© Springer Science+Business Media New York 2015
Ashraf Khan, Ian O. Ellis, Andrew M. Hanby, Ediz F. Cosar, Emad A. Rakha and Dina Kandil (eds.)Precision Molecular Pathology of Breast CancerMolecular Pathology Library1010.1007/978-1-4939-2886-6_1919. Specimens for Molecular Testing in Breast Cancer
(1)
Department of Pathology, University of Massachusetts Medical School, UMass Memorial Medical Center, Three Biotech, One Innovation Drive, Worcester, MA 01605, USA
Keywords
Specimen typesMolecular testingBreast cancerBackground
Molecular testing can be performed on different specimen types. DNA- and RNA-based ancillary studies play an important role in identifying molecular portraits of breast carcinoma and in influencing routine therapies. Preanalytical factors including different fixatives, processing methods, and storage conditions coupled with differences in chemical and physical conditions, including time to fixation, mechanism of fixation, processing temperature and pH, storage time and conditions, all have a significant impact on the quality of the nucleic acids [1–6].
Specimen Types
Commonly used specimen types for molecular testing include fresh, frozen, formalin fixed paraffin embedded (FFPE), or alcohol-fixed samples. Nucleic acid extraction for molecular testing should be validated for each of these specimen types. Similarly, molecular assays used for clinical purposes should be limited to validated specimen types. For breast carcinoma, molecular analysis is usually performed on needle core biopsies or resection specimens, but could also include cytology samples, such as fine needle aspiration (FNA) biopsy [4, 7]. Both core biopsies and large resection specimens are usually fixed before molecular analysis is performed to allow correlation with morphology. Guidelines have been introduced to standardize the processing of breast specimens [4, 5]. To achieve optimal performance, breast resection specimens should ideally be sectioned at 5-mm thickness after appropriate gross inspection and margin designation [4, 8, 9]. Preanalytical conditions may adversely impact molecular assays. The cold ischemia time should be kept to a minimum to reduce RNA and protein degradation. The optimum formalin exposure time for breast tissue specimens varies and is based on the specimen size, but in practice, the consensus is that at least 1 hour (h) of fixation per mm of tissue thickness is required [2, 6, 10, 11]. Strict adherence to these guidelines is crucial to obtain the most accurate results for predictive and prognostic tumor markers. Failure to adhere to these guidelines may significantly affect the clinical management and potentially impact the outcome.
Preanalytical Factors
There are many different parameters affecting optimal tissue preservation. Not all of them have been well studied; however, there is a wealth of evidence documenting some of the more important variables [6]. Here, we briefly touch on the more important preanalytical factors for breast specimen handling:
1.
Prefixation time, which includes warm ischemia and cold ischemia time or the postmortem interval, has a great impact on the quality of the DNA and RNA in breast specimens. Optimally, cold ischemia should be less than 1 h for fluorescent in situ hybridization (FISH) and should be less than 24 h for polymerase chain reaction (PCR). In instances when a specimen falls outside of these parameters, the inclusion of an internal control gene to ascertain the specimen integrity is recommended. The latest American Society of Clinical Oncology/College of American Pathologists (ASCO/CAP) guidelines recommend the time to fixation for breast specimens not to exceed 1 h [2, 12–14].
2.
Fixation parameters:
The following factors have been well studied:
(a)
Fixation chemistry: The mechanism of action for fixatives may be classified as cross-linkers, dehydrants, heat or acid effects, or a combination of these. In breast, as in other tissue types, 10 % neutral-buffered formalin (NBF) is the most widely used universal fixative as it preserves a wide range of tissues and tissue components [15, 16]. Unbuffered formalin fixation is not recommended as this method produces poor DNA yield and quality [17]. The amount of DNA fragmentation associated with formalin fixation is greater than that observed with alcohol fixation [16, 18].
(b)
Tissue penetration: This variable depends on the diffusibility of the fixatives. The general consensus for 10 % NBF in breast specimens is 1 mm of penetration per hour [2, 6, 19, 20]. Compared to formalin, alcohol leads to more rapid fixation, which offers better preservation of labile RNA molecules [21]. Overall the volume of fixative should be in excess of 20 times the volume of the tissue, however, this ratio can be difficult to achieve and requires proper sectioning of the large resections [22].
