Type of therapy
Targeted pathway
Achieved effect
Local tumor irradiation
Antigen presentation and processing
Enhanced intratumor homing of effector CTLs
Release of TAAs
Production of inflammatory cytokines
Endothelin receptor blockade
Upregulation of ICAM expression
Chemotherapy
Inhibition of angiogenesis
Taxanes
Induction of programmed cell death
Ab-mediated targeting of CTLs
Tumor and T-cell concomitant antigen binding
Depletion/inactivation therapy
Enhanced activity of intratumor effector CTLs
MDSCs
Inhibition of DNA replication
Tregs
Inhibition of tyrosine kinase signaling
TAMs
Enzyme inhibition
Cytokine therapy
IL-15
T-cell growth factors
IL-7
DCs activation
IL-12
Vaccine adjuvants
Blockade of negative factors
Anti-CTLA-4 (ipilimumab)
Blockade of T-cell checkpoints
Anti-PD1/Anti-LAG3
Inhibition of receptor signaling
Anti-TGF-β
Induction of T-cell activation
Anti CD40/CD40L
5.3.1 Strategies Targeting Homing of Effector T Cells
Some of the tumor immune escape mechanisms described above interfere with proper trafficking of effector T cells from the peripheral circulation or secondary lymphoid organs to the tumor site. A reduced homing of these effector cells to the tumor will give rise to negative regulatory processes leading to tumor progression. Several strategies to block these processes and enhance intratumor homing of effector cells have been proven effective such as local tumor irradiation, blockade of endothelin receptors, taxane-based chemotherapy, and antibody-mediated targeting of effector CTLs.
5.3.1.1 Local Tumor Irradiation
Local tumor irradiation has long been the standard cure for various types of cancer, with the potential to eradicate tumor cells and induce modifications within tumor stroma with an end curative or palliative result, depending on the type and site of disease. Irradiation is frequently used as adjuvant therapy, in association with other therapies such as surgery, hormonal therapy [60], or bone marrow transplantation. Recently, irradiation has come to the attention of tumor immunologists due to its immunogenic properties. One major immunological effect of local tumor irradiation is the release of large amounts of tumor-associated antigens (TAAs) and danger signals that attract immune cells to the tumor site [61]. Furthermore, it has recently been demonstrated that cancer cells which remain after irradiation present high levels of co-stimulatory molecules and MHC components that render them more immune stimulatory. Other beneficial effects of local tumor irradiation are induction of pro-inflammatory cytokines such as TNF-α and IL-1β [62] and adhesion molecules and death receptors that can enhance CTL responses [63]. These changes within the tumor microenvironment seem to facilitate recruitment of effector T cells to tumors via two distinct mechanisms: first, by promoting vasculature normalization [64] and second, by stimulating overexpression of endothelial adhesion molecules, such as vascular cell adhesion molecule 1 (VCAM-1) [65].
The last decade brought forward substantial clinical evidence that local tumor irradiation has the capacity to activate the immune system. A recent clinical study performed on patients with nonmetastatic prostate cancer reported development of treatment-associated autoantibodies in approximately 14 % of patients undergoing external beam radiation therapy, compared with 0 of 14 patients who received radical prostatectomy [66]. To further substantiate this, another study reported that tumor-specific CTLs increase in prostate cancer patients after irradiation [67]. Local tumor irradiation has also been reported to enhance the efficiency of the antitumor treatment of choice. A recent case report of a patient suffering from metastatic melanoma with disease progression on ipilimumab (a monoclonal antibody targeting CTLA-4 activity) indicates a tumor shrinkage and antibody responses to tumor-specific antigens only after treatment with radiotherapy [68].
Taken together, these preclinical and clinical data illustrate that radiotherapy, alone or in combination with other therapies, effectively stimulates the immune system to fight tumor development by facilitating antigen presentation and processing, causing the release of TAAs, increasing production of inflammatory cytokines, chemokines, and receptors involved in the recruitment of effector CTLs, thus enhancing migration of these active effector CTLs to the tumor site.
