© Springer International Publishing AG 2018
Anita Mahajan and Arnold Paulino (eds.)Radiation Oncology for Pediatric CNS Tumorshttps://doi.org/10.1007/978-3-319-55430-3_2828. Special Radiation Techniques for Pediatric Brain Tumors: Hypofractionation, Radiosurgery, and Brachytherapy
(1)
Department of Radiation Oncology, Stanford University School of Medicine, 875 Blake Wilbur Drive, Stanford, CA 94305-5847, USA
Abstract
Radiation is an important component in the treatment of pediatric brain tumors. Because of the concern for late radiation complications, innovative strategies to improve radiation effectiveness and reduce toxicity have been investigated. Here, we describe hypofractionation, stereotactic radiosurgery, and brachytherapy as advanced radiotherapeutic technologies and strategies currently in use in the treatment of pediatric brain tumors.
28.1 Introduction
Radiation therapy is an important component of the overall treatment paradigm in many tumors of the pediatric central nervous system (CNS). However, the use of cranial radiotherapy in children is associated with concern for long-term morbidity including neurocognitive and growth effects, and risk of secondary malignancy (Merchant et al. 2005, 2009; Shih et al. 2009; Vern-Gross et al. 2014; Lawrence et al. 2010). As such, efforts have been made to reduce or omit the use of cranial radiotherapy in children where possible. Yet, the omission of radiation in the treatment of certain pediatric CNS malignancies has been associated with higher relapse rates in a number of trials (Balmaceda et al. 1996; Kellie et al. 2004), thereby leading to its continued incorporation despite the risk of side effects. Importantly, many of the studies that reported deleterious side effects associated with radiation included patients treated with older radiation techniques, thereby potentially overestimating the magnitude of the deficits associated with current techniques. Recent advances in technology allow for more precise localization with image guidance and smaller margins, thereby reducing the volume of brain receiving radiation and decreasing the dose received by adjacent areas, while allowing the safe delivery of a larger dose per fraction. We discuss here advanced radiotherapeutic technologies and strategies currently in use in the treatment of pediatric brain tumors, and outcomes in specific disease sites.
28.2 Treatment Types
Hypofractionated radiotherapy refers to the shortening of a course of external beam radiation therapy (EBRT) into fewer fractions with higher dose per fraction, while generally maintaining equal or greater biologically effective dose (BED). Interest in hypofractionation first developed in the 1950s and continued through the 1970s, largely due to increased demand for adjuvant radiation in breast cancer and limited resources to provide this care. However, a high rate of side effects associated with early hypofractionation strategies, including skin fibrosis and brachial plexopathy, limited the adoption of this strategy (Ko et al. 2011; Friberg and Ruden 2009). While hypofractionation has obvious practical benefits such as shortening treatment courses and improving the convenience to patients and families, it also has important radiobiological effects (Slotman et al. 1996). The basis of the radiobiological effect of hypofractionation continues to be a source of debate, with some arguing that effects can be predicted by the linear-quadratic (LQ) model, and others arguing that the effect of hypofractionation is greater than that predicted by the LQ model. This greater radiobiological effect sometimes reported with hypofractionation may be related to multiple factors, including differential radiosensitivities of the heterogeneous population of tumor cells and the potential role for immunologic effects in modulating radiation-induced damage (Ko et al. 2011; Yu et al. 2015). Furthermore, shortening of treatment time may reduce tumor cell repopulation during treatment, particularly important in aggressive CNS tumors with rapid doubling times (Iuchi et al. 2014). In employing a higher dose per fraction, the use of hypofractionation may also allow for the effective treatment of tumors previously considered radioresistant. However, these putative benefits must be weighed against the potential short-term and long-term side effects of hypofractionated therapy, which are less well understood than conventional treatment protocols (Iuchi et al. 2014).