(c)
Fixation conditions: Few studies have investigated the optimal temperature and pH necessary to preserve different nucleic acid types. However, the general consensus for the optimal fixation of breast specimens is cold temperature (optimum 4 °C, room temperature acceptable) and the use of nonacidic fixatives such as 10 % NBF. Other conditions lead to more nucleic acid degradation and reduced nucleic acid yields [6, 14, 23].
(d)
(e)
Decalcification: Decalcification methods can affect DNA, RNA, and protein integrity. Methods such as PCR amplification, probe binding, in situ hybridization (ISH), and protein analysis can be adversely impacted. Decalcification using ethylenediaminetetraacetic acid (EDTA) solution, especially in combination with ultrasound when available, is superior to other methods of decalcification [12, 24–27].
3.
Postfixation parameters: Relatively, few studies address the issue of quality control and assurance metrics for the appropriate storage of paraffin blocks [31, 32]. In particular, the storage time of FFPE blocks has been shown to adversely affect the nucleic acid quality. Formalin fixation and residual water exposure can have detrimental effects on nucleic acids, especially RNA [19, 21]. This specifically applies to tissue blocks that are older than 5 years for DNA studies and older than 1 year for RNA studies [4, 6, 9, 20]. Therefore, it is necessary to consider these adverse effects when selecting the blocks for molecular studies. As for the stored sections from FFPE tissue blocks, guidelines recommend molecular testing within 6 weeks for clinical purposes [4, 9].
Fixative Types
NBF (10 %) is the preferred and most widely used fixative, and molecular assays have been optimized for this fixative. It has been shown that formalin fixation forms chemical cross-links between proteins, DNA, RNA, and other macromolecules and leads to fragmentation of DNA that adversely affects molecular testing in a length-dependent manner. Molecular analyses that require amplicons approaching 1000 base pairs (bp) are usually successful when nucleic acids are extracted from fresh or frozen tissue samples, but are far less reliable in formalin-fixed specimens. Consequently, most assays are designed to utilize DNA sequences shorter than 300 bp to obtain satisfactory results [6]. In addition, random nucleotide base changes introduced by formalin fixation may be detected by more sensitive assays, especially in samples with low DNA concentration, and this may lead to false-positive results [6, 33, 34]. Any breast tissue specimen collected for molecular testing (cytology samples, needle biopsies, resection specimens) should be immersed in a sufficient volume of 10 % NBF as soon as possible (time to fixation ideally within 1 h). Several studies have shown that a delay greater than 1 h may lead to erroneous results in HER2 FISH testing [2, 10]. Resection specimens should be sectioned through the tumor upon receipt. Cold ischemia time, type of fixative, and the time the sample was placed in fixative must be documented. Evidence suggests that the optimal duration of fixation for 10 % NBF is between 6 and 72 h [4, 8]. Alternative fixatives containing heavy metals such as mercury chloride (B-plus, B5, and Zenker) or acid zinc formalin and acidic solutions (Bouin and bone decalcifier) have detrimental effects on nucleic acids. This is also true for unbuffered formalin, because spontaneous oxidation of formalin, in time, produces formic acid. Heavy metals can have inhibitory effects on enzymes, which are routinely used in molecular assays. For instance, heavy metals inhibit DNA polymerases through competition with magnesium, an essential cofactor for polymerase activity [35]. On the other hand acidic solutions can “nick” the DNA backbone producing tiny fragments and cause depurination, making them unsuitable for testing [6]. If a sample gives a negative result in the absence of internal control elements, testing should be repeated on an alternate sample, such as different tissue block or specimen [4, 9].
