17 Margot Umpleby and Barbara A Fielding University of Surrey Stable isotopes have been used to study metabolism since the 1930s, when Shoenheimer and Rittenberg fed linseed oil labelled with deuterium to mice and showed that 33% of the deuterium-labelled fatty acids appeared in adipose tissue. The lack of availability of stable isotopes and the cost of the mass spectrometers needed for their measurement did not lead to widespread use. Because there was a plentiful supply of radioisotopes that could be easily measured by scintillation counters, this led to their use instead for the study of metabolism and nutrition in humans. The concern about the health hazards of radioisotopes, the development of more affordable mass spectrometers and the lack of availability of some suitable radioisotopes – for instance, for nitrogen the only available radioisotope has a half-life of 10 minutes – led to the greater use of stable isotopes in the late 1960s and early 1970s. Today in the UK, metabolic tracer studies almost exclusively use stable isotopes, although many of the techniques that are used were developed using radioactive tracers. Certain elements are composed of atoms that are chemically identical but of a slightly different weight due to different numbers of neutrons. These are termed isotopes. The most abundant isotopes of the major elements in the biological environment, hydrogen (H), carbon (C), nitrogen (N) and oxygen (O), are 1H, 12C, 14 N and 16O and are by definition stable, but there are other stable isotopes of these elements that are much less abundant (0.02–1.1%); that is, 2H, 13C, 15 N and 18O. For the purposes of this chapter, the term ‘stable isotope’ will refer to the less abundant stable isotopes. The substitution of one of these less abundant isotopes for the more common form in a molecule, known as ‘labelling’, creates a ‘tracer’ that has the same chemical properties as the original molecule, known as the tracee. Isotopic enrichment is expressed as the tracer:tracee ratio (TTR), corrected for the baseline TTR or as atom % excess (APE) or mole % excess (MPE). The standard nomenclature for a stable isotope tracer gives the isotope and the number of the atoms substituted with the isotope; thus, [2H7]glucose indicates the presence of seven atoms of 2H, and [U-13C]glucose indicates that every carbon has been replaced by a stable isotope (this is known as ‘uniformly labelled’). The stable isotope of 2H is called deuterium and a common nomenclature for this is D, for example [2H2] glucose is shown as D2 glucose. Unlike radioactive isotopes, stable isotopes are not a source of ionising radiation. In the quantities administered they are safe and non-toxic and studies can be undertaken in humans, including pregnant women and children. Administration of a tracer either orally or intravenously enables the measurement of carbohydrate, lipid and protein metabolic pathways either qualitatively or quantitatively, depending on the methodology used. Tracers of vitamins can be used to study their absorption, metabolism and excretion. There are also less abundant stable isotopes of most of the major dietary minerals and the trace elements, and these can be used to study their absorption and kinetics. The application of stable isotope tracers should induce only minor changes in the body’s natural isotope ratios. Since there is a natural abundance of stable isotopes, any isotopic tracers that are administered will add to the existing background. Measuring the background levels is thus very important when undertaking stable isotope tracer studies. Because stable isotopes tracers have mass, it is important that the quantities used are low so that the concentration of the molecules (or elements) being measured is not significantly changed, as this could affect the kinetics of the tracee. Since measurement techniques are very sensitive, this requirement is usually satisfied. The natural abundance of stable isotopes has been of great interest to geochemists for many years and the development of the Isotope Ratio Mass Spectrometer (IRMS), initially used in the geochemistry field, has allowed natural abundance measurements to be feasible in nutrition research. The notation used to describe natural abundance comes from a system developed by geologists and is based on the difference in isotopic abundance of a sample compared with an international standard; for carbon this was initially PeeDee Belemnite (PDB), a rock formation that had a particularly high 13C enrichment content. All measurements are normalised to the standard, so the output is a delta value (δ13C 0/00) or difference from the standard. On a practical note, a standard can be bought that has been verified against PDB in order to calibrate equipment. Natural abundance measurements are usually negative, because the 13C enrichment is usually less than the standard. However, a simple formula can convert the delta value to a TTR, which is more easily understandable and from which APE and MPE can be calculated. Figure 17.1 shows the