Stereotactic body radiation therapy (SBRT) has become the preferred treatment approach for patients with stage I non–small cell lung cancer who are medically inoperable or refuse surgery. SBRT consists of 3 to 5 radiation treatments delivered over a 10- to 14-day period. Local control is achieved in approximately 90% of patients, and the risk of toxicity is relatively low. Ongoing studies are examining whether SBRT can replace surgery for certain patients, the optimal dose-fractionation scheme, and how best to treat patients having central tumors near the primary tracheobronchial tree.
Key points
- •
Stereotactic body radiation therapy (SBRT) is an effective treatment for medically inoperable stage I non–small cell lung cancer with local control achieved in approximately 90% of patients.
- •
Most patients tolerate treatment exceptionally well. As high doses of radiation therapy are used, care is necessary when treating tumors that are located near the brachial plexus, primary tracheobronchial tree, skin, or chest wall. SBRT is currently being compared with surgery in randomized trials.
- •
SBRT has also been used for limited pulmonary metastases and studies are ongoing to determine the optimal method of integrating SBRT with systemic therapy.
Introduction
A conventional course of radiation therapy for most epithelial malignancies consists of daily treatments over a period of approximately 6 to 8 weeks. Stereotactic radiosurgery (SRS) has been successfully used for many years to manage both benign and malignant intracranial tumors. SRS contrasts dramatically with conventional radiation therapy. Instead of small daily fractions over many weeks, a single large treatment is precisely administered to a target while minimizing the dose to surrounding tissues. This approach has been adapted for extracranial targets whereby either a single or small number of dose-intense treatments are given over a 1- to 2-week period. This technique is commonly referred to as both stereotactic body radiation therapy (SBRT) and stereotactic ablative radiotherapy.
Because SBRT consists of large radiation doses, which are associated with a greater potential for local tumor control but also normal tissue complications, with the radiation dose highly conformal to the target (making precise delivery critical), 3 components are mandatory for the successful and safe implementation of SBRT. The first component is reproducible and comfortable patient immobilization to prevent movement during the radiation treatment. This immobilization is most commonly performed using a Styrofoam (The Dow Chemical Company, Midland, Michigan) mold, vacuum bag, or a body frame. The second component is the assessment of respiratory-induced motion. Liver and lung tumors, in particular, are not stationary during the respiratory cycle and their motion must be taken into account during both the planning and treatment phase. Numerous strategies have been implemented to minimize and/or account for this. The third component includes the ability to image the tumor immediately before the treatment to ensure that the target and radiation dose distribution are aligned properly. This process is termed image-guided radiation therapy and is typically performed with computed tomography (CT) imaging or with implanted fiducial markers.
SBRT has emerged as an attractive, and potentially more effective, alternative to conventional radiation therapy for a variety of malignancies. Numerous prospective studies have evaluated SBRT for medically inoperable stage I non–small cell lung cancer (NSCLC). For most other malignancies, the data remains preliminary, although numerous prospective studies are currently ongoing. SBRT is being formally investigated for prostate cancer, pancreatic cancer, breast cancer, painful bone metastases, and liver and lung oligometastases, to name just a few. This article aims to summarize the data on SBRT, with a particular focus on stage I lung cancer and pulmonary metastases.
Introduction
A conventional course of radiation therapy for most epithelial malignancies consists of daily treatments over a period of approximately 6 to 8 weeks. Stereotactic radiosurgery (SRS) has been successfully used for many years to manage both benign and malignant intracranial tumors. SRS contrasts dramatically with conventional radiation therapy. Instead of small daily fractions over many weeks, a single large treatment is precisely administered to a target while minimizing the dose to surrounding tissues. This approach has been adapted for extracranial targets whereby either a single or small number of dose-intense treatments are given over a 1- to 2-week period. This technique is commonly referred to as both stereotactic body radiation therapy (SBRT) and stereotactic ablative radiotherapy.
Because SBRT consists of large radiation doses, which are associated with a greater potential for local tumor control but also normal tissue complications, with the radiation dose highly conformal to the target (making precise delivery critical), 3 components are mandatory for the successful and safe implementation of SBRT. The first component is reproducible and comfortable patient immobilization to prevent movement during the radiation treatment. This immobilization is most commonly performed using a Styrofoam (The Dow Chemical Company, Midland, Michigan) mold, vacuum bag, or a body frame. The second component is the assessment of respiratory-induced motion. Liver and lung tumors, in particular, are not stationary during the respiratory cycle and their motion must be taken into account during both the planning and treatment phase. Numerous strategies have been implemented to minimize and/or account for this. The third component includes the ability to image the tumor immediately before the treatment to ensure that the target and radiation dose distribution are aligned properly. This process is termed image-guided radiation therapy and is typically performed with computed tomography (CT) imaging or with implanted fiducial markers.
