75 Stereotactic Body Radiation Therapy
History of Stereotactic Body Radiation Therapy
Derived from the Greek term stereo, meaning solid or three-dimensional, and the Latin term tact, meaning to touch, stereotactic radiosurgery (SRS) has evolved as a widely used radiation technique that delivers high doses of radiation with exquisite accuracy to target lesions. By cross-firing radiation beams, rapid dose fall-off at the junction of the target and adjacent tissue is achieved, which reduces radiation dose to adjacent normal tissues. Introduced by Swedish neurosurgeon Lars Leksell in 1951 using a rigidly affixed skull frame to achieve accuracy, SRS has been widely successful in the management of a variety of intracranial conditions. The broad application of this concept to areas outside of the brain and head and neck regions in the past decades was limited by the complexities of internal organ motion, as well as less sophisticated technology. However, in 1991, 40 years after Leksell coined the term stereotactic radiosurgery, Ingmar Lax and colleagues at the Karolinska Hospital in Stockholm were credited as the first to propose the idea of extending stereotactic treatment approaches outside of the head to targets in the thoracic and abdominal cavities, reporting the initial results of this treatment in 1995.1 Soon thereafter, Hamilton and colleagues at the University of Arizona published the first studies on spinal radiosurgery using a skeletal fixation device applied during an operative procedure followed by treatment on a linear accelerator under anesthesia.2 Evolving from similar concepts as SRS, stereotactic body radiotherapy (SBRT) has emerged during the past decade and a half, coincident with the availability of high-speed computing and advanced imaging to allow for improved methods of dealing with respiratory motion and less invasive treatment delivery. Currently, with several mature prospective clinical trials of SBRT completed and many more ongoing, the first decade of the 21st century has witnessed an extraordinary expansion of treatment advancements for spinal tumors, lung cancer, pancreatic cancer, and liver malignancies. Emerging data in prostate cancer and renal cancer are also promising.
Radiobiology
The linear quadratic model (LQM) was developed as a mechanistic model to describe the radiobiologic effects of cell killing and sublethal repair.3 The LQM describes the probabilities of double-strand DNA breaks (DSBs), which are considered the lethal lesion induced by radiation. This probability is governed by a linear component that represents single-track damage causing a DSB, whereas the quadratic component is derived from two separate actions on the DNA leading to DSBs. The LQM predicts the isoeffect relationships for alternative fractionation schedules for early and late normal radiation reactions. Although the early models accounted mainly for repair, modifications to the original model have been made over time to account for the effects of the Rs of radiobiology: Repopulation, Redistribution, Reoxygenation and Repair.4,5
Although the LQM has proven to be almost indispensible for clinical judgments using relatively low fractional doses, the terminal bend of the survival curve at high doses creates some ambiguity about its utility for SBRT. Using the LQM, validations against clinical data appear to overestimate cell kill and underestimate toxicity at high fractional dose ranges. Some argue that mechanistic models may not fully account for all processes that are at work and favor empirically derived models. Based on the notion that the elements of the therapeutic ratio, namely tumor control as well as normal tissue complications are ultimately related predominantly to cell kill, Brenner demonstrated that the LQM has been validated for fractional doses of up to 10 Gy per fraction. Moreover, he argues that it is probably reasonable to use the LQM for fractional doses of up to 18 Gy.6 Citing a study by Ch’ang et al. who irradiated mice with a range of single fractions and analyzed gastrointestinal stem cells, Kirkpatrick et al. argues that the validity of the LQM is in question at very high doses.7 In this study, although animals irradiated to doses of 8 to 13 Gy did not die of the gastrointestinal syndrome, there was death of stem cells by apoptosis resulting in gastrointestinal syndrome and death of the animal following single doses greater than 17 to 18 Gy.8 The suggestion is that (at least in mice), with doses greater than 17 to 18 Gy, a radiation threshold of either stem cell or vasculature cell killing had been crossed. Because the LQM does not account for these observed effects, caution should be used when applying the LQM to situations of high dose per fraction. Although this and other arguments fuel the fierce debate over its validity for SBRT hypofractionated schemes, in the absence of other suitable models, the LQM remains widely used to make quantitative estimates of radiobiologic effectiveness for SBRT.