5.3.1.2 Blockade of Endothelin Receptors
Various studies demonstrate that endothelial cells from a variety of human cancers overexpress endothelin receptors. Blocking these receptors seems a promising strategy to delay tumor development or stop tumor cell proliferation. In fact, selective ETAR blockade by the experimental drug atrasentan has been shown to delay progression of hormone-refractory prostate adenocarcinoma [69] and enhance the effect of paclitaxel/docetaxel used for treatment of prostate cancer [70] in patients. In a mouse model of HPV-induced cervical carcinoma, blockade of ETAR caused inhibition of tumor growth [71]. Although it can be hypothesized that the effect of ETAR blockade on tumor growth is mediated by an increase in T-cell homing to the tumor site, further studies need to be performed to elucidate the underlying mechanisms. On the other hand, in the context of ovarian and also other cancers, overexpression of ETBR was associated with the absence of tumor-infiltrating lymphocytes and short-term patient survival [72]. Moreover, it was shown that interaction between ETBR and its corresponding ligand ET-1 induces downregulation of intercellular adhesion molecule 1 (ICAM-1) expression, a molecule involved in transmigration of lymphocytes into tumors. This effect is rescued by administration of an ETBR small molecule inhibitor BQ-788. Neutralization of ETBR by administration of the inhibitory peptide BQ-788 suppressed intercellular communication and cell growth in melanoma nude mice [71] and significantly increased T-cell homing to tumors [72].
5.3.1.3 Taxane-Based Chemotherapy
Originally, taxanes have been categorized as a class of chemotherapeutic drugs which block tumor development by inducing mitotic inhibition through disruption of microtubule functionality. Other studies suggested towards additional antitumor mechanisms, such as binding to and blocking the functions of the antiapoptotic molecule Bcl-2 expressed on the surface of tumor cells, thus inducing programmed cell death [73]. More recently, the idea of taxanes as enhancers of effector CTL homing into the tumor site came into place. A recent study aimed at investigating whether inhibition of angiogenesis could contribute to overcoming tumor escape from immunity. The results clearly indicated that the angiogenesis inhibitor paclitaxel was capable of increasing leukocyte rolling on the tumor wall vessel and thus infiltration of circulating effector T cells to the tumor [74].
5.3.1.4 Antibody-Mediated Targeting of Effector CTLs
Monoclonal antibody (mAb) therapy is a method most commonly used to functionally inactivate or deplete suppressive immune populations such as myeloid-derived suppressor cells (MDSCs) or regulatory T cells (Tregs). Various studies using bispecific mAb suggest that they can also be successfully used as a tool with antitumor therapeutic application. These antibodies are artificial proteins composed of fragments of two distinct mAbs that can bind to two different types of antigen. For use in cancer immunotherapy, they are engineered to simultaneously bind to a cytotoxic cell (e.g., using a receptor like CD3) and a tumor cell, which needs to be eradicated. Different studies have shown that they display potent in vitro [75] and in vivo [76] effects against tumor cells.
5.3.2 Strategies Targeting the Activity of Effector T Cells
Enhancing intratumor homing of immune effector cells will most likely not be sufficient for an effective tumor control, as cells that do effectively migrate to tumor metastases are often found to be anergic or otherwise dysfunctional. Multiple mechanisms within the tumor microenvironment, as also indicated in the paragraph above, such as MDSCs or Tregs, negative regulatory factors such as CTLA-4, inhibition by ligands such as PDL-1 or factors such as TGF-β, and metabolic deregulation by enzymes such as IDO have all been implicated in generating this immunosuppressive tumor environment. To develop effective strategies to increase activity of intratumor effector T cells, both inhibition of tolerance mechanisms and restriction of tumor-induced immunosuppression should be addressed. To effectively target the above-described negative regulatory mechanisms, several strategies have been studied. Some of the strategies that are most widely studied preclinically as well as in patients will be addressed.
5.3.2.1 Circumventing the Activity of Suppressive Immune Populations: Depletion or Inactivation Therapy
One commonly used mechanism to target innate as well as adaptive immunity that leads to tumor regression [77] is manipulation of the immunosuppressive functions of MDSCs, Tregs, or TAMs. A more intrusive alternative, however extremely efficient, is depletion of suppressive immune populations. Different depletion methods, with specificity for the targeted immune population at hand, have been developed.