Stereotactic radiosurgery (SRS) was developed in the early 1950s as a method of treating an intracranial lesion with an ablative dose of radiation, made possible by the ability to precisely localize the target, often utilizing real-time tracking (Leksell 1951). This precise localization allows for treatment with little to no margin around the tumor and with extremely steep dose falloff. Multiple types of SRS exist, including Gamma Knife radiosurgery (GKS), which uses multiple cobalt-60 sources and directs these sources to a central target at the isocenter, with an accuracy of 0.3 mm. GKS requires an invasive head frame for immobilization and depending on the contour of the volume to be treated may require multiple isocenters. In contrast, linear accelerator-based (linac-based) SRS utilizes a single X-ray source that moves around the patient, with the collimator having the capability of using cones or multi-leaf collimators, enabling treatment with static fields or moving arcs. The couch is also mobile, allowing correction for translational and rotational errors. Additionally, since linac-based SRS can be performed with a noninvasive mask instead of frame-based immobilization, treatment fractionation is more feasible.
Brachytherapy is a specialized form of internal radiation therapy which, in the setting of CNS disease, is delivered in an interstitial manner and generally involves implantation of iodine-125 seeds. This treatment will deliver dose directly to the area of implantation, but with a rapid dose falloff thus minimizing dose to the adjacent normal brain. The procedure is typically performed immediately after biopsy, often using a target software program (Ruge et al. 2011a). In contrast to externally delivered radiotherapy, brachytherapy provides prolonged low-dose activity, potentially minimizing toxicity while providing consistent and ongoing treatment of the lesion in question (Peraud et al. 2007).
28.3 Considerations in Pediatric Patients
Special considerations must be given to pediatric patients, in terms of practical aspects of the logistics of therapy, as well as in attention to long-term effects. The typical radiosurgical and radiotherapeutic considerations require modification for the treatment of children, particularly in the area of immobilization. Frame-based techniques typically require skull fixation with pinning for the precise localization of the target volume, in order to ensure safe and accurate delivery of a higher dose of radiation. The thinner and relatively flexible skulls of young children require extra consideration in pinning to avoid damage to normal tissues. In addition, the thin skull of a child is more deformable than that of an adult, potentially complicating accuracy of treatment. Attempts have been made at noninvasive immobilization for frame-based SRS, with trials of various head immobilization techniques, including helmet techniques attached to the upper teeth (Greitz et al. 1980; Kingsley et al. 1980). However, these may make airway access for anesthesia difficult or impossible. Other immobilization devices without skull fixation have been studied, but may produce errors up to 3–4 mm, precluding the safe and accurate delivery of high-dose radiation in a single fraction (Kooy et al. 1994). Robotically controlled radiosurgical systems such as CyberKnife use a frameless delivery system that relies on an advanced fusion algorithm to provide similar accuracy to frame-based systems (Giller et al. 2007). This produces comparable accuracy as frame-based systems using a noninvasive approach and a simple custom face mask, which can be modified to provide access for anesthesia (Murphy and Cox 1996).
Another important consideration in the treatment of children is the frequent need for anesthesia which can be particularly challenging when combined with certain immobilization techniques. Even older children undergoing GKS or frame-based radiotherapy in which immobilization is paramount may require general anesthesia, increasing the risks associated with therapy. In a study of 68 pediatric cases of anesthesia and stereotactic radiosurgery, there were four serious events associated with procedures (Stokes et al. 1995). Because of the risks and challenges associated with daily general anesthesia, the fact that SRS is completed in one to five fractions is particularly appealing in the pediatric patient population.