Current Stereotactic Body Radiotherapy Technologies
By today’s standards, the first SBRT treatments may seem simplistic and less sophisticated. However, the concepts of stereotactic localization and internal motion managment have served as a foundation for expanding the application of SBRT. In 1994, Lax et al.9 first described a nonrigid stereotactic body frame that contained markers for localization and a vacuum system to maximize the body contact with the frame. To reduce internal motion caused by breathing, slight constant pressure was applied to the abdomen, reducing the measured excursion of the diaphragm from 1.5 to 2.5 cm to 0.5 to 1.0 cm.9 An array of approximately eight noncoplanar, isocentric beams, which were shaped to the beam’s-eye view, were used to achieve a conformal dose distribution around the target. Similar to the initial SBRT treatments, the most widely used systems for SBRT use linear accelerators. Unlike the early techniques, several of the newer systems for SBRT in use today are integrated image-guidance systems, which incorporate either digital x-ray or tomographic imaging to assist in localization. The most widely used systems for SBRT fall into one of the following categories:
Clinical Outcomes By Organ Site
Lung Tumors
Early Stage Non–Small Cell Lung Carcinoma
Approximately 15% to 20% of patients with non–small cell lung carcinoma (NSCLC) present with stage I or II surgically resectable disease.10 Surgical resection is still the preferred curative treatment for medically operable patients, yielding 5-year survival rates of 60% to 70%.11,12 However, the surgical approach may not be an option for patients who have poor lung function, cardiac disease, other comorbid conditions, or who refuse surgery. In the past, these patients have been treated with conventional radiation therapy achieving poorer 5-year local control rates (30%-50%) and overall survival rates (10%-30%). In an analysis of Survival, Epidemiology, and End Results data comparing the outcomes of more than 4300 non–surgically resected, early stage lung cancers treated with or without radiotherapy, Wisnivesky et al.13 showed that radiotherapy was associated with improvement in median survival from 14 months to 21 months. However, these general outcomes are still poor compared with surgery. Recent studies have suggested that better local control of the primary lesion correlated with the dose of radiation delivered. In a phase I dose escalation trial of 3-D conformal radiotherapy, escalating doses from 70.2 Gy to 84 Gy delivered in 1.8-2 Gy fractions, doses of greater than 80 Gy were associated with significantly higher local control and overall survival rates, implying a need for radiation dose intensification for improved outcomes.14 In this study, dose could not be escalated beyond 84 Gy because of unacceptable toxicity associated with irradiating excessive volumes of normal tissue. Using more sophisticated respiratory motion management and improved treatment planning techniques, SBRT has emerged as the platform to achieve dose intensification in treating early stage lung cancer.15,16
Several groups worldwide have now reported encouraging prospective and retrospective results of SBRT for NSCLC.17–21 More recently, these data have been analyzed to determine factors that may predict for improved outcome and avoidance of excessive complications. Factors that have been determined to be of significance are: (1) tumor size, (2) effective radiation dose delivered, and (3) location of the tumor. In a retrospective analysis of 257 patients with early stage NSCLC who were treated with doses ranging from 18 to 75 Gy (1-22 fractions), Onishi et al.22 demonstrated a dose response for survival and local control. In this study, the dose regimens were converted to a biologically effective dose (BED) according to the LQM. Local control was significantly improved when BED was greater than 100 Gy; in other words, local recurrence rates were 8.4% for BED larger than 100 Gy versus 42.9% for BED less than 100 Gy (P < 0.001). Moreover, the 5-year overall survival rates were also significantly improved for BED larger than 100 Gy versus less than 100 Gy, of 70.8% versus 30.2%.22
Building upon a previously reported phase I dose escalation trial of SBRT,23 Timmerman et al. reported the results of a phase II study of patients with stage I inoperable NSCLC who received 60 to 66 Gy in three fractions. With a median follow-up at 17.5 months, the 2-year local control rate was 95% and the 2-year overall survival rate was 54.7%.24 In a recent update of this series with a median follow-up of 50.2 months (range 1.4-64.8 months), 3-year local control was 88.1% and 3-year overall survival was 42.7%.25 Tumor volume (<5 cc, 5-10 cc, 10-20 cc, >20 cc) did not significantly affect survival. Although interim reporting of these data showed that the majority of the 14 of 70 patients who experienced grade 3 through 5 toxicity had centrally located tumors adjacent to the proximal bronchial tree and central chest,24 in this more recent analysis, grade 3 to 5 was not statistically different between the cohorts with peripheral lung tumors (10.4%) and central tumors (27.3%) (Fisher’s exact test, P = 0.088). Furthermore, there was no significant survival difference between patients with peripheral versus central tumors (median survival 33.2 versus 24.4 months, respectively, P = 0.697). Even in light of these emerging data, SBRT practitioners remain cautious when considering treatment of centrally located tumors.