Depletion of MDSCs was achieved either by treatment with tyrosine kinase inhibitors, such as sunitinib [78,79], which also induced reversal of Treg elevation or by treatment with inhibitors of DNA replication, such as 5-fluorouracil [80] or gemcitabine [81]. Sunitinib is a broad-spectrum tyrosine-kinase inhibitor capable of inducing selective MDSCs apoptosis in patients with metastatic renal cell carcinoma [82,83]. The reported response rate of sunitinib as a frontline drug is 48 % [83], thus representing one of the most promising drugs for reducing tumor-induced immunosuppression. Examples of chemotherapeutic agents which effectively target the immunosuppressive MDSC populations in preclinical studies include gemcitabine and 5-fluorouracil.
Another immunosuppressive population that has been intensively targeted for improving antitumor response is represented by Tregs. To date, several methods to deplete Tregs have been developed over time. Depletion of CD4+CD25+ Tregs by mAb therapy has been shown in both tumor-bearing animal models and clinical trials [84,85]. In animal models, Treg depletion was obtained by the administration of anti-CD25 mAbs before inoculation of tumor cells [81]. In line with this approach, it was recently reported that selective depletion of FoxP3+ Tregs in transgenic DEREG (depletion of regulatory T cells) mice, in combination with therapeutic immunization against melanoma, greatly enhances the antitumor effect [86]. However, the potency of a combination of immunization and Tregs depletion not only depends on the involvement of Tregs in the tumor model studied but also on the involvement of Tregs induction or activation in the immunization strategy. For example, depletion of Tregs by treatment with an anti-folate receptor 4 antibody did not enhance the immune response induced by immunization with the recombinant viral vector vaccine Semliki Forest virus encoding for the early human papilloma virus (HPV) viral proteins E6 and E7 (SFVeE6,7) in a mouse model of cervical carcinoma [87]. In the clinical setting, one method to deplete Tregs by targeting their high CD25 expression is by employing the immunotoxin denileukin diftitox (OntakTM Ligand Pharmaceuticals), approved for clinical use as therapy against cutaneous T-cell lymphoma [88]. In combination with immunization, it has also been used for the treatment of other types of tumors [89]. Daclizumab (Hoffmann-La Roche) is another anti-CD25 agent, previously used in patients with T-cell leukemia [90,91] and more recently in combination with a peptide vaccine for treatment of metastatic breast cancer [92]. However, anti-CD25 antibodies can also target activated CD25+ effector T cells. Alternatives that circumvent this disadvantage are the use of novel antibodies with human specificity such as anti-glucocorticoid-induced TNF receptor (GITR) antibodies or low doses of Treg-depleting cyclophosphamide [93].
Regarding TAMs, selective depletion is promoted by IL-15/TGF-α in human primary colorectal adenocarcinomas [94]. In other studies, IL-15 has been shown to reverse T-cell anergy [95] and rescue the tolerant phenotype of CD8+ T cells [96]. Although TAMs depletion can be achieved by different approaches, such as blockade of TAMs chemoattractant chemokines (e.g., blockade of CCL-2 with the inhibitor molecule bindarit [47] or immunization with a legumain-based minigene vaccine [97]), the most efficient depletion method in animal models involves the usage of clodronate liposomes. Clodronate liposomes are artificial spheres formed by dispersion of phospholipid molecules into an aqueous solution of clodronate bisphosphonate. Intraperitoneal or subcutaneous administration of clodronate liposomes induced efficient depletion (75–92 %) of TAMs in both murine teratocarcinoma and human rhabdomyosarcoma mouse tumor models [98] and in a mouse model of human cervical carcinogenesis, respectively [99]. Yet it should be noted that nonselective depletion of TAMs also results in the depletion of tumoricidal macrophages, whereby any beneficial effect can be counteracted.
