Fig. 21.1
Semiquantitative analysis of MMP-2- and MMP-9-concentrations by determining the optical densities of a respective zymogram using peripheral blood of allogenic stem cell donors (n = 6) before and after GM-GCSF-stimulation (5d, 10 μg/kg BW). Data are given as mean ± SD, *p < 0,05 compared to baseline, Mann-Whitney-Test
Temperature
As expected by an enzyme-triggered mechanism the CD40L release is temperature-dependent. As an example CD40L concentrations in plasma samples are shown during a storage period (Fig. 21.2). The CD40L release kinetic was lowered from 7.1 ± 2.9 pg/mL/min at 37 °C to 1.9 ± 1.2 pg/mL/min at 4 °C. So the CD40L accumulation should be decelerated in stem cell products during the storage at 4 °C after the respective preparation. These expectations could be confirmed by Woods et al. (2010) who observed in products obtained by peripheral stem cell apheresis of allogenic donors only a small increase in sCD40L after a 48 h storage period.
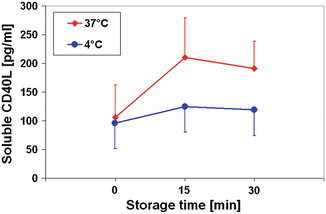
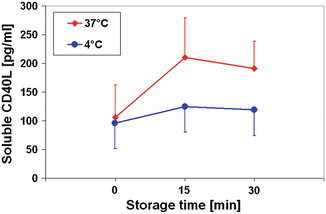
Fig. 21.2
sCD40L concentrations in platelet-rich-plasma samples of healthy blood donors (n = 8) under different storage temperatures. Data are given as mean ± SD
sCD40L Concentrations in Peripheral Blood and in Stem Cell Products
In the literature, for plasma sCD40L of the peripheral blood a range between 40–240 pg/mL (Chew et al. 2010; Wenzel et al. 2008) is described. These levels could also be found in autologous as well as allogenic stem cell donors. For example, a platelet loss (95/nL ± 57/nL at the beginning, to 75/nL ± 26/nL (during stem cell collection) and to 55/nL ± 27/nL at the end of the procedure (p < 0.05, Student’s t-test)) during autologous stem cell apheresis (n = 6) was accompanied by a significant lowering of sCD40L concentrations in peripheral blood samples from 241 pg/mL ± 137 pg/mL at the beginning, to 192 pg/mL ± 91 pg/mL (during stem cell collection) and to 124 pg/mL ± 73 pg/mL at the end of the procedure (p < 0.05, Student’s t-test, correlation coefficient r = 0.9 corresponding to the platelet count) (Fig. 21.3a). However, in the case of platelet counts remaining in a physiological range instead of the apheresis procedure – especially in allogenic stem cell donors – sCD40L levels are also not altered.
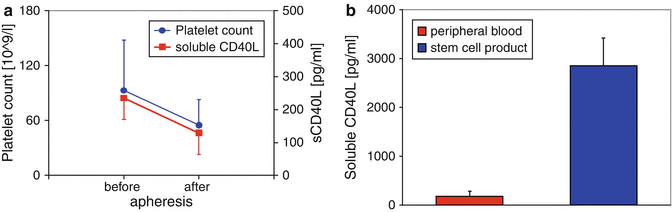
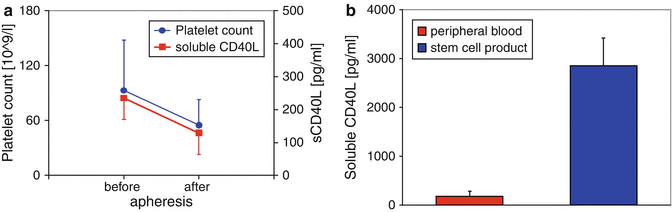
Fig. 21.3
(a) Platelet count and sCD40L concentrations in peripheral blood from autologous donors (n = 6) during stem cell apheresis. (b) Soluble CD40 Ligand (sCD40L) concentrations in plasma samples (peripheral blood) and in stem cell products from autologous donors (n = 5). Data are given as mean ± SD
In apheresis stem cell units, the platelet count showed normally pathophysiological levels and ranged from about 900/nL up to 3,000/nL. In parallel, sCD40L concentrations were elevated substantially above peripheral blood levels to a range from about 2,000 pg/mL up to 4,000 pg/mL. For example a comparison between sCD40L concentrations in the peripheral blood and in the respective stem cell product of patients (n = 6) undergoing peripheral stem cell apheresis is given (Fig. 21.3b). The sCD40L concentrations were elevated substantially above peripheral blood levels to 2,756 pg/mL ± 387 pg/mL (range: 2,189–3,641 pg/mL; p < 0.05 compared to peripheral blood) (Wenzel et al. 2011b).
In stem cell units collected from bone marrow, the sCD40L concentrations reached also a pathophysiological range from about 600 pg/mL up to 1,600 pg/mL in comparison to the peripheral blood, but remained in comparison to the apheresis products at lower levels. This may be due to the fact that the platelet count in the bone marrow showed also a reduced range from 80 to 160/nL.