Despite the practical benefits of hypofractionating therapy in children with CNS disease, there remains the hypothetical concern for increased side effects, such as brain necrosis. Image-guided hypofractionated or SRS approaches treating smaller fields and with less volume of the adjacent brain receiving radiation may help limit such toxicity and are also beneficial in considering the known radiation dose–volume effects in the brain in children (Lawrence et al. 2010). Another major concern in treating children with CNS-directed radiation is the risk of secondary malignancies later in life. However, models have estimated a relatively low risk of secondary malignancy after SRS, which is less than the risk of death from general anesthesia (Rowe et al. 2007). Finally, the optimal dosing of hypofractionated or SRS in pediatric patients remains to be determined. Concerns for late effects have prompted some attempts to decrease doses by approximately 25% in pediatric patients (Giller et al. 2007). However, such dose reductions have been reported to result in inferior local control as compared to standard adult doses (Smyth et al. 2002). The rate of serious adverse events such as radiation necrosis remain relatively low at this time and has prompted some to increase radiation doses to the adult level (Tanaka et al. 1996).
28.4 Individual Applications of Hypofractionation and Stereotactic Radiosurgery
28.4.1 Malignant Disease
28.4.1.1 Brainstem Glioma
Diffuse Intrinsic Pontine Glioma
Perhaps the best-known use of hypofractionation in pediatric CNS tumors is in the setting of diffuse intrinsic pontine glioma (DIPG). Despite many trials of systemic therapy and various radiotherapeutic alterations including hyperfractionation, conventionally fractionated radiation therapy has remained the standard of care (Vallero et al. 2014). The standard course of radiation typically involves 6 weeks of daily radiation, but outcomes remain poor with most children surviving less than a year after diagnosis. A small retrospective case series found that hypofractionation (45 Gy in 15 fractions) had no major effect on survival and was reasonably well tolerated. However, several patients required steroids and/or reduction of the daily fraction size from 3 to 2 Gy (Negretti et al. 2011). Similarly, another study of hypofractionated therapy (13–16 fractions) with a matched-cohort analysis comparing conventionally fractionated radiation showed comparable overall survival (9.0 vs. 9.4 months in the hypofractionated and conventional therapy groups, respectively) (Janssens et al. 2013). Toxicity was comparable between the two groups. In the largest study to date of hypofractionated therapy for DIPG, Zaglhoul et al. conducted a trial of 71 patients randomized to either 39 Gy in 13 fractions or 54 Gy in 30 fractions. Although the study failed to demonstrate statistical non-inferiority of hypofractionation, the two groups had comparable results. Hypofractionation had a worse median overall survival (7.8 vs. 9.5 months) but better 1-year survival (36.4% vs. 26.2%) (Zaghloul et al. 2014). As in other studies, there was no significant difference in toxicity between the two groups. In summary, hypofractionation appears to yield similar outcomes and toxicity to conventional therapy, while significantly reducing the duration of treatment. Therefore, hypofractionation appears to be a reasonable and safe approach, particularly in patients who may have difficulty attending treatment, or who wish to minimize the burden of treatment in this disease with a relatively short overall survival.
Focal Brainstem Glioma
Focal brainstem gliomas are a distinct subset of brainstem gliomas with a more indolent course and better prognosis, yet resection is technically challenging or impossible, and the tumor location commonly poses issues due to local progression. The use of SRS has been explored as both an adjunct and an alternative to surgery. A retrospective review of 20 patients treated with GKS from 1990 to 2001 suggests that SRS may be an effective treatment for focal brainstem glioma. A prescribed dose of 10–18 Gy was delivered, with tumor shrinkage in 12 patients and complete remission in 4 patients. As in other studies of SRS, intratumoral changes in magnetic resonance enhancement were frequently seen but did not correlate with response (Karger et al. 1997; Kihlstrom et al. 1994). Side effects were generally well tolerated, though there was one patient who despite responding radiographically, developed fluctuating impairment of consciousness (from somnolence to coma) lasting for 6 months (Yen et al. 2007). These results were similar to another retrospective report of 12 patients with focal brainstem glioma treated with GKS, with mean peripheral dose of 12 Gy, where responses and stable disease were seen in 6 and 5 patients, respectively (Fuchs et al. 2002).