5.3.2.2 Immunostimulatory Cytokines: Cytokine Therapy
In addition to the above-discussed IL-15, various other cytokines are viewed as promising immunorestorative drugs. IL-7, a survival cytokine crucial for T-cell development [100], increases the numbers of peripheral CD4+ and CD8+ T cells in patients [101]. IL-12, a cytokine naturally produced by DCs, is a potent immune adjuvant promoting IFN-γ release from immune cells and thus inducing Th1 polarization and proliferation of antitumor effector T cells [102].
5.3.2.3 Blockade of Negative Regulatory Factors: Antibody Therapy
Antibody therapy against developing tumors has been employed in clinics for many years and belongs to the category of “molecular targeted therapy” of cancer. Despite the emergence of a large palette of monoclonal humanized anticancer antibodies, only a small number are approved for patient use, such as trastuzumab (Herceptin) for HER2-positive breast cancer and rituximab (Rituxan) for B-cell lymphoma. Due to their low toxicity profile and capacity to activate several distinct host effector mechanisms [103], these mAbs are seen as very promising anticancer drugs. The mechanisms most commonly employed by these antibodies are direct interference with tumor cell progression and cell-mediated cytotoxicity by ligation of Fc receptors expressed on the surface of different immune cells [104].
Another antibody that has been very recently approved for treatment of late-stage melanoma is ipilimumab (Yervoy), a human mAb directed against the CTLA-4 inhibitory molecule expressed on the surface of activated T cells [53]. CTLA-4 is a negative regulatory factor that competes for the same APC-expressed ligands as the T-cell co-stimulatory molecule CD28, however presenting significantly higher affinity [105]. Thus, it downregulates the antigen-specific immune responses initiated by interaction of APCs with T cells. Due to its capacity to inhibit this negative signaling pathway and contribute to restoration of the antitumor antigen-specific immune response, anti-CTLA4 is nowadays used as a novel therapy for solid tumors [106].
Programmed death-1 (PD-1) and lymphocyte-activation gene 3 (LAG-3) are yet two other negative regulatory factors of T-cell functions [107]. Recently, PD-1 blockade has been shown to increase the induction of effector T cells in the spleen, prolong T-cell proliferation, and enhance the recruitment of effector T cells to tumor sites [108]. In multimodality therapy regimens, PD-1 blockade increased therapeutic efficacy of total body irradiation and DCs transfer therapy [109]. Also, antibody blockade of LAG-3 in two murine models of self- and tumor-tolerance increased the accumulation and effector function of antigen-specific CD8+ T cells [110]. Thus, combination of mAb therapy against PD-1 or LAG-3 with immunization strategies has been recently demonstrated to restore the functions of tolerized antigen-specific CD8+ T cells [111].
Several approaches have been employed to induce high-avidity effector T cells in an attempt to target the inhibition of tumor-induced tolerance. One such approach involves blockade of TGF-β-induced signaling. In a xenograft mouse model of prostate cancer, transfer of tumor-reactive, TGF-β-insensitive CD8+ T cells leads to a 50 % decrease in average tumor weight, when compared with tumors of mice which underwent transfer of naïve CD8+ T cells [112]. Also, mAbs against TGF-β which are nowadays in clinical trials seem to be very promising antitumor candidates as they present little systemic toxicity [113]. Another approach aimed at manipulating TGF-β to improve antitumor immune responses involves generation of TGF-β-insensitive DC vaccines. Transduced DCs, which have been rendered insensitive to TGF-β, maintain their normal phenotype, present upregulated expression of surface co-stimulatory molecules (CD80/CD86), and induce potent tumor-specific cytotoxic T-lymphocyte responses in vivo [114].
Another class of antibodies capable of stimulating antigen presentation functions of APCs are agonistic antibodies against CD40 and/or CD40L [115]. As signaling via CD40 has been repeatedly shown to increase HLA expression and production of IL-2 by APCs, thus leading to T-cell activation [116], agonistic anti-CD40 antibodies presently tested in phase I clinical trials have a promising therapeutic potential.
