Fig. 24.1
The main association and projection fascicles demonstrated in anatomic dissections were summarized and were colored for an easier recognition. Green = IFOF; Violet = uncinate fascicle; Blue = SLF/AF; Red = cortico-spinal tract; Orange = stratum sagittalis. (Left hemisphere; A, anterior; P, posterior; S, superior; I, inferior). According to this anatomy, the yellow arrow shows one of the major deep functional boundaries during glioma resection within the left frontal lobe, that is, the crossing between the left SLF/AF and the cortico-spinal tract. Modified from [121]
24.5 Mapping the Brain Connectome Applied to Surgical Functional Anatomy in DLGG
An improved knowledge of the connectomal organisation of the central nervous system, breaking with the classical localisationist model, is crucial to optimize the functional outcomes following DLGG resection. In other words, the principle of “hodotopy” is not only an esoteric concept, but also a useful tool for brain surgery, especially in neuro-oncology [122]. Here, the goal is to briefly summarize the different pathways which should be detected as functional limits of resection at the end of surgery for DLGG involving distinct cerebral regions (where DLGG is most frequently located)—in other words, to detail the surgical functional anatomy according to each glioma location.
24.5.1 Precentral-Frontal DLGG (Fig. 24.2)
Posterior limits: pyramidal pathways (with the risk to induce permanent deficit concerning complex movement such as bimanual coordination if the resection is pursued up to this cortico-spinal tract) and/or fibers involved in the control of movement and speech (i.e. fronto-striatal tract and frontal aslant tract in the left “dominant“ hemisphere, respectively) if the patient wants a perfect recovery [84, 87, 91, 92].
Deep limits: convergence of the anterior part of the superior longitudinal fascicle/arcuate fascicle (involved mainly in articulatory/phonological processing in the left hemisphere and mainly in the theory of mind in the right hemisphere, as well as in working memory bilaterally) [45, 76, 105] and of the anterior part of the inferior fronto-occipital fascicle (involved in semantic processing bilaterally, mainly verbal semantics in the left hemisphere and mainly the non-verbal semantics in the right hemisphere) [26, 112]. Of note, for mesio-frontal DLGG (e.g. within the supplementary motor area), the cingulum may be involved in high-level mentalizing [45]. The head of the caudate (involved in executive control) is also an important boundary in the depth [110].
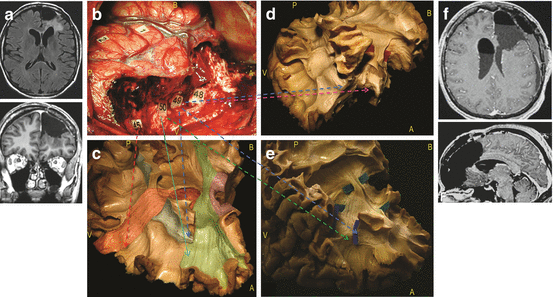
Fig. 24.2
Illlustrative case of resection according to functional boundaries, concerning a patient who underwent a reoperation for a left dominant frontal DLGG (a). The first surgery was performed in another institution under general anaesthesia, achieving a partial resection. A second surgery was performed in our institution under direct brain mapping in awake conditions. The intraoperative picture shows the cortico-subcortical boundaries identified by DES (b). Reproducible speech arrests were induced at the level of the ventral premotor cortex (tags 1, 2). No further functional responses were elicited by stimulations of other frontal cortices, including the so-called Broca’s area. The resection was extended up to the pars opercularis of the inferior frontal gyrus posteriorly, and to the precentral gyrus medially, with preservation of the supplementary motor area. The fronto-orbital and fronto-polar region was removed, reaching the head of the caudate nucleus in the depth. White matter critical components constituted the deep limits of the resection. Perseverations were induced by DES at the level of tag 48, phonemic paraphasias at the tag 49, semantic paraphasias at the tag 50, language and motor arrest at the tag 45 (c–e). The anatomical analysis of the subcortical pathways have been provided according to functional results of the DES in awake surgery. Tag 48, corresponding to the head of caudate nucleus (pink arrow), represents the deep limit of the lobectomy and is identifiable antero-laterally by means of the tip of frontal horn of the lateral ventricle (d); tag 49 refers to the SLF/AF stem running in the depth of the Inferior frontal sulcus (blue tags, blue arrows) (c–e); tag 50 represents the frontal fibers of the IFOF (green tags), crossing the superior longitudinal fascicle at the level of the pars opercularis and triangularis of the inferior frontal gyrus (green arrow) (e); tag 45 are the fibers coming from the supplementary motor area, including the fronto-striatal tract (red arrow) (c). The tumor was radiologically completely removed, as showed by the postoperative MRI (f). The patient fully recovered despite some transitory post-operative language disturbances and resumed a normal socio-professional life. (Left hemisphere; A, anterior; P, posterior; B, base; V, vertex). Modified from [121]
24.5.2 Retrocentral-Parietal DLGG
Anterior limits: somatosensory pathways and/or fibers involved in the control of movement if the patient wants a perfect recovery of bimanual coordination [84, 107].