SBRT has emerged as an attractive, and potentially more effective, alternative to conventional radiation therapy for a variety of malignancies. Numerous prospective studies have evaluated SBRT for medically inoperable stage I non–small cell lung cancer (NSCLC). For most other malignancies, the data remains preliminary, although numerous prospective studies are currently ongoing. SBRT is being formally investigated for prostate cancer, pancreatic cancer, breast cancer, painful bone metastases, and liver and lung oligometastases, to name just a few. This article aims to summarize the data on SBRT, with a particular focus on stage I lung cancer and pulmonary metastases.
SBRT: stage I NSCLC
Surgery remains the preferred treatment modality for stage I NSCLC. However, patients with lung cancer often present with serious medical comorbidities, often related to tobacco abuse, which make them high-risk operative candidates. Many such patients are observed and do not receive any cancer-directed treatment. Despite their other comorbidities, most patients who are observed eventually die of lung cancer. Many other patients have minimal pulmonary reserve and undergo sublobar resections (eg, wedge resection), which are associated with a higher risk of local disease recurrence. Other patients, particularly the elderly, wish to avoid surgery and seek out less-invasive alternatives.
Historically, conventional radiation therapy was the treatment of choice for patients with inoperable stage I NSCLC. The typical treatment was 60 to 66 Gy in 1.8- to 2.0-Gy daily fractions. Although this was generally well tolerated, 5-year survival was poor (∼15%), with approximately 25% to 50% of tumors recurring at the treated site. The primary reason for such a high risk of treatment failure may have been an inadequate radiation dose. Therefore, beginning in the early 1990s, investigators from around the world began exploring SBRT as an alternative approach. Biologically, a few very large treatments are more potent than many small treatments, even when the total radiation dose is the same. Furthermore, multiple radiation fields from different directions are used in SBRT. This method helps create a dose distribution that is conformal to the target, limiting the high dose to surrounding tissues and allowing for the safer administration of larger radiation doses ( Figs. 1 and 2 ).
The first phase I dose-escalation study was performed by Robert Timmerman and colleagues at Indiana University. Eligible patients were required to have stage I NSCLC measuring 7 cm or less, with medical comorbidities precluding an operation. Patients were grouped into 3 cohorts (T1, T2 <5 cm, T2 >5–7 cm). SBRT consisted of 3 treatments over approximately 2 weeks, beginning with a total radiation dose of 24 Gy (8 Gy × 3), with the dose progressively escalated to 72 Gy (24 Gy × 3). The maximum tolerated dose was not reached for T1 and T2 tumors less than 5 cm and was 66 Gy in 3 fractions for T2 tumors larger than 5 cm.
A subsequent phase II study from Timmerman and colleagues treated 70 patients with medically inoperable stage I NSCLC using the fractionation scheme established in the phase I study (20–22 Gy × 3). After a median follow-up of 50 months, 3-year actuarial local control, cancer-specific survival, and overall survival was 88%, 82%, and 43%, respectively. Local control with SBRT far exceeded results with conventional radiation techniques. However, as mentioned previously, larger radiation doses can potentially lead to toxicities not typically observed with more conventional fractionation schedules. It was observed that patients with central tumors and larger tumors were at higher risk of high-grade toxicity compared with patients with smaller and more peripheral lesions.
A multicenter phase II study performed by the Radiation Therapy Oncology Group (RTOG; 0236) was the first North American multicenter, cooperative group study to evaluate SBRT in medially inoperable patients. Given the complexity of SBRT, each center was required to pass central credentialing standards for protocol participation. Because of the findings from the Indiana University phase II study, only patients with smaller (<5 cm) and peripheral (greater than 2 cm from the proximal bronchial tree) tumors were eligible. Patients deemed medically operable were ineligible. All patients (n = 55) were treated with 3 fractions of 20 Gy without tissue density heterogeneity corrections (equivalent to approximately 18 Gy × 3 with corrections). After a median follow-up of 34 months, the 3-year actuarial local tumor control was 98%. The most common site of failure was distant metastases, occurring in 22% of patients. The overall survival at 3 years was 56%. Grade 3 to 4 toxicity occurred in 15% of patients, which was primarily pulmonary toxicity, including decreased pulmonary function tests, pneumonitis, and hypoxia.