5.4 Concluding Remarks
In the last few decades, major progress has been achieved within the field of cancer immunotherapy. However, despite this progress, the outcomes of clinical trials performed so far are significantly lower than expected. Contrary to the excellent therapeutic antitumor responses observed in animal tumors, clinical results in patients are modest, likely due to the variety and complexity of immune inhibitory mechanisms present within the tumor microenvironment. Some explanations that can account for this outcome are insufficient homing and activation of antigen-specific immune effector cells within the tumor or development of immunosuppressive mechanisms, capable to inhibit their cytolytic activity. This paradigm calls for strategies that could suppress the suppressors themselves, thus introducing the necessity of multimodality treatment regimens to achieve long-term tumor regression. Emerging clinical trials indicate towards the development of potent immunization strategies, leading to generation of high levels of effector T cells with a proper phenotype and specificity, as a possible answer to the problem. A desirable, highly effective immunization strategy should accomplish two purposes. On the one hand, it should aim at increasing both the recruitment of antigen-specific effector T cells to the tumor site and their intratumor arrest for the time necessary to exert their antitumor activity. For this purpose, combination of immunization regimens with ways to enhance homing of these cells to the tumor site, such as local tumor irradiation, endothelin B receptor blockade, antibody-mediated targeting of effector CTLs, or taxane-based chemotherapy, could be a promising strategy. On the other hand, targeting only the homing of vaccine-induced effector T cells to the tumor site might not be enough. We may speculate that once these cells have reached the tumor, they can be anergyzed or tolerized by diverse immunosuppressive mechanisms developed by the tumor itself or by secondary immunosuppressive populations. To counteract this effect, strategies that aim at maintaining or potentiating the activity of these intratumor antigen-specific effector T cells, such as depletion or functional inhibition of immunosuppressive populations and blockade of negative regulatory factors, are necessary.
In conclusion, development of new multimodality strategies in which immunization therapies are combined with effective antitumor immunological or conventional approaches aimed at increasing homing of immune effector cells to tumors and their intratumor activity is of crucial importance and represents the next step forward in cancer immunotherapy.
References
2.
Whiteside TL. Immune responses to malignancies. J Allergy Clin Immunol. 2010;125(2 Suppl 2):S272–83.PubMedCentralPubMed
3.
Sahin U, Tureci O, Pfreundschuh M. Serological identification of human tumor antigens. Curr Opin Immunol. 1997;9(5):709–16.PubMed
4.
Lee PP, Yee C, Savage PA, Fong L, Brockstedt D, Weber JS, et al. Characterization of circulating T cells specific for tumor-associated antigens in melanoma patients. Nat Med. 1999;5(6):677–85.PubMed
5.
Rabinovich GA, Gabrilovich D, Sotomayor EM. Immunosuppressive strategies that are mediated by tumor cells. Annu Rev Immunol. 2007;25:267–96.PubMedCentralPubMed
6.
Sadun RE, Sachsman SM, Chen X, Christenson KW, Morris WZ, Hu P, et al. Immune signatures of murine and human cancers reveal unique mechanisms of tumor escape and new targets for cancer immunotherapy. Clin Cancer Res. 2007;13(13):4016–25.PubMed
7.
Dunn GP, Koebel CM, Schreiber RD. Interferons, immunity and cancer immunoediting. Nat Rev Immunol. 2006;6(11):836–48.PubMed
8.
