Fig. 23.1
The largest surgical serie of DLGG ever reported, by the French Glioma Network, which demonstrated with an experience of 1097 patients that both the postsurgical residual volume (upper) as well as the extent of resection (lower) were independent prognostic factors significantly associated with a longer overall survival. Modified from [52]
Interestingly, the value of EOR was evident not only within the general hemispheric DLGG population, but also for specific DLGG limited to certain subregions, such as insular DLGG [56, 57].
23.3.3.2 Impact on Malignant Transformation
Such an impact on OS is due to the fact that surgery delayed histological upgrading. It was demonstrated that the volume of residual tumor served as a predictor of malignant transformation [58]. In the recent series by the UCSF group 216 DLGGs, after adjusting for the effects of age, KPS, tumor location and tumor subtype, EOR remained a significant predictor of malignant progression-free survival (HR = 0.983; 95% CI, 0.972 to 0.995; p = 0.005) [39]. In an experience including 191 consecutive patients with DLGGs, Chaichana et al. also showed that gross total resection was an independent factor associated with malignant degeneration (RR 0.526, 95% CI 0.221–1.007, p = 0.05) [59]. Similarly, the French Glioma Consortium recently demonstrated that surgical resection was an independent prognostic factor associated with increased malignant progression-free survival (p < 0.001) [52]. Interestingly, as for OS, the value of EOR on malignant transformation was significant not only for the general DLGG population, but also for specific areas. For instance, the UCSF group showed that, within the insula, the interval to malignant progression of DLGG was longer in patients who had undergone greater resections [56].
Nonetheless, one could argue that the impact of EOR on malignant transformation and OS might be biased by a more favorable molecular pattern in DLGG amenable to a maximal resection. To examine this issue, the predictive value of 1p19q, IDH1, 53 expression and Ki67 index was recently investigated for the EOR in 200 consecutive DLGG patients (2007–2013) [60, 61]. Data were modeled in a linear model. The analysis was performed with two statistical methods (arcsin-sqrt and Beta-regression model with logit link). There was no deletion 1p19q in 118 cases, codeletion 1p19q (57 cases), single deletion 1p (4 cases) or19q (16 cases). 155 patients had a mutation of IDH1. p53 was graded in four degrees (0:92 cases, 1:52 cases, 2:31 cases, 3:8 cases). Mean Ki67 index was 5.2% (range 1–20%). Mean preoperative tumor volume was 60.8 cm3 (range 3.3–250 cm3) and mean EOR was 0.917 (range 0.574–1). Interestingly, the statistical analysis was significant for a lower EOR in patients with codeletion 1p19q (OR 0.738, p = 0.0463) and with a single deletion 19q (OR 0.641, p = 0.0168). There was no significant correlation between IDH1 or p53 and the EOR. Therefore, this study demonstrates in a large cohort of DLGGs that a higher EOR is not attributable to favorable genetic markers. This original result supports maximal surgical resection as an important therapeutic factor per se to optimize prognosis, independently of the molecular pattern [60, 61].
23.3.4 Towards a Supratotal Resection of DLGG
Despite this value of gross-total resection (MRI based) on malignant transformation and survival, a study using biopsy samples within and beyond MRI-defined abnormalities showed that conventional MRI underestimated the actual spatial extent of DLGG, since tumor cells were present beyond the area of MRI signal abnormalities, up to 20 mm—even when gliomas were well defined on MR images [63]. Interestingly, it was suggested that an extended resection of a margin beyond these MR imaging-defined abnormalities might improve the outcome of DLGG. Indeed, an original series reported that a “supratotal” resection—that is, resection extending beyond the area of MR imaging signal abnormalities (Fig. 23.2)—performed in 15 patients bearing a DLGG within “non-eloquent” brain regions avoided malignant transformation in a mean follow-up of 35.7 months (range 6–135) [64]. This series was compared with a control group of 29 patients who had “only” complete resection for a DLGG: malignant transformation was observed in seven cases in the control group but in no cases in the series of patients who underwent supratotal resection (p = 0.037). Furthermore, adjuvant treatment was administrated in 10 patients in the control group compared with one patient who underwent supracomplete resection (p = 0.043). However, 4 of 15 patients with supracomplete resection experienced recurrence [64].