These excellent results have been corroborated by multiple prospective studies conducted in different countries ( Table 1 ). Although a variety of dose fractionation schemes have been used, actuarial local control in most studies approaches or exceeds 90%, particularly when the biologic effective dose is more than 100 Gy 10 . In a population of patients who are medically inoperable, 3-year actuarial survival rates of 50% to 60% are also promising and seem to be greater than series in which conventional radiation therapy techniques were used. Patterns of failure seem to have shifted with SBRT, with distant metastases being the most common site of failure compared with conventional radiation therapy where local failure is more prominent ( Fig. 3 ).
Study | n | Dose (Fractionation) | Survival (%) (Year) | Local Failure (%) (Year) |
---|---|---|---|---|
Nagata et al, Japan | 45 | 48 Gy (12 Gy × 4) | 83: T1 (5) 72: T2 (5) | 5: T1 (5) 0: T2 (5) |
Baumann et al, Sweden | 57 | 45 Gy (15 Gy × 3) | 60 (3) | 8 (3) |
Fakiris et al, Indiana | 70 | 60–66 Gy (20–22 Gy × 3) | 43 (3) | 12 (3) |
Ricardi et al, Italy | 62 | 45 Gy (15 Gy × 3) | 57 (3) | 12 (3) |
Bral et al, Belgium | 40 | 60 Gy (20 Gy × 3) a 60 Gy (15 Gy × 4) b | 52 (2) | 16 (2) |
Hoyer et al, Denmark | 40 | 45 Gy (15 Gy × 3) | 47 (2) | 15 (2) |
Timmerman et al, RTOG | 55 | 54 Gy (18 Gy × 3) c | 56 (3) | 2 (3) |
SBRT: complications and risks
Overall, most patients tolerate SBRT exceptionally well. It is an outpatient procedure that takes approximately 45 minutes to administer. The actual treatment only takes a few minutes, with most of the time dedicated to patient setup and tumor position verification. SBRT consists of large doses, which can be associated with complications even when carefully administered. Fortunately, high-grade toxicity occurs in only a minority of patients (∼15%).
Pulmonary Toxicity
Most patients develop increased tissue density on CT in the high-dose region after SBRT. This increased density is typically asymptomatic but it can be difficult to differentiate from persistent or progressive tumor. Most studies have shown that pulmonary function tests are relatively preserved after SBRT, permitting the use of SBRT even in patients with severely compromised pulmonary function.
Other pulmonary complications have been reported after SBRT, including radiation pneumonitis and pneumonia. High-grade toxicity has been reported to be higher in patients with central tumors and larger tumors after SBRT, particularly with high-dose 3-fraction regimens (20 Gy × 3).
Chest Wall/Skin Toxicity
Many patients with inoperable stage I lung cancer have peripheral tumors in close proximity to the chest wall. In patients with breast cancer receiving conventionally fractionated radiation therapy, there is a small increased risk of rib fractures. Rib fractures and chronic chest wall pain have also been observed after SBRT. Defining the chest wall as the bone and soft tissues 3 cm from the lung/chest wall interface, one study noted that grade 3 chest wall pain or rib fractures were observed when the volume of chest wall receiving 30 Gy or higher (in 3–5 fractions) exceeded 30 cm 3 . The risk of chest wall toxicity increased to 30% when the volume receiving 30 Gy exceeded 35 cm 3 . Similar findings were noted from MD Anderson. In another study specifically evaluating rib fractures, the risk was related to the total dose delivered to 2 cm 3 of the rib. These studies suggest that there is a dose response but further work is necessary to refine our understanding of chest wall toxicity after SBRT.
Skin reactions are very common after conventionally fractionated radiation therapy and are almost universally transient without long-term complications. However, with large radiation fractions used during SBRT, the risk of severe toxicity increases. In a large series of patients with peripheral lung tumors from MD Anderson, the risk of grade 0, 1, 2, and 3 toxicity after lung SBRT (12.5 Gy × 4–50 Gy) was 61%, 29%, 7%, and 3%, respectively. A case of grade 4 skin necrosis has also been reported. Radiation oncologists must be vigilant in evaluating the dose to the skin, especially in thin patients with posterior tumors near the chest wall.