Deep limits: lateral part of the superior longitudinal fascicle (involved in articulatory process) and posterosuperior part of the arcuate fascicle (involved in phonological processing and repetition, mainly in the left hemisphere) [105, 123]. Importantly, the part II of the SLF is crucial for spatial awareness in the right hemisphere [78]. The optic tracts may also be detected bilaterally, according to the wishes of the patient (who can accept to have visual field deficit—or not) [15, 108]. Of note, for mesio-pariteal DLGG (e.g. within the precuneus), the cingulum may be involved in consciousness [115].
24.5.3 Temporal DLGG
24.5.4 Insular DLGG
Superior and posterior limits: dorsal stream (SLF/AF)
Anterior (in the temporal stem) and inferior limits: IFOF
Deep limits: somatosensory and motor pathways [125]
In all cases, these bundles should constitute the subcortical functional limits of the resection, in particular for large DLGG involving several lobes.
In summary, it is mandatory to map both horizontal cortico-cortical connectivity (long-distance association fibers) as well as vertical cortico-subcortical connectivity (projection fibers), with the goal to preserve the networks underlying the “minimal common core” of the brain [22, 23, 26]. Finally, it is worth noting that, for deep lesions, the shorter trajectory is not always the safer. In some cases, it can be more adapted to select a more complex surgical approach in order to avoid cutting functional pathways, on the basis of the results provided by intrasurgical cortical and subcortical mapping [122].
24.6 Functional Outcomes
In the past decade, brain mapping has led to an impressive improvement of functional and oncological results in DLGG surgery. Because oncological outcomes have already been detailed in the previous chapter by Duffau, here, the aim is to focus on functional results.
24.6.1 Increase of Patients Selection for Surgery in Eloquent Areas
Firstly, patients who were classically not selected for surgery for a long time, on the basis of pure anatomical criteria (e.g. gliomas involving the precentral gyrus or the pars opercularis of the left inferior frontal gyrus), can now benefit from resection with no dogmatic a priori against the surgical feasibility due to the tumor location. In particular, it was demonstrated that the use of intrasurgical DES allowed a significant increase of the surgical indications for DLGG involving so-called “eloquent areas”, when compared with a control group of patients who underwent resection under general anesthesia with no mapping [1, 126]. For example, surgical resection is possible with no permanent neurological worsening for DLGG located within the Broca’s area, Wernicke’s area, insula, and the central region (see previous chapters by Duffau) [17–19, 23, 25–29, 60]. In practice, this means that contra-indication for DLGG resection is essentially represented by very diffuse glioma “gliomatosis-like”, especially when invading massively the subcortical white matter tracts or both hemispheres through the corpus callosum.