Detection of ERBB2 (HER2) Gene Amplification by In Situ Hybridization (ISH) Assay
ERBB2 (HER2) gene status may be assessed by various ISH methods including fluorescence or bright field in situ hybridization. These are molecular cytogenetic techniques that identify HER2 gene amplification [1, 4]. Reverse transcriptase real-time PCR assays employed by Oncotype Dx also provide information about HER2 gene mRNA levels, but are not routinely used to make treatment decisions for HER2 targeted therapy. A variety of factors influence the performance of these assays. Time to fixation will have a different effect depending on the specific nucleic acid analysis platform [2, 12, 36]. A cold ischemia time of more than one hour leads to reduced FISH signals [2]. RNA-based assays are even more sensitive to this variable [37–39]. HER2 testing can be compromised by overfixation as well. The current ASCO/CAP guidelines recommend using 10 % NBF with tissue fixed for 6–72 h [4]. Needle core biopsies fixed for less than 1 h or resections fixed for less than 6 h should not be tested [4, 14]. Fixation with alcohol results in DNA condensation and this may influence FISH interpretation and should be considered when alcohol fixative is used [28]. On the other hand, DNA cross-linking, which commonly occurs with formalin fixation, may prevent probe penetration and binding to target DNA resulting in faint signals [6, 12]. Enzymatic digestion times for ISH can be modified to optimize the probe signal intensity. Overdigestion with proteases may result in split or fragmented signals that can lead to misclassification as gene copy number gain [4, 14].
Other Routine Molecular Assays
Aside from the HER2 gene FISH testing, there are several other prognostic and predictive gene sets in breast cancer. Reverse transcription polymerase chain reaction (RT-PCR) is a relatively recent approach to detect HER2 gene amplification in breast cancer. This approach has also been utilized in well-known and widely used FDA approved assays, such as Oncotype Dx (Genomic Health Inc, Redwood City, California), MammaPrint (Agendia, Amsterdam, the Netherlands), and PAM50 (Nanostring Technologies Inc., Seattle, Washington) . All 3 tests can provide an overall risk assessment of breast cancer recurrence [40–43]. These assays have benefited greatly from standardization of fixation practices, designed to ensure consistent results for HER2 and estrogen receptor (ER) and progesterone receptor (PgR) and other ancillary studies [43, 44].
Oncotype DX is a multiplex, 21-gene, RT-PCR assay optimized for quantification of RNA extracted from FFPE tumor tissue that predicts the likelihood of disease recurrence (recurrence score- RS) in women with stages I or II, hormone receptor-positive, lymph node-negative, invasive breast cancer [41, 45].
MammaPrint is a 70-gene expression profile that was initially developed using oligonucleotide expression array (25,000 genes). Analysis consisted of consecutively collected tumor specimens from a cohort of women with stage I or II breast cancer who had undergone definitive surgery only without systemic therapy and with at least 5-year clinical follow-up [46, 47]. Originally, Mammaprint assay was limited to fresh tissue for assessment, but recently specimens fixed in 10 % NBF have been validated [48]. These assays heavily rely on careful primer design to minimize the effects of RNA degradation [48].
Prediction Analysis of Microarray (PAM) 50 is a 50-gene breast carcinoma subtype predictor, which was initially developed using microarray and quantitative RT-PCR. PAM50 measures the expression levels of 50 genes in surgically resected breast carcinoma samples. The risk of recurrence (ROR) can be determined in both patients without previous systemic therapy, and with systemic therapy. The PAM50 gene set is therefore used for gene expression-based subtyping of breast carcinomas and for probability of disease recurrence [49–51].
Other Molecular Assays
Other than commonly used molecular assays, there are some other methods that have gained popularity in recent years. As mentioned in the previous section, RT-PCR can be used to test for the HER2 gene status in breast carcinoma specimens. When proper fixation protocols are followed, studies have shown a concordance rate of 92–94 % between FISH and RT-PCR. Some studies even proposed that RT-PCR may represent a better methodology than FISH, especially for equivocal samples by IHC [52–54]. HER2 gene amplification is highly associated with HER2 mRNA overexpression, and quantification of HER2 mRNA by RT-PCR shows strong agreement with HER2 gene amplification by FISH [55]. Compared with FISH, which offers semiquantitative values, RT-PCR offers quantitative values [44]. Despite these advantages, the labile nature of RNA introduces the risk of false negative results and therefore is not widely accepted as a clinical test to assess HER2 gene status. This method is also limited by the need for a nearly pure invasive tumor cell population, which may require expensive and specialized methods, such as laser capture microdissection (LCM). Microarray-based expression profiling has similar limitations. In addition, this method provides only a semiquantitative assessment of gene expression, and sensitivity and specificity are limited by the nature of the probes included in the platform [56, 57].