subtle differences in the isotopic abundance of stable isotopes within an environment. For example, the enrichment of 13C in atmospheric CO2 is higher than in fossil fuels. Marine plants have different isotopic signatures from terrestrial plants, fish are different from meat and so on. There are also small but significant differences between plants determined by the carbon fixation pathways used (the so-called C3 versus C4/CAM [Crassulacean acid metabolism] plants). Maize is a C4 plant and is isotopically enriched with 13C, so should be avoided by volunteers prior to in vivo studies. An interesting point is that the difference in isotopic signature between the sugars in honey and cane or corn sugar can be used to detect the adulteration of honey with sugar. The same principle is also employed to detect adulteration in other foods. Figure 17.1 Range of carbon stable isotope natural abundance (in ‘delta notation’, see text for explanation) in environment and food sources. Photosynthesis favours the lighter 12C over the heavier 13C, so the carbon in the atmosphere contains more 13C than it would if there were no plants. Fossil fuels are derived from plant material and are therefore low (delta value more negative) in 13C enrichment. Redrawn from Mateo, M.A., Ferrio, J.P. and Araus, J.L. (2004) Isótopos estables en fisiología vegetal. In M.J. Reigosa, N. Pedrol and A. Sánchez (eds), La ecofisiología vegetal, una ciencia de síntesis, Paranimfo, Madrid, pp. 113–160. Fractionation of different isotopes can occur in biological reactions. This is because the strength of a chemical bond is dependent on atomic mass, such that bond strength increases with the substitution of heavier isotopes. An example is pyruvate dehydrogenase, which produces acetyl CoA, which is depleted of 13C relative to the precursor, pyruvate. Differences in the natural isotopic abundance of foodstuffs allow the habitual nutrition of animals and humans to be investigated; in addition, naturally enriched nutrients can be used to trace metabolism. With certain caveats, the isotopic analyses of tissues can indicate the relative contribution of C3 versus C4/CAM plants and C3 versus marine sources. For animals, this can distinguish between a grazer (C4) and a browser (C3). Using a combination of N and C isotopes can be more revealing, as carnivores have greater 15 N enrichment than non-meat-eaters. Dietary reconstruction using these techniques has been useful in ecology and paleontology and mathematical modelling can be employed to estimate dietary parameters. The natural abundance of 15 N enrichment has been used as an index of reduced nutrient intake. Isotopic signatures have been explored for use in studies of human nutrition and natural abundance measurements have the potential for distinguishing between meat versus fish, and the content of cane sugar and high-fructose corn syrup in the diet. Measurement of stable isotopic tracers in a biological matrix (blood, expired air, urine, faeces, tissue etc.) requires an instrument that can quantitate either the isotopic element, for example 46Ca, relative to the most abundant form of the element, or the tracer molecule, for example [1-13C]palmitate, relative to the unlabelled molecule, in this case palmitate (i.e. the tracee). This is a major advantage of stable isotopes over radioactive isotopes, since both labelled and unlabelled molecules (or elements) are measured simultaneously with very high precision. Instruments used are either nuclear magnetic resonance (NMR) or mass spectrometry (MS); the latter is often interfaced with an instrument that separates the tracee or molecule of interest, for instance gas chromatography (GC), liquid chromatography (LC) and so on. There are three components to a mass spectrometer: the ion source where the sample is ionised, the mass analyser that separates the ions and the detector (Figure 17.2). The mass analyser can be a magnetic mass analyser, a quadrupole mass analyser (or multiple quadrupoles, for example a linear series of three quadrupoles can be used, known as a triple quadrupole mass spectrometer), time-of-flight mass analyser, ion trap analyser or variations of these. Ions are separated according to their mass-to-charge ratio. There are different forms of ionisation depending on the phase (solid, liquid, gas) of the sample. Examples include electron ionisation and chemical ionisation for gases, thermal ionisation for solids and electrospray ionisation for liquids. Figure 17.2 Diagram of a mass spectrometer with a magnetic mass analyser. A sample is injected; the molecules are ionised and accelerated, then separated by mass and charge by the mass analyser. Used with permission from Thermo Fisher Scientific. An IRMS, which has a magnetic mass analyser, is set up to measure the isotopic enrichment of a gas. Samples must be processed before entering the ion source of the mass spectrometer, so that only a single chemical species enters at a time. This technique is used for measuring expired 13CO2 generated from the oxidation of a 