24.6.2 DLGG Surgery Preserves and Even Improves Neurological and Cognitive Status
Secondly, despite an increased number of surgeries in critical regions, the rate of permanent neurological deficits is now significantly lesser than in the classical literature, thanks to awake mapping [1, 80]—i.e. less than 2% in the recent series using intraoperative stimulation. Interestingly, this rate of less than 2% of permanent deficits is very reproducible among the teams using awake mapping worldwide [76, 77, 101]. In comparison, in series which did not use awake mapping, the rate of sequelae ranged from 13 to 27.5%, with a mean around 19% (for a review, see [1]). Interestingly, a recent meta-analysis studying more than 8000 patients who underwent surgical resection for a brain glioma demonstrated that the use of intrasurgical mapping allowed a statistically significant reduction of permanent deficit, despite an increased rate of resection within eloquent areas [80]. In addition, the extent of resection was increased [80]. In other words, despite a frequent transitory neurological worsening in the immediate postoperative period—due to the attempt to perform a maximal tumor removal according to cortico-subcortical functional limits using intraoperative mapping, leading to a specific functional rehabilitation (see chapter by Herbet and Moritz-Gasser)—more than 98% of patients recovered the same status than before surgery after glioma resection within eloquent brain areas guided by functional mapping, and returned to a normal socio-professional life [76, 77]. This is also true in very diffuse low-grade gliomas, when surgery was achieved following neadjuvant chemotherapy, that allowed a shrinkage of the peripheral tumoral invasion within the deep connectivity (initially not accessible to resection): global QoL was roughly preserved on the EORTC QLQ C30 + BN 20 (median score: 66.7%) in 10 DLGG patients who benefited from Temozolomide followed by surgery [127].
Of note, these excellent results do not mean that the patients do not experience postoperative subtle cognitive disorders. Indeed, when objective neuropsychological and health related QoL assessment have been performed after surgery, postoperative visuo-spatial, memory, attention, planning, learning, emotional, motivational and behavioral deficits have regularly been observed (for a review, see [128]). Interestingly, a recent study showed that increased reaction time during naming task performed immediately after resection was significantly correlated to return to work [129]. However, one should remind that numerous neurocognitive deficits already existed before surgery, as demonstrated by baseline assessment (see above). Indeed, by comparing cognitive scores before and at 6 months after surgery in a series of DLGG patients, no worsening was found [112]. In the same way, Satoer et al. evaluated the issue of when is the best time to assess neurocognitive function following surgery [130]. These investigators used the same battery of tests before and at 3 months and 1 year following surgery of 45 patients with gliomas located in eloquent regions and compared the results with normal control subjects. The patients with glioma were found to have deficits in all cognitive areas at both baseline and after resection. However, they identified improvement in 2 areas of verbal fluency at the 1-year assessment, supporting both the need for longer duration follow-up of neurocognitive function in these patients as well as the possibility of latent networks of cognition that may be able to be activated over time [130]. Such postoperative improvement is in agreement with the experience reported by Teixidor et al., who performed a longitudinal cognitive investigation, in particular with regard to verbal working memory (vWM), before and after surgical resection of DLGG involving eloquent areas in eight patients [131]. Preoperatively, 91% of patients had vWM disorders. Immediately after surgery, 96% of patients had vWM worsening. At 3 months, following cognitive rehabilitation, among the eight patients examinated, five recovered their preoperative vWM score, and three significantly improved it. Thus, these longitudinal follow-up studies show that DLGG surgery is possible not only without major long-term damage of cognitive functions, but also with an improvement of the preoperative cognitive status [131].
Interestingly, in the series by Satoer et al., extent of resection was no additional risk factor for cognitive outcome [130]. This is in agreement with the study by Jalola et al., who reported that, in long-term survivors with DLGG, an aggressive surgical approach does not lower health-related QoL compared to a more conservative surgical approach [132]. In other words, DLGG with preservation of neural networks does not go against the quality of resection and its impact on the natural history of the disease [133]. Again, this finding supports early and radical safe surgical resection, and pleads against watchful waiting in DLGG.