Schoenborn JR, Wilson CB. Regulation of interferon-gamma during innate and adaptive immune responses. Adv Immunol. 2007;96:41–101.PubMed
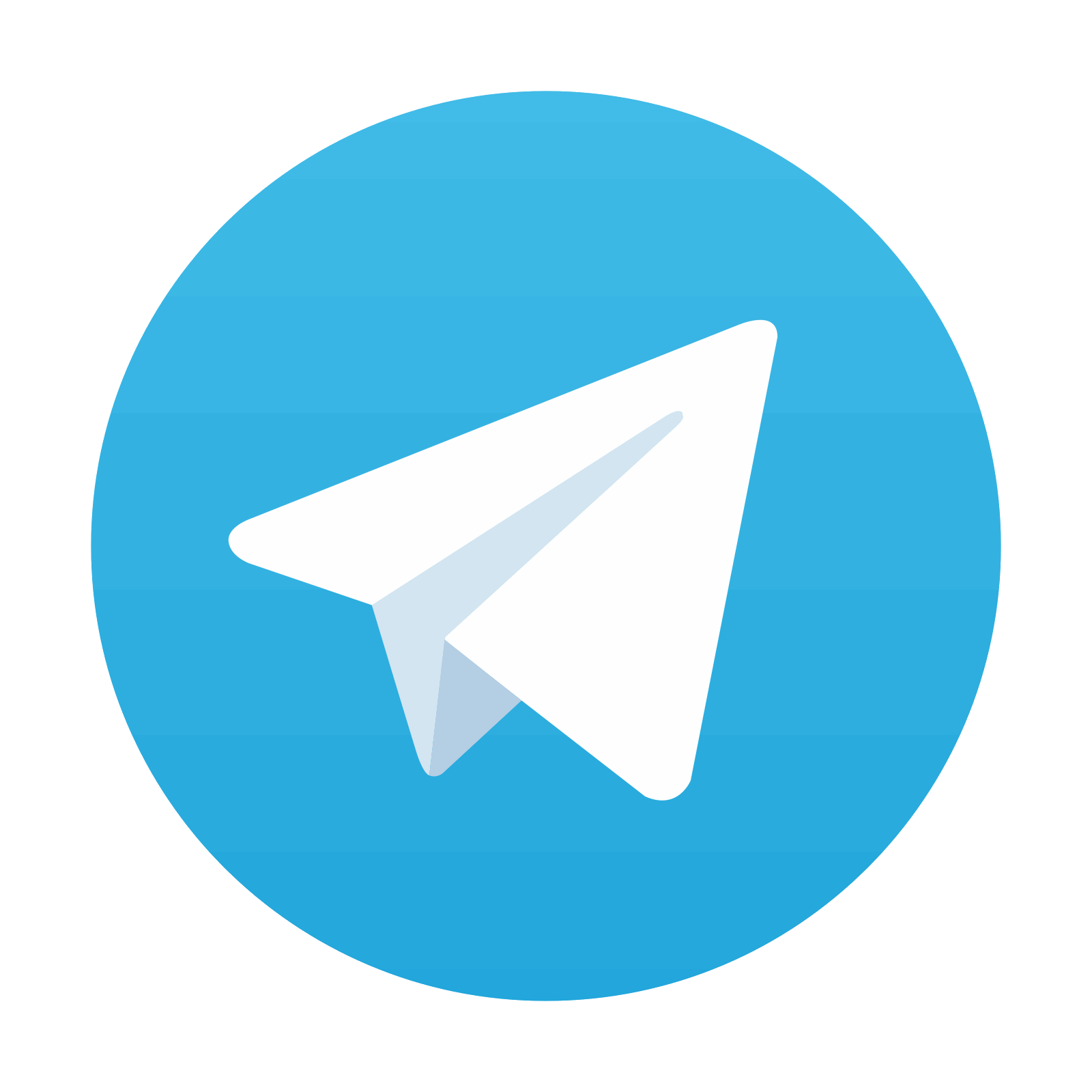
Stay updated, free articles. Join our Telegram channel

Full access? Get Clinical Tree
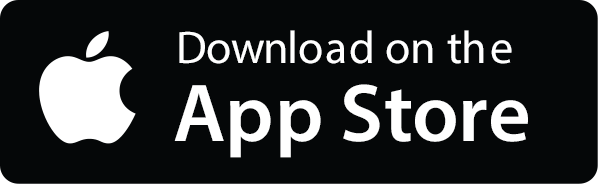
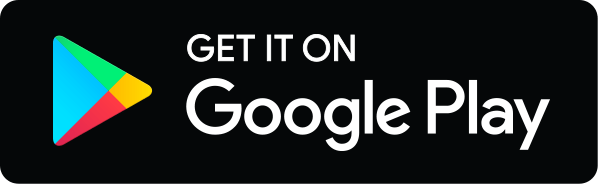