13C-labelled molecule and from this the oxidation rate can be inferred. Non-gaseous samples can be converted to simple gases by, for example, combustion or pyrolysis and the gas to be measured is purified by traps, filters, catalysts and/or chromatography. Because of the high sensitivity of this method it is used to measure very low enrichments of tracer molecules, such as LDL apolipoprotein B enrichment (Section 17.9), and is also used in natural abundance studies (Section 17.2). GCMS is probably the most widely used method for measuring molecules labelled with a stable isotope. Their relatively small size, low cost and ease of use have made stable isotope tracer studies much more accessible. GCMS requires that the analytes first be derivatised, which can add considerable complexity to the method. LCMS can measure many metabolites directly with limited sample preparation. Tracers of minerals and trace elements are measured by thermal ionisation mass spectrometry or inductively coupled mass spectrometry. In the latter technique the sample is introduced through a nebuliser into high-temperature argon plasma, where it is volatilised and ionised. The suppliers of stable isotopes of minerals and trace elements are limited. There are a larger number of suppliers of isotopically labelled molecules and the cost varies depending on the site of labelling and the number of labels. If a specifically labelled molecule is not listed by a supplier, they can often manufacture a small batch for a client, although this is costly. While stable isotopically labelled vitamins are also available, the supply of some can be intermittent. An alternative approach used by some researchers is to grow plants in a labelled medium containing 2H or 15 N or in an atmosphere enriched with 13CO2. This produces plants with intrinsically labelled nutrients, including vitamins or provitamins, which can be incorporated into a meal. Intrinsic labelling of animal-derived macronutrients has also been used. The degree of enrichment obtained is not necessarily very high, for example a relative 13C enrichment of 1 · 4 APE was successfully used in a physiological experiment in humans, in which peas were labelled. Infusion of a 13C-labelled amino acid in cows has been employed to generate labelled milk proteins, which were used to assess protein digestion and absorption kinetics and the subsequent muscle protein synthetic response in humans. There are several different techniques and the choice of technique is dependent on the question to be answered. The principle of this technique is that if a known amount of tracer is added to a biological system, sampling from the body pools where the tracer is mixed, and measurement of its dilution will provide a measure of the size of the body pool. This has been used for the measurement of body composition (Section 17.14). To measure the exchange between body pools or ‘flux’, the dynamic isotope dilution technique is used. This technique requires the tracee to be constant during the study; that is, in a steady state. The tracer is usually infused intravenously (iv) at a constant rate. It is assumed that the tracer is diluted in a single body pool, such as plasma. An isotopic steady state is reached with time, this being determined by the half-life of the tracee and the size of the tracee pool. At this point the tracer is lost from this pool at the same rate as the tracee. The dilution of the tracer by the tracee at an isotopic steady state is a measure of the rate of appearance (Ra) of the tracee into the pool, which in a steady state is equal to the rate of loss from the pool (also known as the flux; Figure 17.3). Figure 17.3 Schematic illustration of isotope dilution. Redrawn from Wolfe, R.W. (1992) Radioactive and Stable Isotope Tracers in Biomedicine: Principles and Practice of Kinetic Analysis, Wiley-Liss, New York. F, tracer infusion rate; Ra, rate of appearance; Rd, rate of appearance In an isotopic steady state Tracer/tracee = F/Ra. In a steady state Ra=Rd. In a steady state it is assumed that Ra = Rate of disappearance = flux. The metabolic clearance rate (MCR) of the tracee, a measure of the efficiency of the tracee removal, is calculated as If the pool size is large relative to the flux of the tracee, the duration of tracer infusion will be prolonged. To reduce this, a priming dose (PD) can be administered. The aim of the PD is to instantaneously label the total miscible pool of the tracee, to the level that would be achieved by an unprimed constant infusion. The appropriate priming dose is calculated as A bolus injection of the tracer rather than a constant infusion can also be used, although care should be given to ensure that the dose used does not disturb the steady state of the tracee. The tracer will decay exponentially with time and curve-fitting techniques are required. This method is usually combined with a mathematical model to describe the dynamics of the tracer. An exception to this is the use of labelled H2O to measure total energy expenditure (Section 17.13). The