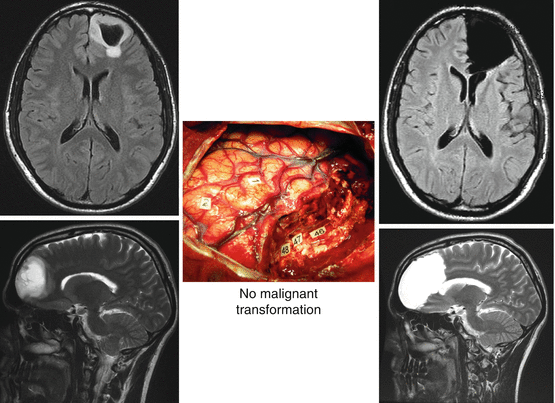
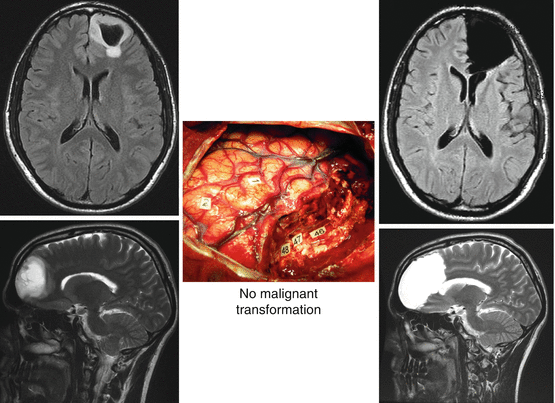
Fig. 23.2
Supratotal resection performed according to functional boundaries detected using intraoperative cortico-subcortical electrical mapping. Left: preoperative MRI showing a left prefrontal DLGG; middle: intrasurgical photograph; right: postoperative MRI demonstrating that the resection was achieved beyond the FLAIR/T2-weighted MRI abnormalities. Modified from [64]
In a more recent series, sixteen consecutive patients who underwent supratotal resection for a DLGG with a long-term follow-up (minimum of 8 years after surgery) have been analyzed [65]. There were seven men and nine women (mean age, 41.3 years, range, 26–63 years) with a glioma (10 right and 6 left tumors) diagnosed because of seizure in 15 cases (one incidental discovery). The resection was continued up to functional cortical and subcortical structures defined by intrasurgical electrical mapping. All patients resumed a normal life after surgery (no neurological deficits, no epilepsy). No adjuvant treatment was administrated after resection. The mean duration of postoperative follow-up was 132 months (range, 97–198 months). There was no relapse in eight cases. Eight patients experienced tumor recurrence, with an average time to relapse of 70.3 months (range, 32–105 months), but without malignant transformation. Five of them have been retreated, with a reoperation (two cases), chemotherapy (three cases) and radiotherapy (two cases). All patients continue to enjoy a normal life. This is the first series demonstrating the prolonged impact of supratotal resection on malignant transformation of DLGG [65].
Nevertheless, the tumor relapse in 50% of cases is probably due to the fact that it was not possible to take at least 20 mm of margin all around the tumor in all patients, due to the functional structures. It is worth noting that some patients had a relapse after only 18 months after supratotal resection, while other had no reccurence with 198 months of follow-up after the sole surgery [64, 65]. This could be explained by the fact that some DLGGs are more “invasive” whereas other DLGGs are more “proliferative”. It is likely that surgery, in particular supratotal resection, in essence has a better chance of controlling the latter than the former. In the future, advances in physiological/metabolic imaging, closer to the neuropathological tumor infiltration as validated by new method of coregistration of histological and radiological characteristics [66, 67], might allow for better selection with respect to indications for supracomplete resection. Another useful method could be the new biomathematical models of proliferation and diffusion, based on at least two sets of MRI acquired 3–6 apart, before any treatment [68] (see chapter by Mandonnet). Therefore, the goal of supratotal resection is currently to avoid malignant transformation for a long period by reducing the number of peripheral tumoral cells and to delay the use of adjuvant therapy, without nonetheless (yet) claiming to cure patients with DLGG—except maybe in some selected cases with a more “proliferative” pattern and with an early diagnosis that permitted to take at least 2 cm of margin all around the glioma [64, 65]. This is the reason why a screening in the general population could be considered, because the rate of supramarginal resection is higher in series reporting removal of incidental DLGG (see the last chapter of this book by Mandonnet et al.) [69].