24.6.3 The Positive Impact of Maximal DLGG on Epilepsy
As mentioned, epilepsy is frequent in DLGG patients, and it significantly impacts QoL: indeed, both epileptic seizure and antiepileptic drugs predispose patients to cognitive impairments [36, 134, 135]. Interestingly, in addition to its impact on survival, complete surgical resection is a predictor of epileptic seizure control, as demonstrated in two monocentric studies of 332 and 508 patients, respectively, and a systematic literature review with meta-analysis, pooling 773 patients from 20 small-sized studies [134, 136, 137]. More recently, in the largest population of 1509 patients ever studied on DLGG in adults, the French Glioma Consortium reported that control of epileptic seizures and their medically-refractory status worsen during the natural course of DLGG; and that seizure control after oncological treatment is related to the extent of surgical resection: indeed, subtotal (P = 0.007) and total (P < 0.001) resections were independent predictors of total epilepsy control [138]. In addition, patients diagnosed with epileptic seizures and those with complete and early surgical resections have better oncological outcomes. Maximal surgical resection is thus required for DLGG, both for oncological and epileptological purposes.
In accordance with an emerging hypothesis regarding glioma-related epileptogenicity, no association between epileptic seizure history and histopathological findings, tumour growth speed and molecular correlates was found, suggesting that glioma-related epileptic seizures may not be triggered by specific intrinsic tumour properties [139]. Conversely, these data also demonstrated that tumour anatomical and functional locations were predictive of epileptic seizure incidence—especially tumor involving the insula or the central region [140]—suggesting that glioma-related epileptic seizures may be triggered by interactions between glioma and neocortex. Electrophysiological recordings and histopathological analyses support this hypothesis by demonstrating that epileptic seizures arise from the peritumoral neocortex and not from the tumour core and that infiltrated isolated glioma cells permeate the peritumoral neocortex [139]. Taken together, these findings support the realization of a supratotal resection (i.e., an extended tumor removal beyond the signal abnormalities on MRI) not only to avoid malignant transformation [8, 9] but also to improve seizure control and thus to optimize the QoL. Indeed, in DLGG patients who benefited from supramaximal resection, the rate of postoperative seizures was nil, with arrest of antiepileptic drugs or at least decrease of doses in all cases [9]. One step forward, Ghareeb et al. demonstrated the significant impact of hippocampectomy in patients with intractable epilepsy generated by a paralimbic DLGG, even if the glioma did not seem to invade the hippocampus on the preoperataive MRI [141]. Indeed, hippocampal resection allowed seizure control in all cases, with an improvement in Karnofsky Performance Scale score since all patients resumed their social and professional activities after surgery—while they were not able to work before surgery [141].
24.7 Future Directions: the Link Between Neuroplasticity and Repeated Resections
In the past decade, many observations of dramatic recovery following massive resection of brain regions invaded by DLGG have been reported [17, 18]. Such functional compensation was attributed to cerebral plasticity, namely, the continuous process allowing short-term, middle-term and long-term remodelling of the neuronosynaptic maps, to optimize the functioning of brain networks (see the chapter by Duffau on “Interaction Between DLGG, Brain Connectome and Neuroplasticity”) [19, 23]. Non-invasive task-based or resting-state functional neuroimaging allows the additional study of mechanisms of reshaping before and after surgical resection [142, 143]. Interestingly, longitudinal studies based on serial functional imaging after surgery showed new degrees of reshaping, as a probable consequence of both tumor removal and personalized postoperative functional rehabilitation. For example, functional neuroimaging performed following the recovery of a transient postsurgical supplementary motor area syndrome showed the compensatory recruitment of the contralesional supplementary motor area and premotor area [142], with changes in the intrinsic connectivity [143]. A “jump” of the perilesional activations was also reported following DLGG resection, for instance from the precentral sulcus to the central sulcus in tumors involving the premotor region, allowing the resection of the “knob of the hand” with no permanent deficit (Fig. 24.3) [144].