isotope dilution technique can also be used in non-steady-state modelling. An isotopic steady state is achieved first with the tracer infusion and with the tracee also in a steady state. The steady state can then be disturbed, for instance with the infusion of a hormone, the subject exercising or eating a meal. From the change in TTR and concentration with time, the change in the flux of the tracee can be determined using non-steady-state equations or mathematical modelling. If a 13C-labelled metabolite is administered, the appearance of 13C in CO2 can provide a measure of the oxidation rate of that metabolite. Expired air is collected in small bags or tubes and the 13CO2 content is measured by IRMS. A measure of the CO2 production rate is also needed to calculate the 13CO2 expiry rate. This can be obtained using a gas analyser when collecting the samples. When combined with the isotope dilution technique, this can provide a quantitative measure of the tracee oxidation rate if a steady state of 13CO2 is achieved. The main consideration with this method is correction for any fixation of 13CO2 in the body bicarbonate pool and other metabolic pathways. To measure fatty acid oxidation, loss of 13CO2 in the Krebs cycle must be corrected for using an acetate recovery factor. This is estimated from a 13C2-labelled acetate infusion in a separate study, which mimics the conditions employed for the determination of substrate oxidation. When using 13C-labelled tracers to measure oxidation rates if there is a change in the metabolic state during the study, care must be taken to ensure that any increase in 13CO2 expiry rate is due to the oxidation of the tracer and not due to a change in the background enrichment. For example, the infusion of (unlabelled) dextrose, which is derived from corn starch, during a euglycaemic hyperglycaemic clamp will increase the 13CO2 expiry rate, simply because the glucose infused has a higher natural abundance of 13C than the body’s glucose stores. This can compromise the results if the experimental protocol includes the measurement of 13CO2 expiry rate from a 13C-labelled stable isotope tracer such as [13C]leucine. To overcome this, potato starch can be used, which has a natural abundance similar to the body’s glucose stores. The appearance of 13C in CO2 after administration of an oral 13C-labelled tracer can be used to provide information about gastric emptying, oro-caecal transit time, gut bacterial overgrowth, fat and protein digestion, and clinically for the diagnosis of infection with Helicobacter pylori. This technique is used for measuring oral absorption. A tracer of the molecule or element of interest is given orally with a meal and a tracer that is distinguishable from the oral tracer is given iv. This method has been used in the study of mineral absorption and cholesterol absorption. A modification is employed to measure postprandial glucose metabolism. Mathematical models are required to interpret the data. This technique is used for measuring macromolecule synthesis rates. It is based on the assumption that the fractional rate of synthesis of a product can be measured if the TTR of the precursor is known and the TTR of the product is measured. If the absolute concentration of the product is also measured, then the absolute synthesis rate can be calculated. The difficulty with this method is that the TTR of the precursor is not always known. One approach to calculating this is using mass isotopomer distribution analysis (MIDA). This method determines the precursor enrichment from the pattern of labelling in the product (Section 17.11). Stable isotope studies of mineral and trace element metabolism have made a significant contribution to the quantification of their absorption and metabolism and have led to important dietary recommendations. Stable isotopes are available for most of the minerals necessary for mammalian life. The first studies using stable isotopes were in the 1960s. Methods for the measurement of mineral absorption and kinetics are now well established, especially for calcium. Stable isotope tracers are administered orally and/or iv, with the measurement of the tracers in blood, urine and faeces. The standard method for assessing calcium absorption from a single meal is the dual tracer technique. A stable isotope of calcium is given orally with a meal and a second isotope is given iv as a short infusion over a few minutes. The isotopes used are usually 42Ca, 44Ca or 46Ca, although both 43Ca and 48
Stable Isotopes in Nutrition Research
17.1 Introduction
17.2 Natural abundance
Habitual nutrition
17.3 Measurement techniques
17.4 Availability of tracers
17.5 Stable isotope tracer techniques
Isotope dilution
Oxidation rates using 13C-labelled molecules
Dual isotope method
Precursor product methods
17.6 Trace elements/mineral absorption, body utilisation and turnover
Stay updated, free articles. Join our Telegram channel

Full access? Get Clinical Tree
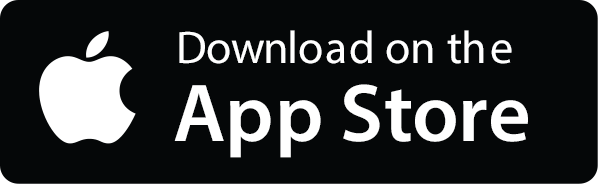
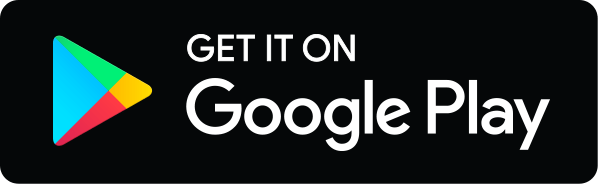