The radiosurgical experience in young children with focal brainstem glioma is limited. There is concern for increased toxicity in these children given ongoing neurologic development. Encouraging results have been reported in a case report of two children, ages 2.5 and 5.5 years. In each case, the tumor was located in an area not amenable to microsurgical resection. These patients were successfully treated with GKS with a mean tumor dose of 14–16 Gy and peripheral doses of 11–12 Gy. Both children showed remarkable tumor shrinkage and no adverse neurologic outcomes, at follow-up of 4 and 8 years (Liao et al. 2012).
Thus far, the limited evidence in pediatric focal brainstem gliomas has found stereotactic radiosurgery is both a safe and effective alternative to surgery in select cases. In cases where treatment-related toxicity occurs, they appear to be most commonly transient neurological deficits that resolve over time (Sharma et al. 2008).
28.4.1.2 Ependymoma
The standard treatment for ependymoma involves surgery followed by postoperative radiation therapy. Given favorable outcomes, effort has been made toward reducing radiation fields in the setting of nondisseminated disease. The primary pattern of failure in pediatric ependymoma patients is local, with extent of surgery reported by multiple groups to be the most important prognostic factor in survival (Robertson et al. 1998). Radiation dose also appears to be important in outcome particularly in those patients who have undergone subtotal resection (Lo et al. 2006). Two trials of SRS boost in the primary management of unfavorable ependymoma have been reported with favorable results. In a study of patients with residual tumor measuring 3 cm or less in diameter following initial surgery and chemotherapy or following conventional radiation, SRS boost was prescribed to the 80% isodose line with maximum dose ranging from 11.25 to 18.75 Gy. Of a total of five patients, four patients who received SRS within 30 days of primary radiation remained alive without progression of disease at a median of 24 months post-treatment (Aggarwal et al. 1997). No acute neurotoxicity was noted in any patient though one patient developed an apparent subacute reactive process which required treatment with short-term steroids with subsequent resolution of symptoms (Aggarwal et al. 1997). A single patient was treated with SRS boost 6 months after initial external beam radiation therapy, and this was the only patient who developed progressive disease. A report of the Washington University experience in treating nine children with unfavorable ependymoma found similar benefit to incorporating SRS in primary therapy. In four patients treated with SRS as part of primary therapy, none had experienced relapse at 3 years. Dose was 14–18 Gy prescribed to the 50% isodose line for patients treated with GKS, and 14–20 Gy to the 80–90% isodose line for patients treated with linac-based SRS, with only two severe side effects noted, including one patient with post-treatment seizure disorder and one patient with facial nerve palsy (Mansur et al. 2004). A phase II trial which planned to incorporate SRS boost after conventional EBRT if there was residual enhancement of the tumor following EBRT would have helped assess the benefit of SRS in unfavorable ependymoma, but unfortunately the study was closed due to poor accrual (Lo et al. 2006).
The use of hypofractionation and SRS has been more widely reported in the setting of recurrent ependymoma. Liu et al. reported the outcomes of six children with locally recurrent ependymoma treated with maximally safe resection, followed by hypofractionated irradiation on a linear accelerator to 24–30 Gy in three fractions. With a follow-up of 28 months, all six children are alive without evidence of disease, with three children having evidence of radiation necrosis clinically or on imaging, but none requiring intervention (Liu et al. 2009). At the Mayo Clinic, 26 patients with recurrent ependymomas had SRS, 8 of whom were under the age of 18 years. The investigators report 3-year local control rate of 72%, and 2 patients (8%) with symptomatic radiation necrosis after SRS, both of whom had undergone previous EBRT (Stauder et al. 2012). Another study of recurrent ependymomas treated with SRS was reported by the Pittsburgh group. Among 39 patients with a median age of 22.8 years, 32.1% of patients remained alive at 5 years after SRS, with a progression-free survival of 45.8%. It appeared that smaller volume and homogenous contrast enhancement were significant predictors of response to SRS, and other groups have reported this as well. Though children are generally thought to have poorer outcomes than adults with more aggressive anaplastic ependymoma, there was no significant difference seen by age in this study (Kano et al. 2010). Others have found less encouraging results in the setting of recurrent ependymoma. In one study which included 25 patients with recurrent ependymoma, only 12% remained disease-free on follow-up (Hodgson et al. 2001), while another study of 7 patients treated with SRS for recurrence found none remained in remission on follow-up (Grabb et al. 1996).