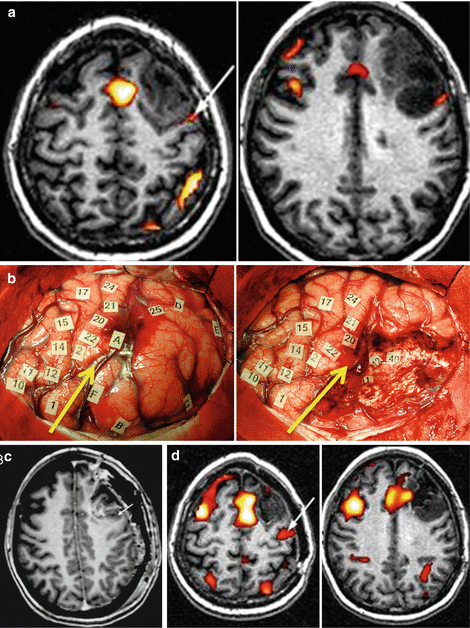
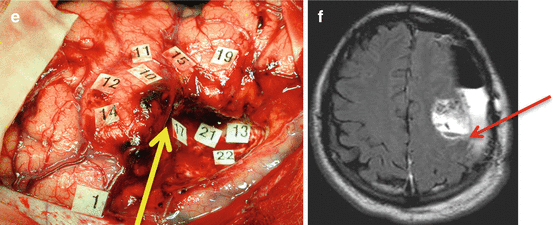
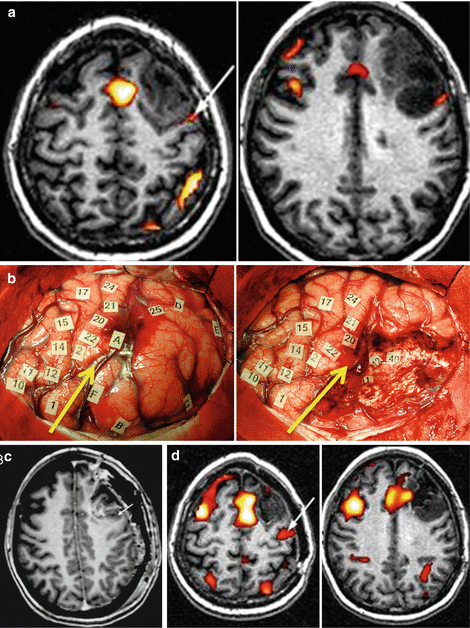
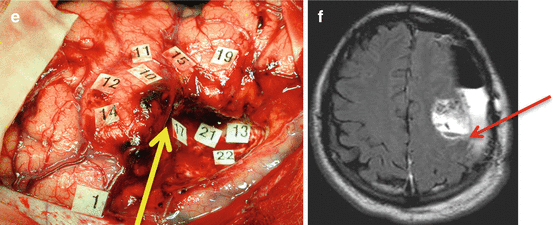
Fig. 24.3
Illustration of the multiple-stages surgical approach (a) Preoperative fMRI in a patient without deficit, haboring a DLGG involving the left premotor area: activation was very close to the posterior part of the tumor, within the precentral sulcus (arrow). (b) Intraoperative views before (left) and after (right) resection of the glioma, delineated by letter tags. DES shows a reshaping of the eloquent maps, with a recruitment of perilesional eloquent sites, allowing a subtotal resection with nevertheless a posterior residue due to invasion of crucial areas (number tags). The yellow arrow shows the precentral sulcus, demonstrating that it was not possible to remove the part of the glioma involving the precentral gyrus (c) Immediate postoperative enhanced T1-weighted MRI showing the residue (arrow), in front of the precentral gyrus (d) Postoperative fMRI 4 years after the first fMRI, demonstrating a recruitment of the controlateral hemisphere, and the posterior displacement of activation previously located at the posterior border of the tumour, now within the central sulcus (arrow) (e) Intraoperative view during the second surgery, confirming the remapping, and allowing a more extensive tumor resection posteriorly, with no permanent deficit. Again, The yellow arrow shows the precentral sulcus, demonstrating that, this time, it was possible to remove a part of the glioma involving the precentral gyrus (f) Immediate postoperative axial FLAIR-weighted MRI (3 h after surgery) showing the improvement of the extent of resection within the left precentral gyrus, thanks to functional reshaping (the red arrow shows the central sulcus). The follow-up is now 11 years since the first surgery, with no reccurrence since the second operation, in a patient enjoying a normal social and professional life. Modified from [144]
This better knowledge of plasticity phenomena has led to propose reoperation(s) when the resection was not complete at the end of the first surgery, due to the involvement of eloquent areas by the tumor. Thanks to functional reshaping (verified using intrasurgical awake mapping), it was possible to increase the extent of resection during a second and even during a third surgery, while preserving brain functions (Fig. 24.3) [144]. Therefore, this multistage surgical approach made possible DLGG removal in critical regions traditionally considered as unresectable, such as the central area, Broca’s area, Wernicke’s area, or the insular lobe [17–19, 23, 25–29, 60, 125].
Recently, such strategy has also been proposed in multicentric DLGG, a rare condition defined as widely separated gliomas in different lobes or hemispheres where there is no anatomical continuity between lesions. In a consecutive surgical series of 5 multicentric DLGGs, Terakawa et al. showed that gross-total or subtotal resection was achieved in all cases, using a single surgery in 3 patients and a 2-stage surgery in 2 patients [145]. Indeed, a single-stage resection of multiple lesions within different lobes may be performed if tumors are located in the same hemisphere. There was no mortality or permanent morbidity associated with surgery. The Karnofsky Performance Scale score ranged between 90 and 100 in all cases. Therefore, the authors concluded that multicentric DLGGs can be removed safely without inducing severe permanent neurological deficits and that surgery should be considered as the first therapeutic option for multicentric DLGG, as in solitary DLGG [145].