23.3.5 The Value of Re-operation(s)
Due to the invasive nature of DLGG, relapse is thus possible after total or even supratotal resection, and continuous growth of the residual tumor is ineluctable after incomplete resection. Interestingly, in this setting, some authors provided argumentations in favor of the oncological impact of a second surgery. Schmidt et al. analyzed the surgical results in a series of 40 patients re-operated for recurrent DLGG without other intervening therapy between surgeries. They provided evidence supporting that a gross-total resection was associated with an increased time to repeated surgery [70]. More recently, Jungk et al. confirmed that a greater EOR was prognostic for time to re-intervention (HR 0.23, p = 0.03) [51]. In a series of 130 DLGGs, Ahmadi et al. showed that extended surgical resection for non-malignant relapse (a total resection could be achieved in 53.1% of recurrent tumors) prolonged the OS significantly [44]. In the series reported by the French Glioma Consortium, subsequent surgical resection was an independent prognostic factor significantly associated with a longer OS [52]. Martino et al. also reported a consecutive series of 19 patients who underwent a second surgery for recurrent DLGG in eloquent areas [71]. A total or subtotal resection was achieved in 73.7% of patients during the reoperation, despite an involvement of functional areas. Such “multistage surgical approach”, with an initial maximal function-guided resection, followed by a period of several years, and then a second surgery with optimization of EOR while preserving QoL, is possible thanks to mechanisms of brain plasticity induced both by the tumor (re)growth (see previous chapter by Duffau) as well as by the first resection itself [72, 73]. In this series, the median time between surgeries was 4.1 years (range 1 to 7.8 years) and the median follow-up from initial diagnosis was 6.6 years (range 2.3 to 14.3 years) with no death during this follow-up period. As a consequence, due to a favorable benefit-to-risk ratio of such strategy, it was proposed to consider reoperation(s) in all recurrent DLGGs. Nonetheless, due to a high rate of malignant transformation histologically proven of 57.9% at reoperation, while the main goal of surgery in (recurrent) DLGG is to prevent degeneration, it was suggested to “over indicate” an early re-intervention rather than to perform a late surgery when histological upgrading already occurred [71].
23.4 The Limited Role of Biopsy in DLGG
Currently, the indications of biopsy are thus very limited in DLGG. First of all, this is due to the fact that by combining clinical and (dynamic) radiological data, the diagnosis of DLGG is typical in the vast majority of cases. Furthermore, the risk of undergrading is very high in biopsy. Indeed, Muragaki et al. have demonstrated that undergrading of WHO grade III gliomas occurred in 28% of cases [74]. This is explained by the huge intra-tumoral heterogeneity of DLGG, with the existence of frequent microfoci of malignant progression in the middle of the tumor [75] (see the chapter by Rigau). Finally, the surgical risk of MR-guided stereotactic biopsies is still around 2% of permanent deficits or death [76]. In practice, this means that beyond patients who don’t want or who are not able to undergo surgical resection for medical reasons, biopsy can be mainly considered in diffuse lesions, such as gliomatosis-like and/or when at least a subtotal resection is not a priori possible [35]. To this end, in addition to the experience of the neurosurgeon, such a prediction of EOR can be optimized by the use of a resection probabilistic map [77] (see the chapter by De Witt Hamer et al.). Such atlas, based on the computation of residual gliomas resected according to functional boundaries in a series of 65 DLGGs, allows a preoperative estimation of the expected EOR with a success rate of 82% [78]. This rationale, directly related to a distinct potential of brain reorganization according to the spatial location of the glioma—in particular with a lesser index of plasticity at the level of the white matter pathways, as detailed in the previous chapter by Duffau [21, 22]—may assist in decisions regarding surgical resection versus biopsy.
23.5 The Paradoxical Negative Effect of Neuroimaging in DLGG Surgery
Despite the valuable help of new methods of non-invasive neuroimaging in the diagnosis of brain tumors, these techniques of oncological and functional imaging have nonetheless also had a paradoxical negative impact on the surgical management of DLGG patients.