Disseminated recurrence can also occur in the setting of ependymoma, with leptomeningeal involvement more common in high-grade ependymoma and a median survival after diagnosis of disseminated disease of 7 months (Calvo et al. 1983). The use of SRS in the setting of disseminated recurrence has not been well studied though a case report of two pediatric patients suggests that SRS may be an effect treatment in this challenging setting. Two children with nodular recurrent disease after initial therapy were treated with SRS to these lesions and remained alive without progression of disease at over 21 months after treatment (Endo et al. 2004).
28.4.1.3 Low-Grade Glioma
Low-grade gliomas demonstrate varying aggressiveness in the pediatric population.
Given that the extent of resection and presence of residual disease after surgery correlates with outcome, there has been interest in using SRS to ablate residual disease (Kortmann et al. 2003), both in the adult (Heppner et al. 2005) and pediatric population (Wang et al. 2006). There is a fairly extensive body of literature regarding the use of stereotactic radiosurgery in patients with pilocytic astrocytoma. In this setting, SRS has been shown to be associated with better progression-free survival (PFS) and complete tumor response as compared with chemotherapy or standard fractionated external beam radiation therapy (Boethius et al. 2002). In a report of 49 patients treated with SRS for pilocytic astrocytomas, tumor control was achieved in 33 of 49 patients (67%), and in 85% of patients with solid, circumscribed tumors. Two patients had temporary acute side effects attributed to radiation, but no permanent complications occurred (Hadjipanayis et al. 2002). Other studies have shown similar promising results (Hallemeier et al. 2012).
In the pediatric setting, Kano et al. report their experience with stereotactic radiosurgery for pilocytic astrocytomas in 50 children, with median age of 10.5 years (Kano et al. 2009). Median margin dose was 14.5 Gy. Progression-free survival after SRS including tumor growth and cyst enlargement was 70.8% at 5 years, and best response was seen in patients with small volume residual solid tumors (Kano et al. 2009). Similar results were found in long-term follow-up of patients treated with GKS for low-grade astrocytoma, including a report of 51 patients, 12 with grade I astrocytomas and grade II astrocytomas. The patients with grade I astrocytoma were primarily pediatric, with mean age of 9.8 years and had a control rate of 91.7%. Radiation-induced edema occurred in 18 patients (16 of whom had grade II disease), cyst formation/enlargement in 5 patients, and transient tumor enlargement in 3 patients, all of whom had grade II disease (Kida et al. 2000). Somaza et al. found similar results in early outcomes after SRS for growing pilocytic astrocytomas in childhood (Somaza et al. 1996).
Among studies including a wider variety of low-grade gliomas, Weintraub et al. reported the use of GKS in the treatment of 24 pediatric patients with low-grade glioma, all of whom had tumors that were unresectable or showed residual disease or recurrence after surgery. At a median imaging follow-up of 74 months and median clinical follow-up of 144 months, 75% of patients had a decrease in tumor size of at least 50%, and complete tumor resolution occurred in 21%. Eighty-three percent of patients had progression-free survival extending to the date of last follow-up. Pretreatment tumor size was the only significant predictor of tumor progression-free survival. The authors conclude that GKS can provide good control of residual or recurrent gliomas in pediatric patients (Weintraub et al. 2012). In the setting of retreatment, outcomes after SRS for glial neoplasms of childhood in a series of 25 children were reported, 13 with pilocytic and low-grade astrocytomas, and 11 had received previous fractionated external beam radiation therapy. At median follow-up of 21 months, 11 of these 13 children had tumor control and all were alive (Grabb et al. 1996).