Brachial Plexopathy
In patients with apical lesions, the brachial plexus should be contoured on the treatment planning software and the amount of radiation administered to that structure assessed. It has been shown that the risk of brachial plexopathy is unacceptably high when the maximum dose exceeds 26 Gy.
SBRT: future directions in lung cancer
Surgery Versus SBRT
Although there are theoretical reasons why lobectomy may be advantageous over SBRT for operable patients (surgical staging, chemotherapy use for incidental stage II disease, removal of interlobar lymphatics that may harbor cancer, and so forth), there are compelling reasons to think that SBRT may be equivalent to, or perhaps even preferred over, wedge resection. A recent single-institution analysis compared SBRT with wedge resection in patients with stage I lung cancer who were not eligible for lobectomy. Patients undergoing SBRT were older, with more comorbidities, and generally medically inoperable. There was a trend for a lower risk of local failure with SBRT (4% vs 20%, P = .07), although ultimate cause-specific survival was equivalent. The overall survival was higher in patients undergoing surgery, because of more non–lung cancer deaths after SBRT, indicating patient selection. It is difficult to compare these patient populations (clinical vs pathologic staging, differences in assessment of local control, prospective vs retrospective collection of data, and so forth), but this study suggests that SBRT may be a reasonable alternative for patients who would otherwise undergo a wedge resection for stage I NSCLC.
Two phase II trials have completed accrual formally evaluating the role of SBRT in an operable population. Preliminary outcomes from the Japanese Clinical Oncology Group 0403 study have been presented demonstrating 3-year progression-free and overall survival rates of 55% and 76%, respectively. RTOG 0618 has also completed enrollment and results are pending.
Randomized trials have also been designed to compare surgery with SBRT. Because of various factors, including long established standards of care, lack of equipoise among specialists, and, in particular, patient randomization to a surgical versus nonsurgical treatment, these trials are expected to be difficult to complete. The Randomized Clinical Trial of Either Surgery or Stereotactic Radiotherapy for Early Stage (IA) Lung Cancer trial was initiated in the Netherlands but closed prematurely because of slow accrual. A CyberKnife (Accuray, Sunnyvale, CA) trial sponsored by Accuray is comparing SBRT with lobectomy and is currently enrolling participants. The American College of Surgeons Oncology Group and RTOG have opened a study for high-risk operable patients comparing sublobar resection and SBRT.
Optimal Dose-Fractionation Scheme
A variety of dose-fractionation schemes have been evaluated in prospective trials (see Table 1 ). In clinical practice, the size of the tumor, the location in the chest, and other factors have an influence on the treatment prescription. A randomized phase II study by the RTOG (0915) has completed accrual comparing 34 Gy in one fraction with 48 Gy in 4 fractions. The less toxic regimen will be compared with the 18 Gy × 3 regimen, which was standardized by the prospective studies at Indiana University and RTOG 0236.
Excess toxicity has been encountered when intense treatment regimens (18 Gy × 3) are used for central tumors. RTOG 0813 is a dose-escalation study attempting to define the maximally tolerated dose for tumors that arise within 2 cm of the tracheobronchial tree.
Systemic Therapy
Finally, patterns of failure show that distant metastases are the primary cause of treatment failure after SBRT (see Fig. 3 ). Incorporation of systemic therapies to decrease this risk seems rational but may be difficult in patients who are medically inoperable. Multiple studies have been proposed but none are completed to date.
SBRT: pulmonary metastases
Rationale for Treatment of Limited Metastatic Disease
Because SBRT has been shown to be an effective local therapy for early stage NSCLC, it has also been investigated as a treatment of pulmonary, hepatic, adrenal, retroperitoneal, spinal, and multiple organ metastases. Initial studies investigating pulmonary SBRT included patients with both primary tumors and metastases because it was assumed that treatment-related toxicity would be similar for both. Since these early retrospective reports, prospective phase I/II studies have shown that SBRT can be used safely and effectively as a noninvasive procedure for many metastatic sites with outcomes similar to surgical metastasectomy. Investigations continue to further refine patient selection criteria, optimize radiation-dosing schedules, and understand how to integrate this technology with systemic therapy.