Indeed, development of neuroimaging led neurosurgeons to achieve a single tumorectomy according to the oncological limits provided by preoperative or intraoperative structural (in particular T2- and FLAIR-weighted MRI) and metabolic imaging (as perfusion or spectroscopy MRI). However, this principle is not coherent neither with the infiltrative nature of DLGGs nor with the limited resolution of current neuroimaging. This is due to the fact that, despite technical advances, MRI (or even PET scan) still underestimates the actual spatial extent of gliomas, since tumoral cells are present several millimeters to centimeters beyond the area of signal abnormalities [63]. Indeed, it is usually admitted that tumors appear on MRI only for cell densities above 500 cells/mm3 [16]. Therefore, the first risk of oncological imaging, including neuronavigation or intraoperative MRI, is to result in premature interruption of resection [79]. In other words, if the DLGG is located in non-critical area, the current goal for many neurosurgeons is to remove only the MRI-defined abnormalities, without attempting to resect a margin around these signal abnormalities. Yet, as mentioned, recent studies have demonstrated that supratotal resection, i.e. resection beyond the MRI-defined abnormalities, enabled to avoid malignant transformation in DLGG for a long period [65]. Because tumoral cells have been identified in the margin removed around the hypersignal on FLAIR-weighted MRI, this means that resecting only the “tumor visible on MRI” is a loss of chance from an oncological point of view, when supramarginal resection was possible in DLGG involving non-eloquent regions.
On the other hand, from a functional point of view, image-guided resection is not logical in DLGG, knowing that critical structures may persist within the diffuse tumoral disease visible on FLAIR-weighted MRI. In other words, resecting solely the MRI-defined abnormalities for DLGG in eloquent areas does not prevent to generate permanent neurological deficit. Indeed, neurosurgeons have tendency to believe that the data provided by functional MRI (fMRI) and diffusion tensor imaging (DTI) is the “absolute truth” with regard to the investigation the individual functional anatomy of the brain. This is the reason why a large amount of recent experiences is based on the exclusive use of functional imaging for the surgical indications and planning, as well as directly into the operating theater (preoperative data incorporated in a neuronavigational system or intraoperative fMRI/DTI) (for a review, see [80]). Yet, functional neuroimaging is not realiable enough at the individual level to be used in clinical routine [81] (see the next chapter by Duffau). Consequently, there is a risk not to select a patient for DLGG surgery because fMRI activations are visible very near or within the tumor, while it was in fact possible to remove it with no permanent deficit—thus with a loss of chance from an oncological point of view. Furthermore, into the operative room, beyond the risk to damage crucial structures not identified by fMRI/DTI (due to their actual lack of sensitivity) and/or due to the brain shift increasing throughout the resection of voluminous gliomas (thus decreasing the reliability of the data provided by DTI), the dogmatic rule which emerged because of the poor accuracy of these techniques is to take 5–10 mm of margin around the presumed eloquent regions according to functional neuroimaging [82]. Again, such strategy is against the oncological goal, that is, to optimize EOR, whereas it was shown on hundreds consecutive patients with DLGG in critical areas that the resection could be pursued with no margin without increasing the rate of permanent morbidity (less than 2%) [83, 84]. Indeed, Gil Robles and Duffau showed that it was not logical to leave a small amount of tumor involving the cortex when the resection was already performed at the subcortical level into the contact of eloquent white matter pathways (according to the results of subcortical stimulation, see below), because this means that the cortical area not removed was in fact already disconnected and thus not functional anymore (Fig. 23.3) [85].