A prospective trial at Dana Farber examined the use of stereotactic radiotherapy and marginal failures in a study enrolling 81 pediatric patients with low-grade gliomas, including 50 with low-grade astrocytoma, 23 with residual or recurrent craniopharyngioma, 4 with posterior fossa ependymoma, and 4 with other types. They received fractionated stereotactic radiotherapy to a mean total dose of 52.2 Gy in 1.8 Gy daily fractions. Progression-free survival was 82.5% at 5 years and 65% at 8 years. Marginal failures were not seen, supporting the use of minimal margins using stereotactic techniques. Six patients had local progression, all of which were within the tumor bed and had received full prescription dose (Marcus et al. 2005).
28.4.1.4 Medulloblastoma
Incorporation of a stereotactic radiosurgical boost into the treatment of medulloblastoma has been reported as a strategy to improve local control in patients with incomplete response to initial therapy or with well-defined recurrent disease. In a study by Patrice et al., 12 patients were treated with subtotal resection and 2 with gross total resection. SRS was delivered to the site of residual disease in 3 patients and to a well-defined site of recurrence in 11 patients. Of note, the three patients treated with a boost as part of initial treatment strategy did not experience local failure at a median follow-up of 27 months, while 6 of 11 patients treated for recurrent medulloblastoma died of progressive disease (Patrice et al. 1995). Similarly promising results in the incorporation of a boost into the initial treatment strategy were reported by Woo et al., with SRS boost to the tumor bed delivered in patients with high-risk medulloblastoma thought to be at elevated risk of local recurrence, with prescription doses of 4.5–10 Gy prescribed to the 80–100% isodose lines. All four patients treated in this manner remain alive without evidence of disease at 12–48 months after treatment (Woo et al. 1997). The only late effect was panhypopituitarism in one patient, which was attributed to the previous whole brain radiation. No patients experienced necrosis in this study.
Stereotactic radiosurgery has also been effectively used in the treatment of small volume metastatic relapsed medulloblastoma, as noted above in the Patrice report, in which 5/11 patients with recurrent disease experienced freedom from second progression after SRS. King et al. reported a series of three pediatric patients treated with Gamma Knife to recurrent medulloblastoma nodules, with single fraction treatment and doses ranging from 15 to 25 Gy. At a mean follow-up of 39 months, two patients were in complete remission, and one had stable disease. No adverse effects related to SRS were noted (King et al. 2014).
28.4.1.5 Primitive Neuroectodermal Tumor
The data for the use of SRS in the management of pediatric primitive neuroectodermal tumor (PNET) is small and primarily involves treatment of local failure or distant intracranial recurrence after multimodality therapy (Hodgson et al. 2001). In the University of Pittsburgh experience using GKS for recurrent pediatric PNETs, seven patients underwent a total of 15 GKS procedures for locally recurrent disease or distant intracranial recurrence. All patients died of disease, with median survival of 37 months from initial diagnosis, and 15 months from GKS. Two patients had early progressive disease (median survival 5 months from GKS), and five had delayed progression (median survival after progression 30 months). GKS appeared to be well tolerated in these patients, all of whom were previously treated with craniospinal irradiation and chemotherapy. However, six of seven children ultimately died of systemic disease (Flannery et al. 2009). Given the overall poor outcomes of these patients pointing to the likelihood of the presence of disseminated disease at time of relapse, others have questioned the value and appropriateness of SRS in this setting (Boop 2009).
28.4.1.6 Germ Cell Tumor
The use of SRS has also been investigated as a boost strategy in patients with intracranial germ cell tumors. Zissaidis et al. reported the results of stereotactic radiotherapy for the treatment of intracranial germ cell tumors without spinal involvement in 18 patients, with tumors measuring less than 50 mm in maximum diameter. Median dose to the tumor was 48 Gy in 1.8–2 Gy daily fractions. Five patients with multiple midline tumors received CSI followed by stereotactic boost to the tumor sites. Eight patients with germinoma in a single site received whole brain radiation followed by a stereotactic boost. Of five patients with mixed histology, two received CSI plus boost, and three received tumor only treatment. Fourteen patients are alive and free of disease at a median follow-up of 38 months. One patient developed hypothyroidism after treatment that was not present prior to radiation (Zissiadis et al. 2001). As the appropriate radiation fields in the first-line treatment setting of germinomas are currently being studied with a goal to reduce volume and dose where possible, treatment using a stereotactic approach could prove to be an important tool in this setting.