However, it is crucial to re-insist of the fact that these extensive resections with no or only slight neurological consequences can be achieved only on the condition that the essential subcortical connectivity is preserved. In other word, as mentioned, this is only possible in a connectomal view of brain organization (i.e. in dynamic and parallel distributed large-scale networks able to compensate themselves) and not in a “localizationnist” framework anymore (one area corresponding to one function) [19, 26].
24.8 Conclusions and Perspectives
Contrary to traditional belief, impaired neurocognitive function is extremely common in DLGG patients, including in cases of incidental discovery, due to the fact that the large-scale functional connectivity is disturbed by tumor growth and migration along the neural pathways. In addition, control of epilepsy and its medically-refractory status worsen during the glioma progression. Altogether, these recent findings plead against a wait and see policy, mainly advocated because of the theoretical risk of surgery. In fact, the QoL of DLGG patients regularly decreases during the natural course of the disease, resulting in more difficulties to restore QoL when surgery is proposed too late—no restoration of brain functions because the limits of brain plasticity have been reached, low chances to control intractable epilepsy.
In this setting, and thanks to recent technical and conceptual advances in brain surgery, the new standard is to achieve early and maximal (possible repeated) surgical resection(s) for DLGG according to functional (and not oncological) boundaries provided by real-time intraoperative neurocognitive monitoring and individual electrostimulation mapping both at cortical and subcortical levels, in a connectomal and plastic framework of cerebral processing. Such an early and radical functional-mapping guided resection has resulted in a preservation and even an improvement of neurocognitive scores as well as QoL of DLGG patients, in particular thanks to seizures control—directly related to the quality of resection. This means that extensive neuropsychological assessment should be achieved in a systematic way before and after each treatment, in particular to adapt a possible specific rehabilitation in the immediate postoperative period. In addition, such dramatic improvement of functional outcome has also led to better oncological results, with an optimization of the extent of resection, enabling a significant delay of malignant transformation and a significant increase of the overal survival (see previous chapter by Duffau). It is worth noting that functional and oncological issues are linked, since a worse QoL is related to a shorter survival.