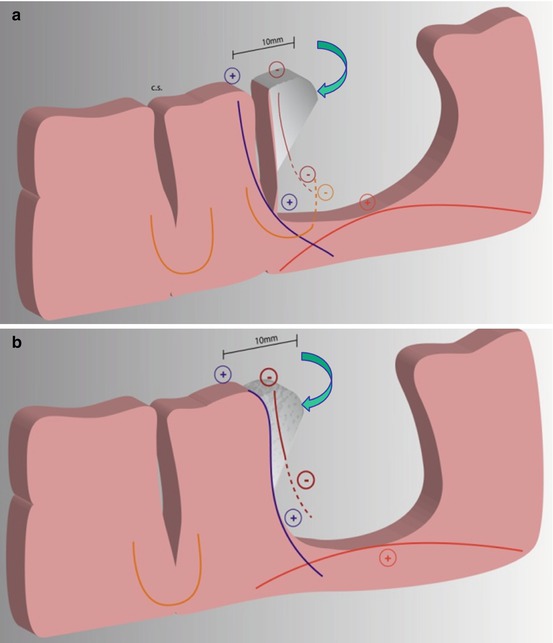
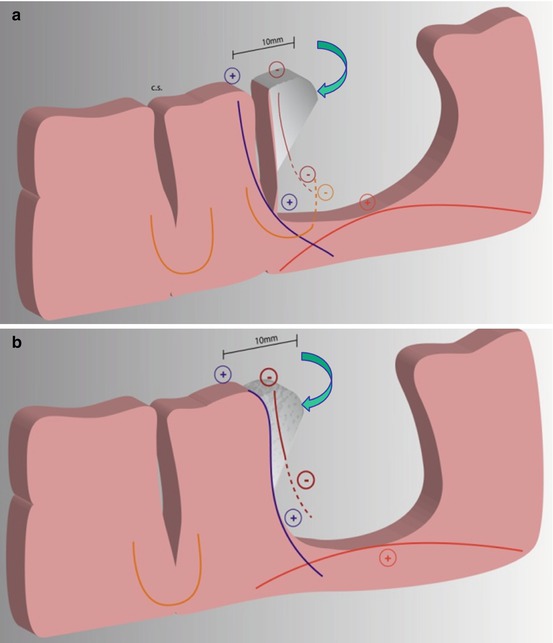
Fig. 23.3
Schematic drawing showing two examples of a resection with margin, which should be avoided. (a) First, a tumor coming into the contact of a sulcus removed by leaving a 10 mm security margin from the positive cortical stimulation site (Blue +) (i.e. with no subpial dissection). If subcortical stimulation are performed, the projections fibers “vertical connectivity” will be identified at the bottom of the sulcus (Blue +, e.g. pyramidal tracts). The U fibers coming from the other side of the sulcus will also be tested throughout the resection (Orange −). At the bottom of the cavity, the “horizontal connectivity” or long distance assocation fibers (e.g. arcuate fasciculus) will represent the deep functional subcortical boundary (Red +) (no margin left around these tracts). Interestingly, the cortex invaded by the tumor (in grey, shown by the blue arrow) is functionally useless, since the resection has been pursued until the subcortical pathways have been encountered (Blue and Red +). Thus, the fibers arising from this cortex have been disrupted, as they do not respond to stimulation (Brown −). As a consequence, this cortex was disconnected and can therefore be removed with no functional risk. This is the reason why a subpial dissection should have been done since the beginning of the resection (b) The second illustration shows the same example in a case of an “intragyral dissection”. Modified from [85]
In summary, in spite of methodological developments based upon computer-aided surgery, and beyond the fact that these techniques are very expensive and not available in all departments of neurosurgery worldwide, no demonstrations have been made that the benefit-risk ratio of glioma surgery was significantly increased thanks intrasurgical neuroimaging. Indeed, no series using such tools were able to show both an increase of overall survival and a decrease of postoperative morbidity [86]. A single randomized controlled trial has been conducted to investigate the effectiveness of neuronavigation in resecting intracerebral tumors: no rationale for the routine use of neuronavigation to improve the EOR and prognosis has been demonstrated [87]. In the same way, a prospective randomized study using high-field intraoperative MRI in gliomas showed a significant increase of the EOR, but with nonetheless a high rate of new neurological deficits about 13% [88]—which is today not acceptable, because the rate of permanent worsening in series using intraoperative electrical mapping is about 3% [89] (see below). In other words, it is difficult to demonstrate the actual role of intraoperative MRI without comparing the outcomes with a control group based upon intrasurgical electrostimulation, and without evaluating both OS as well as QoL. However, such a study does not exist in the current literature.