Hellman and Weichselbaum proposed a distinct clinical state of oligometastases to provide a framework to guide further research and understanding of potentially curable patients with limited metastatic disease. They postulated that cancer was a spectrum of disease including those that were localized to their original site that would not spread and, on the other extreme, those that had spread with many metastases in many destination organs. Contained within this spectrum was a state with metastatic tumors limited in number and destination organ. For some of these patients, it was proposed that progression may not be widespread in the course of their disease. These patients were designated as having de novo oligometastases . Alternatively, a more widely metastatic state could be converted to a limited metastatic burden or induced oligometastatic state following the administration of effective systemic therapy. As imaging and systemic therapy improve, the detection of patients with limited metastatic disease (patients with potential de novo oligometastases) as well as the potential for induced oligometastases should be increasing. Promising outcomes of patients following the surgical removal of pulmonary, hepatic, intracranial, and multiple organ metastases, with a 5-year survival approaching 25%, supports the prediction from the oligometastatic hypothesis that metastasis-directed therapy can result in long-term disease control.
Radiation therapy can also be used as an effective means to aggressively treat metastatic disease. Although radiation is typically reserved for palliation of hemoptysis, dyspnea, dysphagia, cough, neurologic symptoms, and pain in patients with metastatic cancer, it can also be delivered with the intent of improving overall survival. RTOG 9508 randomized patients with 1 to 3 brain metastases to either whole brain radiotherapy or whole brain radiotherapy and stereotactic radiosurgery. Survival was significantly improved with the addition of SRS in patients with one metastasis, those with squamous or non–small cell histology, and in those in recursive partitioning analysis (RPA) class 1 (good performance status, controlled primary tumors, aged <65 years, and no extracranial disease), similar to results seen following surgical resection of brain metastases. These data suggest that for patients who are not technically resectable or medically fit for surgery, aggressive radiation (SBRT) offers a viable alternative for effective metastasis-directed therapy.
