(1)
Institut Bergonié, Bordeaux, France
Abstract
Oxygen is, first of all, the combustive agent required for life and the provider of energy required by all heterotrophic organisms, those which cannot directly use solar energy as chlorophyllous plants do. Oxygen supply must be finely tuned, because insufficiency is as damaging as excess: hypoxia endangers cellular life through lack of energy supply, and reactive oxygen species (ROS) also endangers life because of their toxicity. In order to inform the organism of hypoxia or oxidative stress, signalling pathways are implemented and are studied in this chapter. In relation to oxidative stress, a special oxidised form of nitrogen, nitric oxide, is also a true intracellular messenger with multiple effects and will also be studied here.
The tumour cell is exquisitely sensitive to the effects of hypoxia and oxidative stress; it reacts to hypoxia by stimulating tumour vascularisation (angiogenesis), oxygen-independent energy supply and protein synthesis downregulation; it reacts to oxidative stress by activating signalling pathways still not completely deciphered. Reactive oxygen species appear as a double-edged sword, involved in carcinogenesis but perhaps useful to combat cancers.
16.1 Hypoxia
The main signalling pathway involved in hypoxia response depends on the activation of a transcription factor, HIF (hypoxia–inducible factor), which induces the expression of a large set of genes aimed at remedying this situation. The available oxygen amount is by itself the signal enabling HIF activation; oxygen thus appears as a signalling molecule, a messenger inducing the generation of appropriate responses.
16.1.1 Activation and Role of HIF
HIF is a heterodimeric complex consisting of an oxygen-sensitive α subunit and an oxygen-insensitive β subunit. There exist three distinct α and two β subunits. In the presence of adequate oxygen concentration, several amino acids of the α subunit undergo post-translational hydroxylation, which drives HIFα to ubiquitinylation and proteasomal destruction (Annex C). A decrease in the availability of oxygen induces a decrease of this hydroxylation and a relocalisation of the α subunit to the nucleus; in the nucleus, the α subunit can be associated to the β subunit and the complex can stimulate the transcription of target genes (Fig. 16.1). There are many signalling pathways which activate HIF transcription, especially the proliferation pathways opened by MAP kinases and PI3 kinase (Chaps. 2 and 3). Several transcription factors, such as MYC or NFκB, thus activate the synthesis of HIF mRNAs.
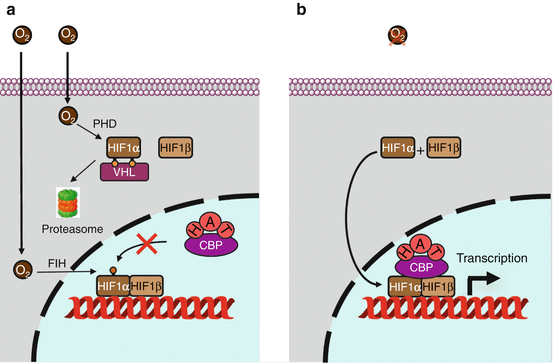
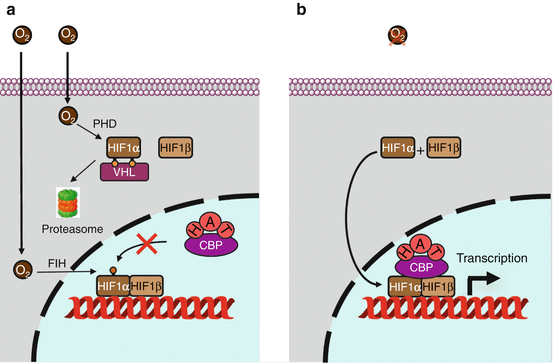
Fig. 16.1
Hypoxia signalling. (a) In the presence of oxygen, the transcription factor HIF1α is hydroxylated in the cytoplasm on two proline residues; this enables its recognition by the protein VHL, which is part of an E3 ubiquitin ligase complex, leading to proteasomal degradation. In addition, HIF1α is hydroxylated in the nucleus as an asparagine residue, which prevents its recognition by the transcription activator CBP (CREB–binding protein) that enables histone acetylation. (b) In the absence of oxygen, these hydroxylations cannot take place; HIF1α is stabilised and migrates to the nucleus, recognises its partner HIF1β and initiates transcription of target genes via the binding of the transcription activator CBP
The α subunits contain a domain called ODDD (oxygen–dependent degradation domain), with two proline residues that can be hydroxylated by prolyl 4-hydroxylases (PHD, genes EGLN) and a C-terminal domain containing an asparagine residue that can be hydroxylated in the nucleus by asparagine hydroxylase, an enzyme also known as FIH (factor inhibiting HIF, gene HIF1AN). These enzymes are non-haem dioxygenases, which use molecular oxygen, α-ketoglutarate and Fe2+ as a cofactor. Their activity is decreased when their substrate O2 is not abundant enough (hypoxia). The hydroxylated proline residues are recognised by the VHL (von Hippel–Lindau) protein, which belongs to an E3 ubiquitin ligase complex called VBC (VHL–elongin B–elongin C), which enables HIF1α to be directed to the proteasome.
In the nucleus, asparagine hydroxylation prevents HIFα binding to a coactivator of transcription, CBP (CREB–binding protein), which acts as a histone acetyltransferase for chromatin relaxation (Annex B). In the nucleus, the HIFα and HIFβ subunits are combined through their N-terminal domains and bind DNA, via a HLH (helix–loop–helix, Annex B) motif, on a hypoxia-responsive element (HRE) belonging to the promoter of the target genes. In the C-terminal domain lays the transactivation domain, TAD, divided, for HIF1α and HIF2α, into a hydroxylation-independent domain (N-TAD) and a domain inhibited by the FIH-mediated hydroxylation of an asparagine residue, C-TAD. The HIF3α subunit could rather play a negative dominant role on the transcription induced by the other HIFα subunits. The main target genes of HIF proteins are those encoding the VEGFs (vascular endothelial growth factors), especially VEGFA (Chap. 1). HIFs appear, therefore, as fundamental regulators of angiogenesis. Several dozens of other target genes are under the dependence of HIFs, involved especially in energy metabolism, erythropoiesis, vasodilatation and autophagy: for all these processes, oxygen availability is a crucial limiting factor.