23.6 Technical Considerations for Maximal Safe Surgical Resection of DLGG
23.6.1 The Conceptual Shift from an Image-Guided Surgery Towards a Functional-Mapping Guided Resection
On the basis of these recent favorable oncological outcomes after DLGG resection, brain surgeons should change their mind, in order to operate the central nervous system involved by a chronic tumoral disease—and not by operating a tumor mass within the brain [1]. The goal is not to content with a single “tumorectomy”, i.e. removal of the part of the glioma visible on neuroimaging, but to perform the most extensive resection of the parenchyma invaded by a DLGG, on the condition that this part of the brain is not essential for cerebral functions. In other words, the neurosurgeon should see first the brain, and not the glioma, to adapt his surgical procedure to the three-dimensional anatomo-functional organization of the connectome for each patient. Thus, both the philosophy and the technique within the central nervous system must be different from the surgical technique outside the brain, because surgical neurooncology is a cerebral networks surgery [90]. Indeed, the main principle in glioma surgery should be to tailor the resection up to individual functional boundaries, with no margin, to maximize the tumor removal while preserving eloquent structures [91]. In this setting, due to the major limitations of oncological and functional neuroimaging (see above), intraoperative electrostimulation mapping is the gold-standard in glioma surgery, because this is currently the sole method able to detect in real-time the cortico-subcortical neural networks crucial for brain functions—which should serve as limits to the resection [18].
Of note, one could consider that both methods, intraoperative MRI and electrostimulation mapping, are not antagonistic and could be combined in the operating theater. In fact, there is a conceptual issue (not a technical one) explaining why imaging-guided resection is incompatible with functional mapping-guided resection. The goal of imaging-guided resection is to remove the signal abnormalities on MRI. In contrast, the principle of functional mapping-guided resection is to stop only when crucial structures have been encountered. In practice, if there are discrepancies in information given by different tools when more than one intraoperative method is used—for example, intraoperative MRI demonstrating tumor but (sub)cortical mapping revealing proximity to functional tissue—the neurosurgeon should rely on stimulation mapping information. In this example, the surgeon should stop the resection to avoid permanent neurologic deficit [79].
23.6.2 Cortico-Subcortical Mapping: The Value of Function-Guided Surgery to Optimize the Onco-Functional Balance (Fig. 23.3)
Here, the goal is not to accurately detail the methodology for awake surgery using stimulation mapping, which will be extensively described in the next chapter—as well as the functional outcome. However, it is seems important to undeline several key issues.
First of all, neurosurgeon has to keep in mind that, in physiology, there is a major interindividual anatomo-functional variability at the cortical level, in particular for cognitive functions such as language [20]—knowing that this variation can be increased in patients with slow-growing DLGG due to mechanisms of neuroplasticity [2, 72]. This means that, even though anatomical landmarks remain important during cerebral surgery, they are definitely not enough.
Therefore, it is crucial to benefit from a positive cortical mapping before to start the resection, in order to tailor it according to the distribution of the neural circuits, in this patient at this time. In other words, one should avoid a false negative (especially for methodological reasons) [84]. Indeed, since eloquent structures can be located within the glioma, the standard surgical principle of debulking tumor from inside to outside is not always safe in DLGG [92]. In the UCSF experience, 4 of 243 (1.6%) patients had a persistent new language deficit: all these four patients had no positive sites detected prior to their resections—“negative mapping” [93]. In another series with 309 patients who underwent surgeries with intraoperative electrostimulation, mapping was found to be negative in 109 cases, and 9% of these patients developed a long-term deficit [94]. Taylor and Berstein previously reported negative mapping in 70% of patients, with 3.6% of them who experienced a permanent neurological worsening [95]. Thus, negative mapping cannot guarantee the absence of eloquent sites and it cannot prevent persistent postsurgical deficit in all cases. This is the reason why other authors continue to advocate a wider boneflap in order to obtain systematic functional responses before the resection, by exposing at least the ventral premotor cortex which will generate articulatory disorders in all cases during stimulation, whatever the side [84, 96]. Of note, by using low intensity of stimulation (mean about 2.25 mA), electrocorticography is not mandatory to obtain a positive mapping and to limit the risk of intraoperative seizures—about 3% in a recent prospective cohort with 374 supratentorial brain lesions, with no aborted awake procedures [84]. In summary, “minimal invasive neurosurgery” means “minimal morbidity” and not “minimal boneflap size”.