Lung Metastases
The rationale of treating lung metastases comes from the surgical observation that showed increase survival after metastatectomy.26–28 The International Registry of Lung Metastases, established in 1991 to assess the long-term results of pulmonary metastasectomy reported 36% actuarial survival at 5 years and 26% at 10 years following complete metastasectomy among its cohort of 5206 subjects.26
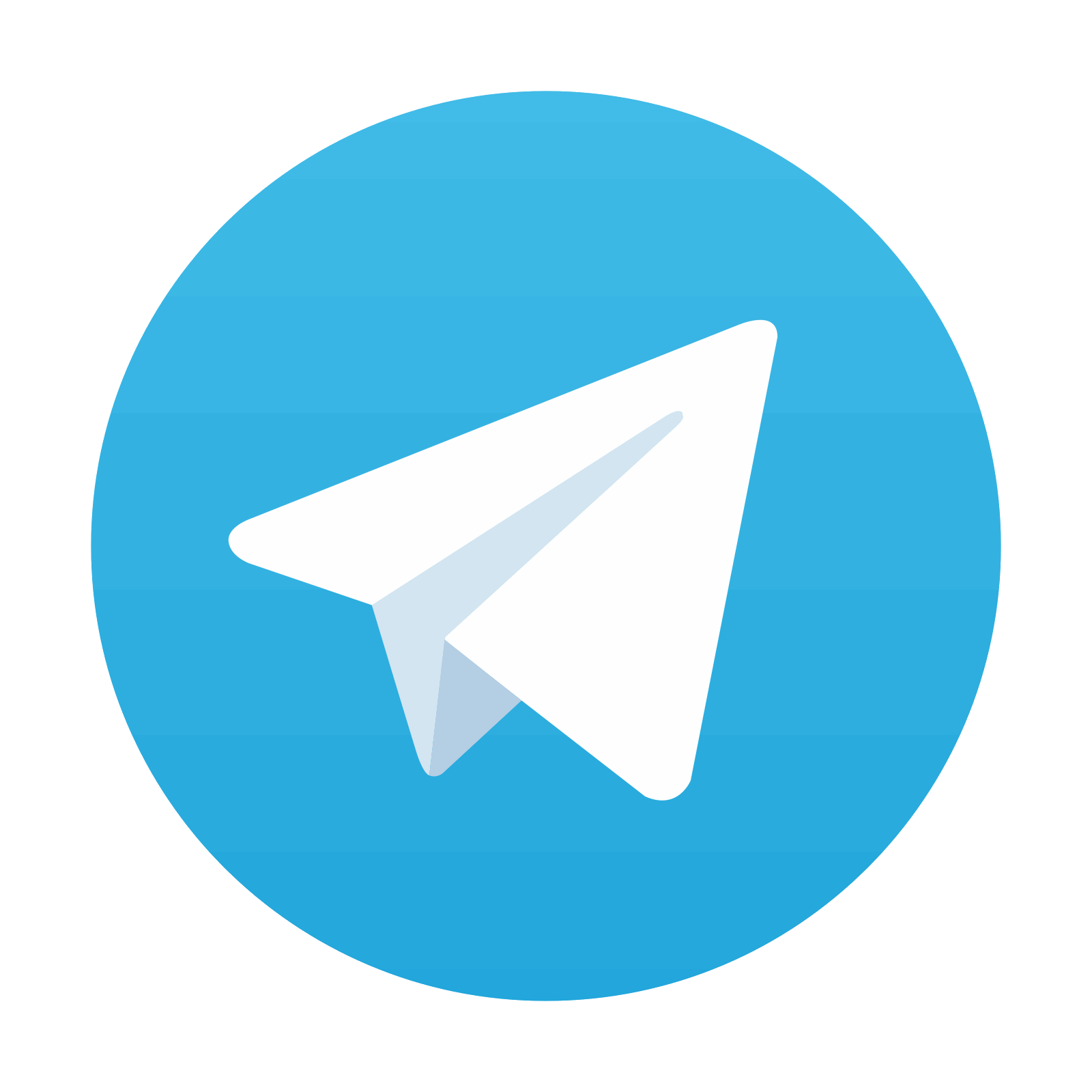
Stay updated, free articles. Join our Telegram channel

Full access? Get Clinical Tree
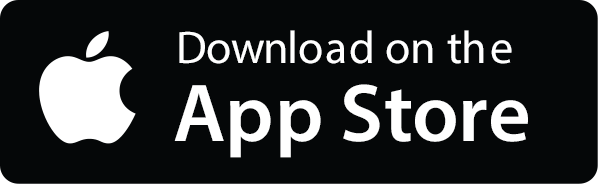
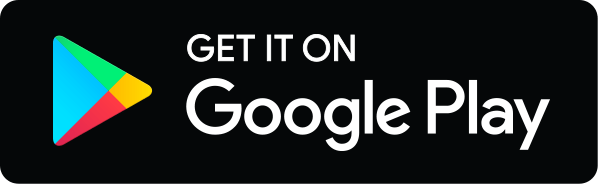