Various DNA-based methods can be used to test for gene copy status in breast carcinoma specimens. Comparative genomic hybridization (array CGH) and single nucleotide polymorphism (SNP) array analysis have provided a wealth of data on gene copy number aberrations in breast cancer and have helped to identify potential therapeutic targets for subgroups of breast cancer patients. However, these array technologies do not provide any information about structural genomic aberrations. SNP array can provide information about nucleotide base pair variations [58, 59]. These array technologies require high quality DNA and consequently FFPE tissues may be unsuitable for these methods. Therefore, gene copy number information has been primarily derived from fresh or frozen tissue [60]. For these reasons and the high cost, these methods have not been adopted into routine clinical practice.
MicroRNAs
MicroRNAs are endogenous, small noncoding RNAs, which regulate gene expression by directly binding to the untranslated regions of the target messenger RNAs and may act either as tumor suppressor or oncogene [57, 61–64]. Levels of specific miRNAs differ between normal and malignant breast tissue and among tumors of varying histological grade, molecular subtype, lymph node status, and hormone receptor (HR) status [65, 66]. In addition, miRNAs have been linked to breast cancer invasion, proliferation, and metastasis [67–69]. Different miRNAs have been shown to be associated with aggressiveness of breast cancer or work as a tumor suppressor [1, 61]. The available miRNA technologies are not without limitations, such as the susceptibility to degradation associated with all types of RNA. Because of their smaller size, miRNAs are relatively less affected by the damaging effects of formalin fixation and can readily be extracted from FFPE tissue [70]. miRNAs have been discussed in greater detail in Chap. 13.
Future Directions
Next-generation sequencing (NGS), also known as massively parallel sequencing, has been rapidly gaining popularity in clinical testing. The Cancer Genome Atlas (TCGA) project has revealed a spectrum of diverse genomic anomalies through exome and whole genome sequencing [58, 71]. NGS is changing our understanding of the molecular traits of tumors at the genomic, transcriptomic, and epigenetic levels [72, 73]. The data from whole genome sequencing can provide a global view of individual tumor biology by integrating a variety of molecular information [73, 74]. The ability to distinguish between driver and passenger mutations is helpful in determining which genes are important in tumor development and therapeutic resistance [75]. Genetic heterogeneity in breast cancers has been confirmed and further reinforced by NGS methods [75, 76]. NGS can be used to analyze the entire genome or to target the gene regions containing high-frequency mutations. The latter targeted approach can provide high-level sequencing coverage and allows detection of low level mutations. The value of NGS in clinical testing is further emphasized by the capacity to sequence small specimens with scant tumor cells, such as samples obtained by FNA. In contrast to traditional Sanger sequencing, NGS also has the advantage of multigene panel testing using as little as 10 nanograms/microliter of DNA [77, 78]. With proper design, these systems are less prone to the adverse effects of formalin fixation and associated DNA fragmentation. The availability of customizable multigene panels has enabled the identification of mutations that may serve as early indicators of disease and may be used to monitor disease progression and therapeutic response [75]. Clinical predictors based on the molecular signature and detection of the vulnerabilities of an individual’s tumor to facilitate precision medicine represent promising opportunities, such as personalized therapy, to improve cancer care [79–81].