Another important issue is the preservation of the subcortical connectivity [1, 2]. Indeed, since DLGG are migrating along main white matter fibers, and because these tracts which subserve the connectome are crucial, as demonstrated using probabilistic atlases of neuroplasticity [21, 22], it is mandatory to identify and to preserve such pathways using subcortical stimulation throughout the glioma removal [18, 83, 90, 96, 97]. As a consequence, beyond electrical mapping, on-line cognitive monitoring should be performed in awake patients (whatever the hemisphere involved by the tumor, see next chapter by Duffau on “Surgery for DLGG: Functional Outcomes”), to check on-line whether no neurological and/or neuropsychological deficits are generated by the resection. To this end, strong real-time relationships are essential between the patient, the neurosurgeon as well as the speech therapist/neuropsychologist directly into the operating theater (see the chapter by Herbet and Moritz-Gasser). On the other hand, due to the fact that the patient can be tired following one to two hours of continuous task, it is recommended to start glioma removal directly into the contact of the eloquent structures detected using cortico-subcortical stimulation mappping, to avoit to waste time, and by disconnecting the part of the brain involved by the DLGG—rather than to “debulk” the tumor from inside and then to come closier to the functional regions only at the end of the resection, when the patient is less cooperative. Indeed, once the invaded brain is disconnected according to the functional boundaries provided by the individual mapping, it is possible to remove it under general anesthesia since the on-line feedback of the patient is not necessary anymore [97].
Interestingly, a recent meta-analysis studying more than 8000 patients who underwent surgical resection for a brain glioma demonstrated that the use of intrasurgical mapping allowed a statistically significant reduction of permanent deficit, despite an increased rate of resection within eloquent areas [77]. In addition, the EOR was increased [77]. These results are in agreement with previous studies which compared both functional and oncological outcomes in two consecutive series of DLGG removed without and then with intraoperative electrical mapping in the same institution [41]. Again, although functional mapping enabled to significantly increase the rate of surgeries within classical “unresectable areas”, the rate of persistent worsening significantly decreased and the rate of total or subtotal resection significantly improved. Indeed, de Benedictis et al. described a series of 9 patients who underwent two consecutive surgeries for a DLGG [98]. The first resection was performed in a traditional way, that is, under general anesthesia and without mapping, whereas the subsequent surgery was done in a maximal way, in awake patients using intrasurgical cortico-subcortical electrostimulation mapping defining the limits of resection. The first tumor removal was subtotal in three cases and partial in six cases, with a postoperative worsening in three cases. Following the subsequent awake surgery, postoperative MRI showed that the resection was complete in five cases and subtotal in four cases (no partial removal) and that it was improved in all cases compared with the first surgery (p = 0.04). There was no permanent neurological worsening. Three patients improved compared with the presurgical status. All patients returned to normal professional and social lives [98]. These original results demonstrate that awake surgery, known to preserve the QoL in patients with DLGG, is also able to significantly improve the EOR for lesions located in functional regions. Furthermore, the group of UCSF demonstrated in a series of 281 patients that the use of functional mapping-guided resection of DLGG in presumed eloquent areas, thanks to a reliable delineation of true functional and nonfunctional regions, allowed not only a maximization of tumor resection but also a significant improvement of long-term survival [99]. Finally, as mentioned, in a recent study, awake surgery was used for DLGG located in non-eloquent areas, with the goal to extend the resection beyond the visible part of the glioma on MRI. Because these “supratotal” resections prevented any malignant transformation in a mean follow-up of 132 months (range, 97–198 months), it means that the concept of functional mapping-resection can also be applied to non-eloquent regions with a significant value on the behavior of DLGG [64, 65].