28.4.2 Benign Disease
28.4.2.1 Arteriovenous Malformation
The most common cause of intracranial hemorrhage in children is arteriovenous malformation (AVMs). Management options include observation, embolization, resection, or stereotactic radiosurgery. There is a relatively large experience reported in the use of SRS in the treatment of pediatric AVMs. In a report of the United Kingdom, experience in treating children with AVMs, 363 patients with a mean age of 12 (range 1–16 years) were treated with a total of 410 treatments, with an obliteration rate of 71.3% in patients treated once, and 62.5% in patients who were retreated, with overall obliteration rate of 82.7%. No correlation was detected between outcome and radiosurgical dose when dose was between 20 and 25 Gy, suggesting that 20 Gy may be sufficient (Dinca et al. 2012).
In the University of Virginia, experience with 200 pediatric patients treated with SRS for AVMs, 49.5% achieved total angiographic obliteration with initial GKS, and obliteration rate increased to 58.6% after second or multiple GKS, and 4.8% achieved subtotal obliteration. The hemorrhage rate was 5.4% within 2 years after GKS, and 0.8% between 2 and 5 years. Of note, six patients developed neurological deficits accompanying the radiation-induced changes, and two patients developed asymptomatic meningiomas 10 and 12 years after GKS (Yen et al. 2010). Promising results were also reported by the University of Pittsburgh group in their experience treating 135 pediatric patients with SRS for AVMs, with median margin dose of 20 Gy. Angiographic obliteration was 67% at 5 years and 72% at 10 years. Hemorrhage occurred in 6% of patients during the latency period. Permanent neurologic deficits related to radiation occurred in two patients following SRS, with cyst formation in one patient (Kano et al. 2012).
28.4.2.2 Craniopharyngioma
Craniopharyngiomas are benign tumors of epithelial origin arising from the remnants of Rathke’s pouch, and though benign, are potentially dangerous due to local growth and invasion of visual structures, hypothalamus, and pituitary. These tumors can occur in children and adults, and most reports of outcomes after SRS for craniopharyngioma include both adult and pediatric patients. Kobayashi et al. report the long-term outcomes of GKS for residual or recurrent craniopharyngiomas after microsurgical resection, with attempts at dose de-escalation with a mean tumor margin dose of 11.5 Gy, and a tumor control rate of 79.6%. Thirty-eight of 98 patients were children (Kobayashi et al. 2005). Other studies have shown similar findings, with radiosurgery for craniopharyngiomas appearing to be safe and fairly effective, with 5-year tumor control rates of 67–69% (Niranjan et al. 2010; Minniti et al. 2009; Xu et al. 2011).