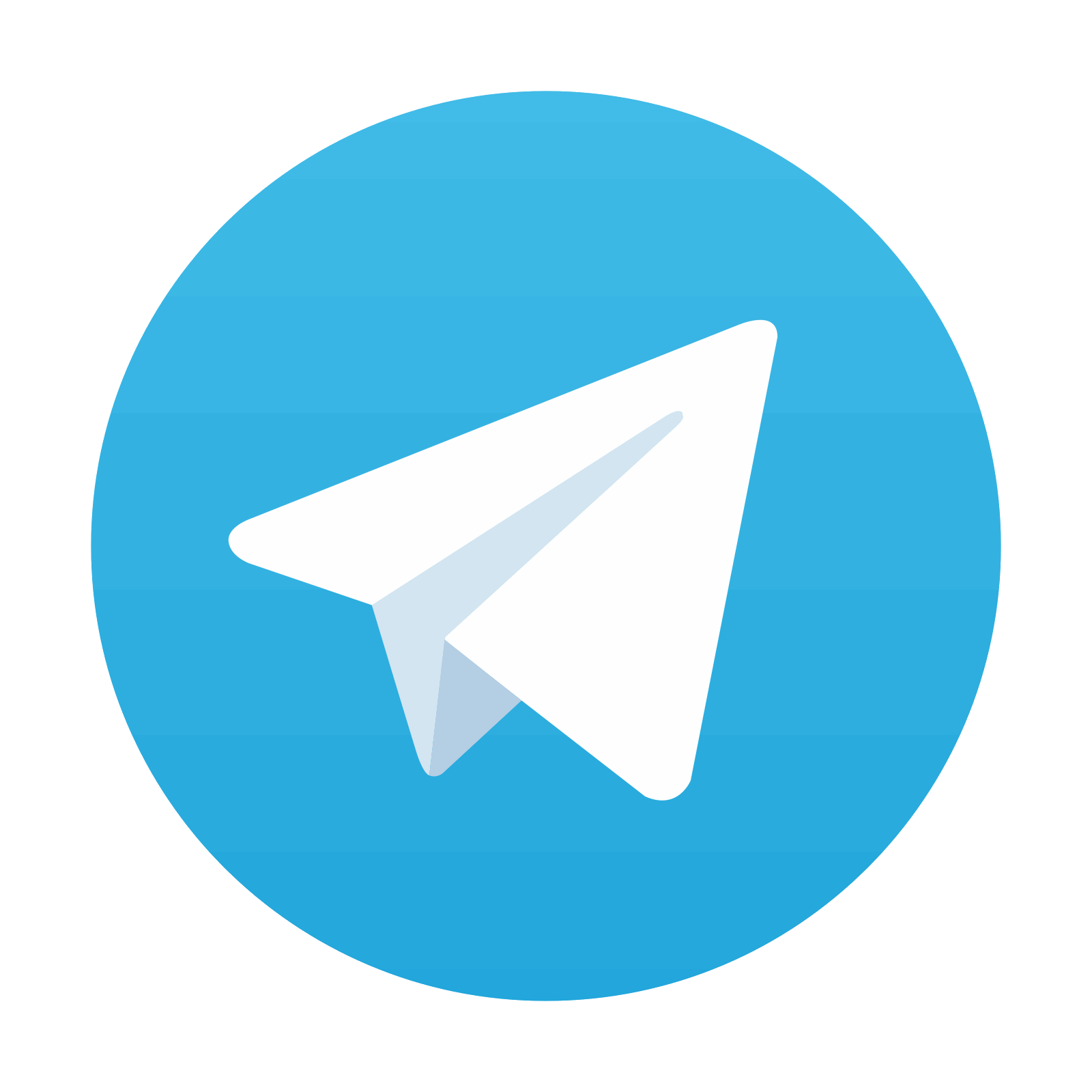
Stay updated, free articles. Join our Telegram channel

Full access? Get Clinical Tree
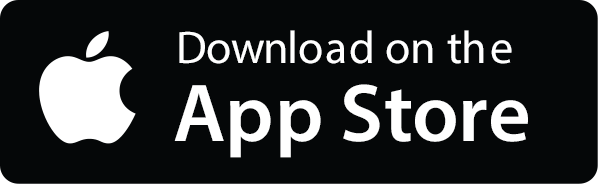
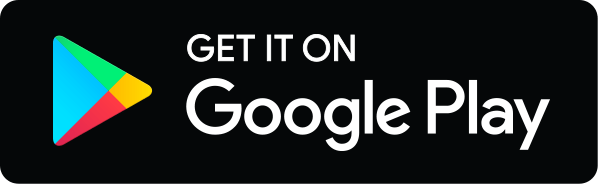