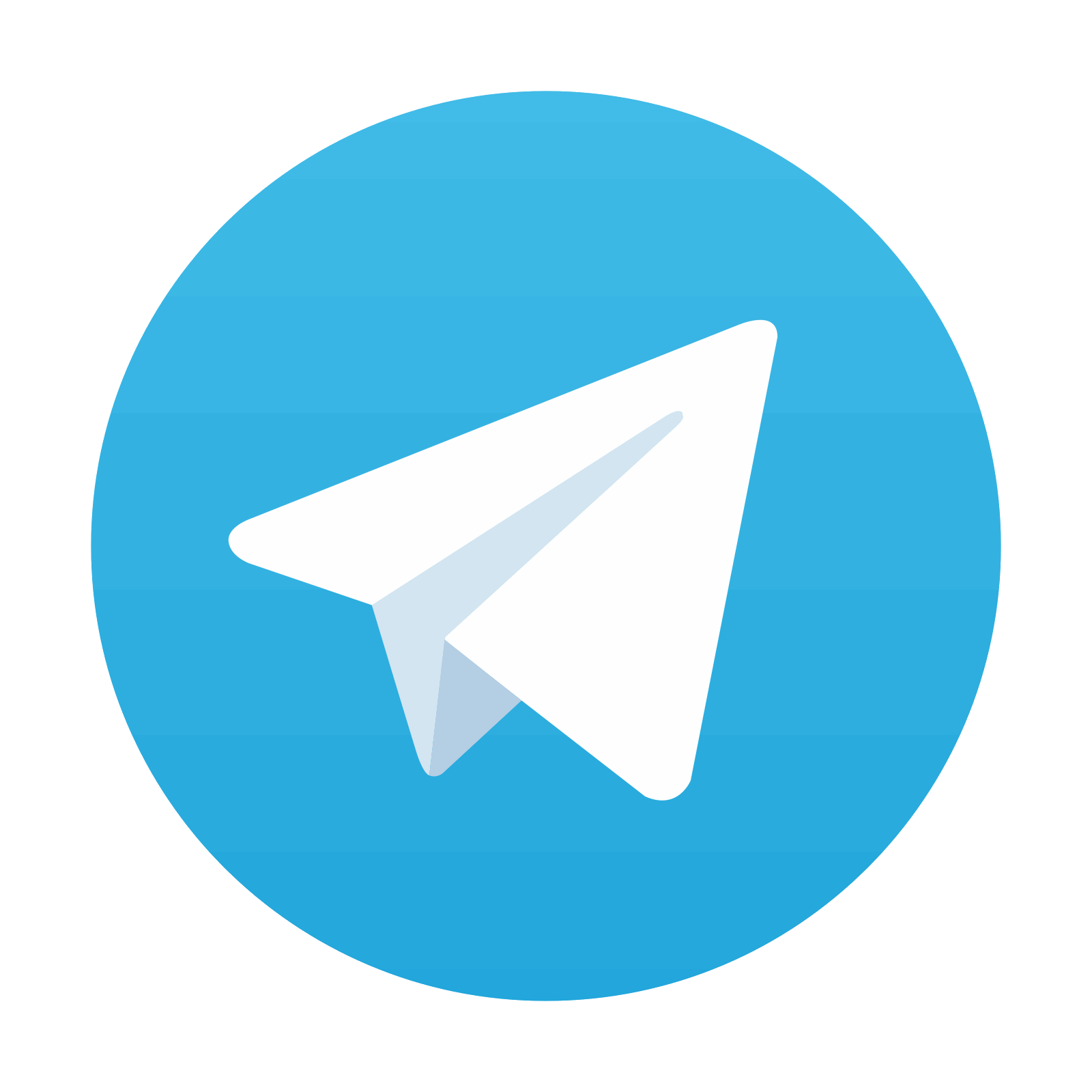
Stay updated, free articles. Join our Telegram channel

Full access? Get Clinical Tree
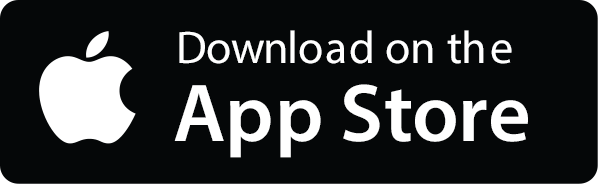
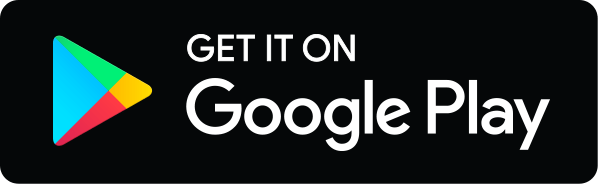