In conclusion, with these facts the behaviour of soluble CD40L in stem cell concentrates could be interpreted: In line with the observations on sCD40L release in platelet concentrates, similarly a sCD40L accumulation in stem cell products could be observed, especially in the platelet-rich products prepared by apheresis. In support of the observations of Woods et al. (2010), our data showed a slow release of soluble CD40L at 4 °C. This may be attributable to reduced activity of the enzymes (MMP-2, MMP-9) responsible for sCD40L generation under these conditions. Thus the main amount of sCD40L that accumulates may be produced during the time interval of stem cell sampling and processing at room temperature before storing the stem cell unit at 4 °C.
At least Khan et al. (2006) demonstrated in an experimental work that sCD40L levels could be indirectly involved in the development of adverse transfusion reactions. But due to a lack of sufficient data regarding the pathophysiological sCD40L levels in the stem cell products under clinical routine use one may currently only speculate on effects of sCD40L influencing directly the haematopoietic stem cells in the product itself or triggering adverse transfusion reactions.
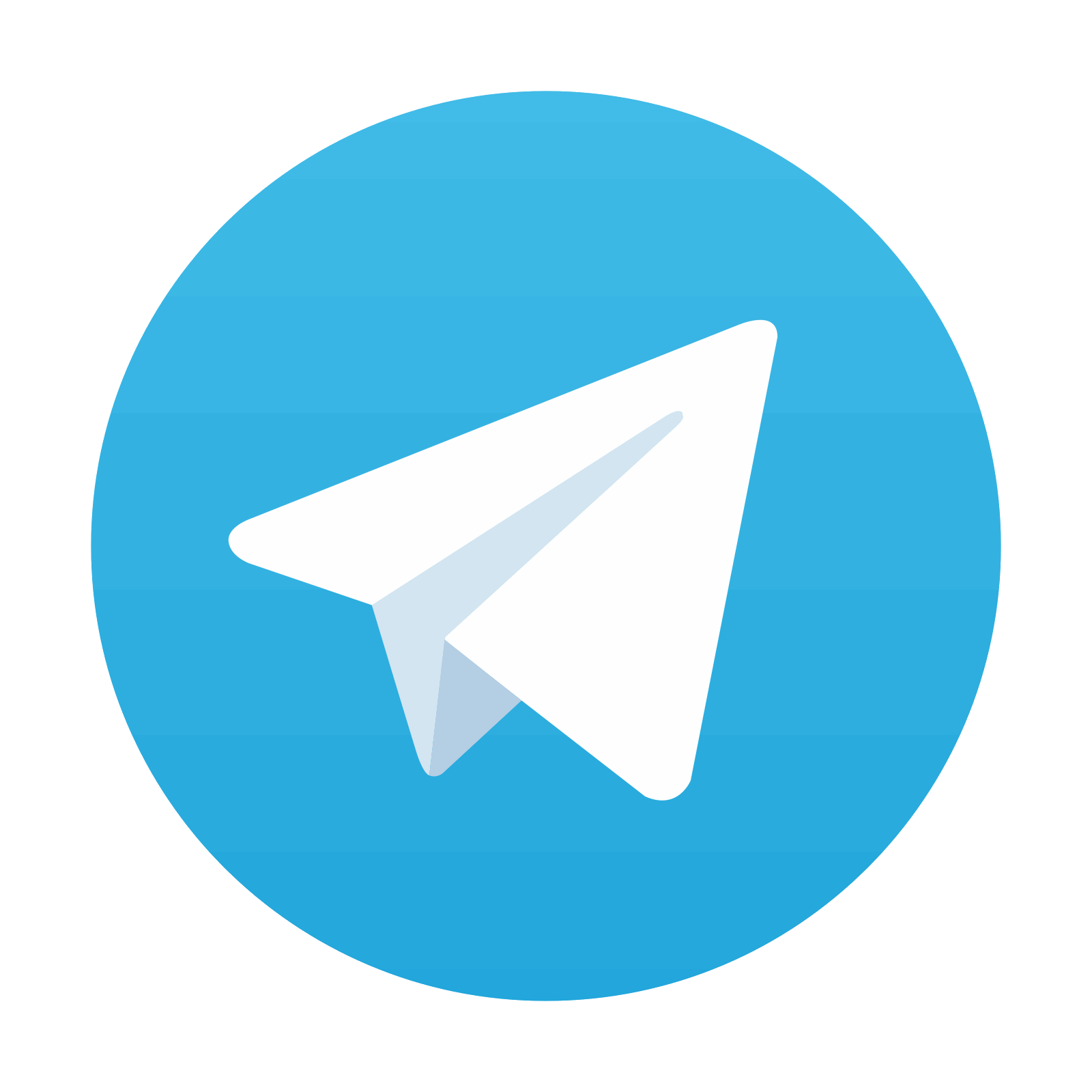
Stay updated, free articles. Join our Telegram channel

Full access? Get Clinical Tree
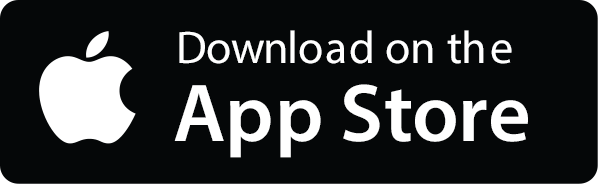
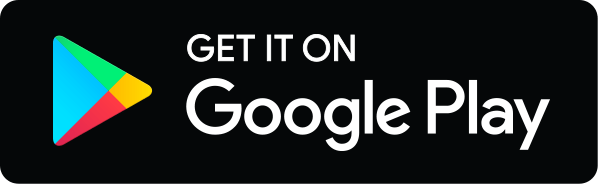