In summary, early maximal surgical resection should be performed in a systematic manner, both for oncological and functional/epileptological purposes in DLGG—knowing that QoL is also a predictor of overall survival.
Recent stronger interactions between cognitive/behavioral neurosciences (which aim of studying the neural basis of cerebral functions, thanks to a combination between anatomy, functional mapping and cognitive models) and oncological neurosurgery, have participated to overcome the classical dilemma—extent of resection versus brain functions—by giving the possibility to become more ambitious, i.e. to solve the problem of the oncofunctional balance. This is only possible by defining precisely the needs of the patient and his/her family before surgery, in order to tailor the tasks administrated intraoperatively according to the actual QoL balanced with regard to the oncological purpose: for example, to map (or not?) mentalizing in a psychologist with a right temporal DLGG into the contact of the ventral (non-verbal) semantic stream mainly subserved by the inferior fronto-occipital fascicle (see the chapter by Mandonnet and Duffau on “The Oncofunctional Balance”). Of course, the decision should not be taken by the neurosurgeon, but by the patient him(her)self, on the basis of extensive explanations given by the expert team before to go to the operating theater. This means that, in order to counsel optimally the patient, brain surgeons must know the neural foundations underlying brain functions (not only with respect to movement or language, but also concerning higher-order functions as semantic control or theory of mind). In other words, neurosurgeons should be first neuroscientists, to build a “precision neuro-oncological surgery”, based upon prevention and treatment strategies that take individual variability into account—not only by talking about proteomics, metabolomics or genomics [146], but first of all, by taking into account the wishes of the patient, namely, the variability in the philosophy of life.
The next step to open the door to prevention in DLGG is to propose a screening in the general population, allowing very early detection and surgery in incidentally discovered DLGG (see the last chapter by Mandonnet et al.). To this end, determination of a population at-risk in which MRI could be proposed (of course with the consent of the subject) might benefit from advances in the study of genetic risk factors. Indeed, from an oncological point of view, single nucleotide polymorphisms (SNPs) at 8q24.21 have been associated with increased risk of IDH-mutated gliomas [147]. In addition, with respect to functional aspects, accumulating evidence supports the contention that genetic variation is associated with neurocognitive function in healthy individuals and increased risk for neurocognitive decline in a variety of patient populations, including cancer patients. SNPs in genes in metabolism and cognitive pathways have been reported to affect high-order functions in different conditions such as temporal lobe epilepsy. Even subjects with no known neurologic disease perform more poorly on tests of memory and executive function if they are carriers of an “at-risk” variant alleles [148, 149]. For example, the epsilon 4 allele of APOE is associated with increased vulnerability to cognitive decline in brain tumor patients [150]. Recently, Liu et al. have demonstrated that polymorphisms in inflammation, DNA repair, and metabolism pathways are associated with neurocognitive performance (memory, processing speed and executive function) in glioma patients before surgical resection [151]. Such original results could have implications for clinical practice, especially by targeting a subpopulation in which early detection of DLGG might be considered before neurocognitive dysfunction, in order to achieve larger and safer surgical resection.
References
1.
Duffau H, Lopes M, Arthuis F, Bitar A, Sichez JP, van Effenterre R, et al. Contribution of intraoperative electrical stimulations in surgery of low grade gliomas: a comparative study between two series without (1985–96) and with (1996–2003) functional mapping in the same institution. J Neurol Neurosurg Psychiatry. 2005;76:845–51.PubMedPubMedCentral
2.
Smith JS, Chang EF, Lamborn KR, Chang SM, Prados MD, Cha S, et al. Role of extent of resection in the long-term outcome of low-grade hemispheric gliomas. J Clin Oncol. 2008;26:1338–45.PubMed