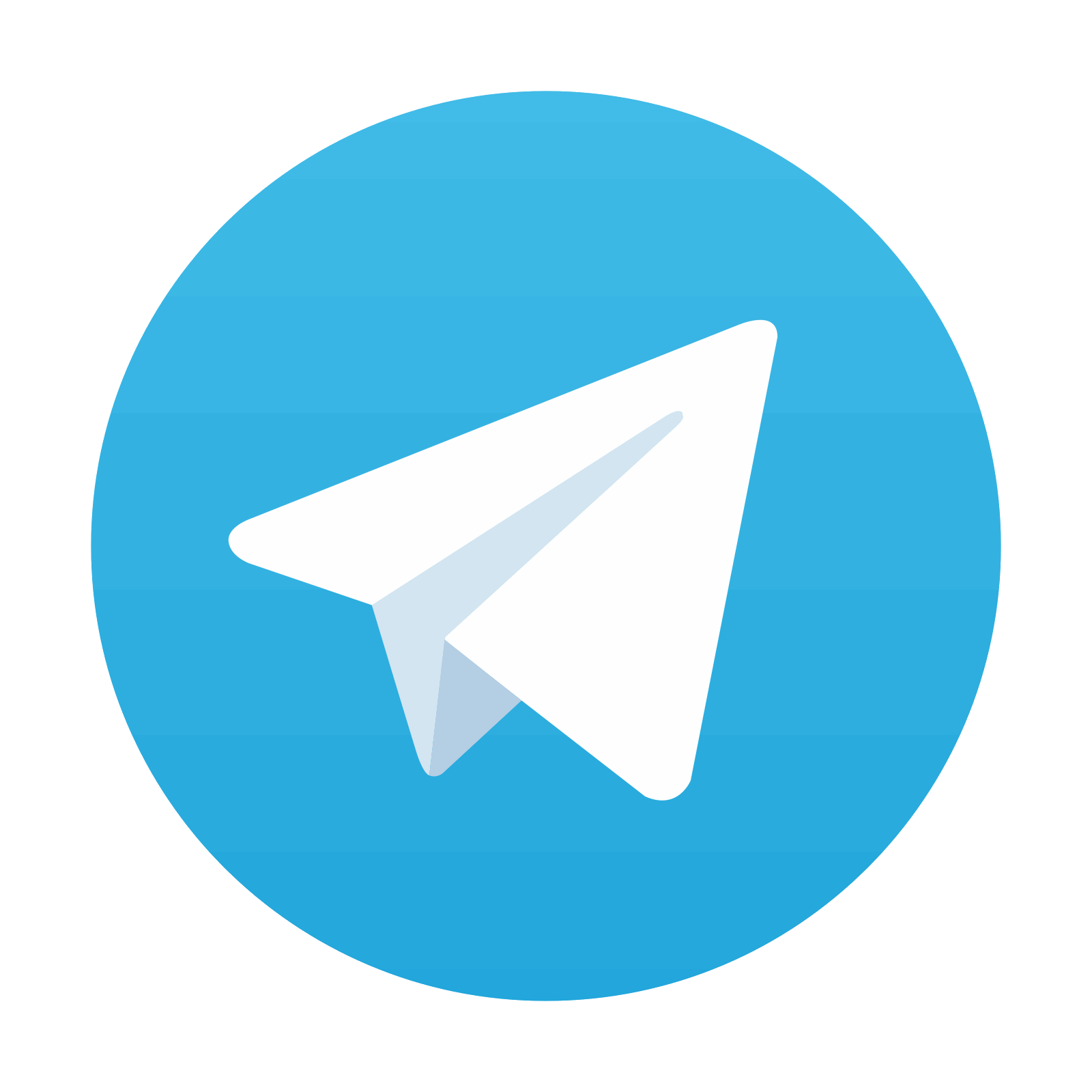
Stay updated, free articles. Join our Telegram channel

Full access? Get Clinical Tree
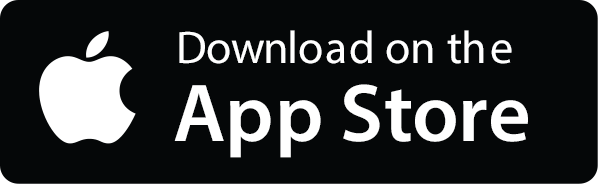
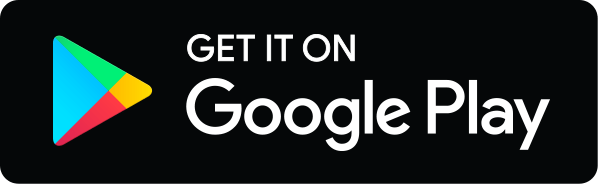
