patient with central lung cancer presenting with massive hemoptysis.
If temporary control of the bleeding cannot be achieved, the patient can be considered for percutaneous embolization of the bleeding vessel to try to temporize bleeding. Massive hemoptysis into the tracheobronchial tree can arise from 2 vascular systems, the systemic bronchial arteries or the pulmonary artery vasculature, and, therefore if the bronchial arteriography is normal, a pulmonary arteriogram should also be performed. Embolization of the bleeding vessels can be performed using absorbable gelatin sponge and can effectively control bleeding; however, most reports of embolization in massive hemoptysis from lung cancer are limited. Rarely, a central pulmonary artery bleeding source can be controlled by intravascular pulmonary artery stenting. Surgical resection is generally not an option in these patients with advanced stages of lung cancer who present with massive hemoptysis because there is no long-term benefit.
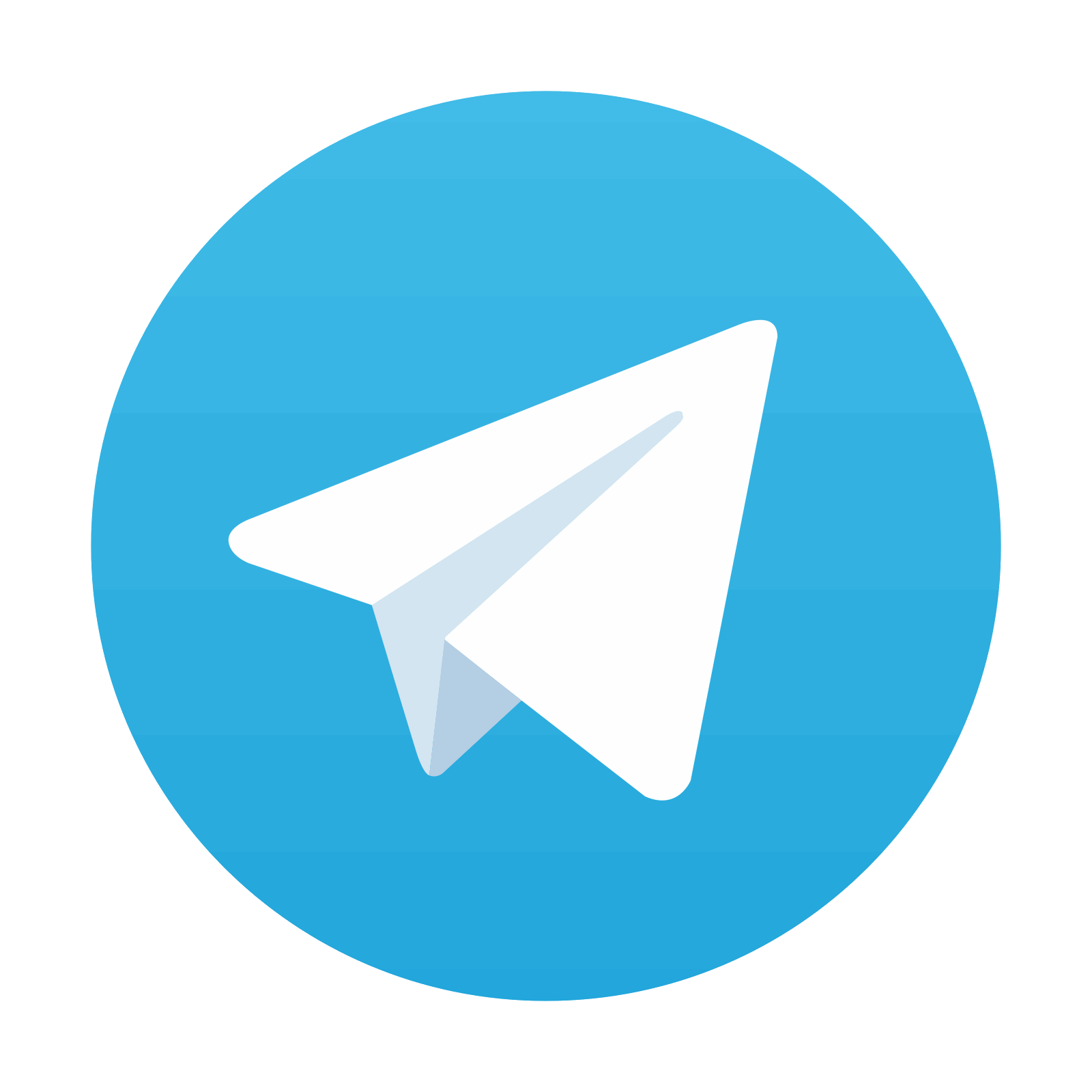
Stay updated, free articles. Join our Telegram channel

Full access? Get Clinical Tree
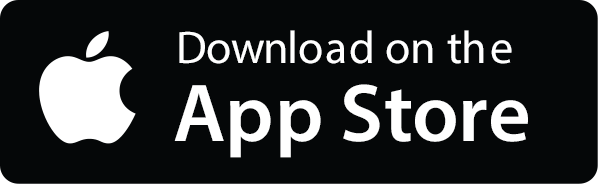
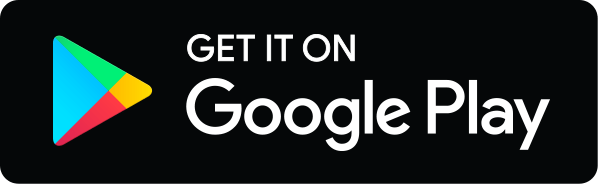