For pulmonary metastases, SBRT has been shown to be an effective metastasis-directed therapy for patients with low-volume disease. The peripheral location and well-circumscribed morphology of many pulmonary metastases allow for delivery of SBRT with a low probability for treatment-related toxicity. Large series reporting on the treatment of pulmonary metastases with SBRT are limited because of the recent development and widespread application of the technique. Selected series are shown in Tables 2 and 3 . Control of treated pulmonary metastases with SBRT is promising, approaching 70% to 90% at 2 years, comparable with that of pulmonary metastasectomy.
Author, Institution | Year | N | Median f/u (Months) | Primary, Mets, Both | Total Dose (Gy) | Number of Doses | Local Control | OS |
---|---|---|---|---|---|---|---|---|
Uematsu et al, National Defense Medical College, Japan | 1998 | 45 P: 23 M: 22 | 11 | B | 30–75 | 5–15 | B: 97% | — |
Nagata et al, Kyoto University, Japan | 2002 | 40 P: 31 M: 9 | 16 | B | 40–48 | 4 | T1a: 100% T1b: 93% Mets: 67% | 1 y: 87% |
Yoon et al, Asan Medical Center, South Korea | 2006 | 91 P: 38 M: 53 | 14 | B | 30–48 | 3–4 | 2 y: P: 81% M: 80% | 2 y: P: 51% M: NS |
Blomgren et al, Karolinska Hospital, Sweden | 1998 | 31 M: 5 | 8.2 | B | Mean 40 | 1–3 | 92% crude | 11 mo (mean) |
Wulf et al, University of Wuerzburg, Germany | 2005 | 81 P: 36 M: 45 | 14 | B | 30.0–37.5 | 3 | 1 y P: 92% M: 72% | 1 y P: 52% M: 85% |
Le et al, Stanford University, United States | 2006 | 32 P: 20 M: 12 | 18 | B | 15–30 Gy | 1 | 1 y P: 78% M: 58% | 1 y P: 85% M: 56% |
Hamamoto et al, Shikoku Cancer Center, Japan | 2010 | 62 P: 52 M: 10 | 14 | B | 48 Gy | 4 | 2 y: P: 88% M: 25% | 2 y: P: 96% M: 86% |
Takeda et al, Ofuna Chuo Hospital, Japan | 2011 | 217 P: 183 M: 35 | 15–29 | B | 50 Gy | 5 | 2 y: P: 93% M: 82% | NS |
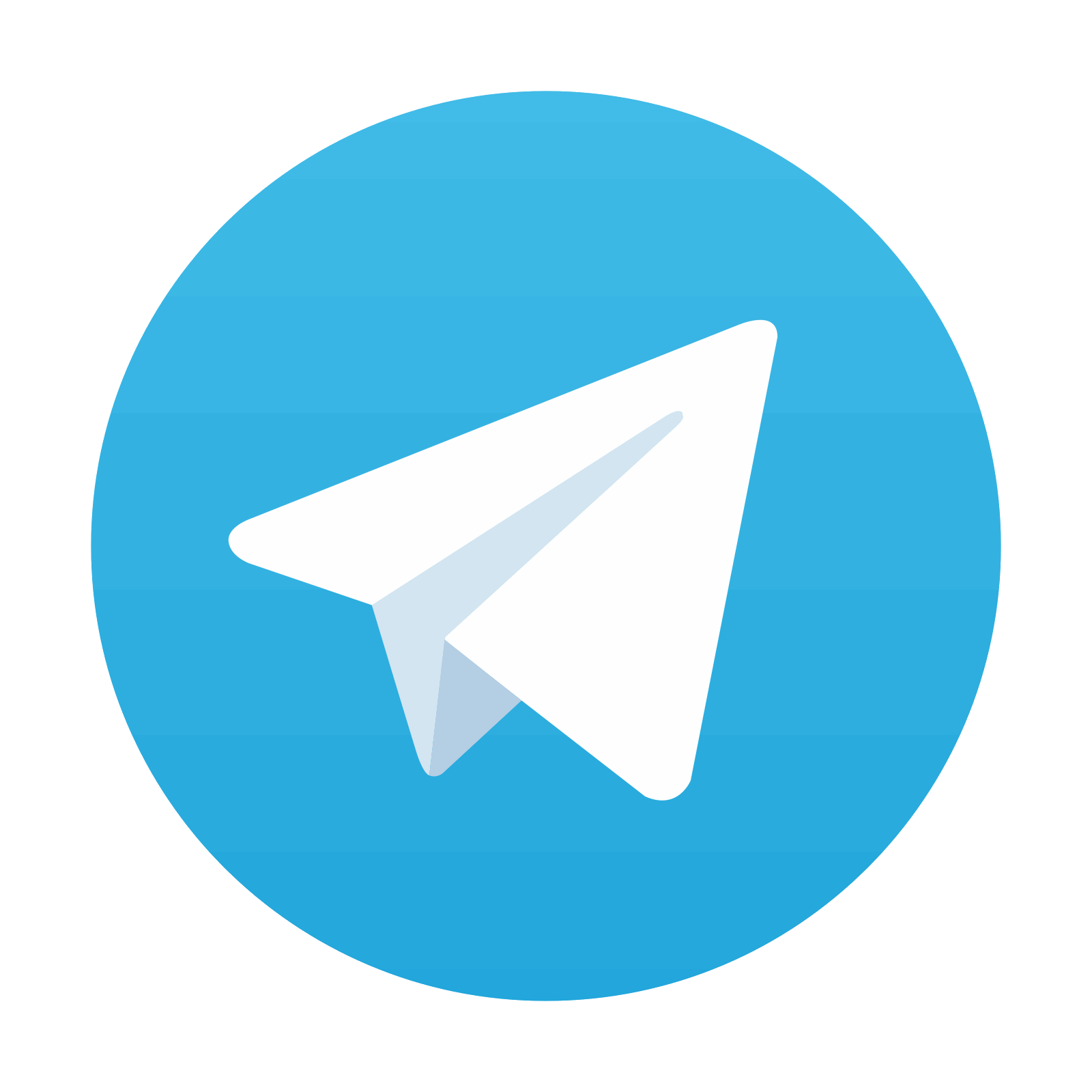
Stay updated, free articles. Join our Telegram channel

Full access? Get Clinical Tree
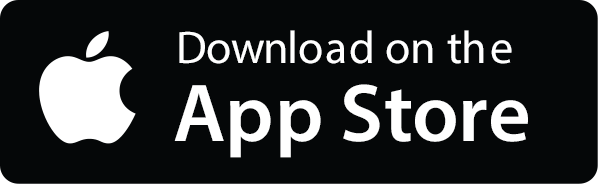
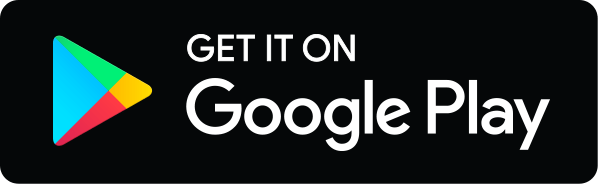