16.1.2 Consequences of Hypoxia on Tumour Angiogenesis
Hypoxia constitutes the more important angiogenesis-promoting signal. Angiogenesis is indispensable to tumour growth beyond some millimetres in diameter, because only vessels can bring to the tumour the required nutriments and oxygen. In the absence of vascularisation, the deep tumour regions do not benefit of this supply and become rapidly necrotic, which limits tumour growth. Neo-angiogenesis is one of the major oncogenic processes and its targeting, which had been suggested 40 years ago as susceptible to bring original anticancer weapons, has eventually allowed the development of therapeutic approaches.
HIF overexpression is commonly observed in human cancers, sometimes in association with poor prognosis. Whereas there are no known activating mutations of the genes encoding the various HIF subunits, a loss-of-function mutation frequently occurs in the VHL gene, especially in renal cancers in which it appears recurrent. In the absence of this E3 ubiquitin ligase activity, the stability of the HIFα subunits is increased and their effects on tumour growth are increased. Antiangiogenic agents such as bevacizumab, an anti-VEGFA monoclonal antibody (Chap. 1), have brought proof of activity in this type of cancer as in several others.
16.1.3 Consequences of Hypoxia on Tumour Energy Metabolism
Among the target genes of HIFs, several genes are involved in glucose transport and anaerobic metabolism. In order to provide ATP, glucose can be metabolised according either to the mitochondrial pathway of oxidative phosphorylation, which requires oxygen, or to the cytosolic anaerobic glycolysis pathway. This pathway is less energetically profitable (two molecules of ATP are formed per molecule of glucose instead of 38), but it can present an utmost interest for hypoxic cells. By favouring glucose uptake and commitment to glycolysis, HIFs enable hypoxic cell survival. It has been known for a long time that tumour cells have a high rate of anaerobic glycolysis, even in normoxic conditions (this is the Warburg effect); this may represent a preadaptation to the risk of hypoxia, which may have been genetically selected during repetitive hypoxia events followed by re-oxygenation.
Whereas there are presently no ways to target tumour cells by agents that would selectively kill the cells using anaerobic glycolysis rather than oxidative phosphorylation, this peculiarity of tumour cells is utilised by an imaging technique, positron-emission tomography: 2-deoxy-2-18 F-glucose, a non-metabolised glucose analogue, serves as a glucose substitute to detect tumour cells.
16.1.4 Other Consequences of Hypoxia
Among the various hypoxia-regulated genes is DDIT4 (DNA damage–inducible transcript 4), which encodes REDD1/RTP801 (regulated in development and DNA damage response). This protein activates the complex TSC1–TSC2, an inhibitor of mTOR activation by the small G-protein called RHEB (Chap. 3); mTOR is involved in protein synthesis, lipogenesis, cell growth and autophagy. Hypoxia appears therefore as a way to reduce protein synthesis and to protect cell survival by autophagy.
Other potential targets of HIFs are the genes involved in apoptosis triggering (Chap. 18), via the opening of the transition pore that enables the efflux of cytochrome c from the mitochondria: proteins of the BNIP (BCL2–interacting protein) family are induced by HIFs. It seems that apoptosis induction can be operated when the hypoxic cell can no longer envisage survival.
Finally, many proteins playing a fundamental role in cell adhesion and migration are also transcriptionally induced by HIFs: vimentin (VIM), fibronectin (FN), keratins (KRT), matrix metalloproteinases (MMPs), cathepsins (CTSs), etc. Indirectly, E-cadherin (CDH1, Chaps. 7 and 11), which plays a major role in inhibiting epithelial-to-mesenchymal transition, is repressed during HIF activation. Hypoxia is, therefore, heavily implicated in metastatic processes.
16.1.5 Pharmacological Targeting of Hypoxia
Since tumour cells generally live and grow in a hypoxic environment, it would be interesting to develop drugs which would selectively target hypoxia. An old method for targeting hypoxic cells is the use of bioreductive drugs, which are activated by hypoxia by monoelectronic reduction catalysed by DT-diaphorase (NQO1) or cytochrome P450 reductase (POR). Mitomycin C is a good example of such drugs. Another approach is the use of nitroimidazoles, which have a radiosensibilising effect at the level of hypoxic regions of tumours; after reduction, these compounds stabilise DNA lesions. Misonidazole is used in positron emission tomography to visualise hypoxic tumour regions.
Upstream HIF1α, hypoxic cells targeting may consist in mTOR inhibition, which would induce a decrease in HIF1α expression (Chap. 3). Rapalogs and mTOR serine/threonine kinase inhibitors can be considered as antiangiogenic treatments and are especially active in renal cancers for which VHL mutations is an oncogenic driver. Targeting HIF1α itself is a difficult challenge. Indirect approaches with heat shock proteins (HSPs) inhibitors, such as geldanamycin, are considered. Direct approaches are represented by antisense oligodeoxynucleotides or by small molecules or monoclonal antibodies targeting HIF1α-induced proteins, such as carbonic anhydrase IX (CA9) or the glucose transporter GLUT1.
16.2 Oxidative Stress
16.2.1 Generation of Reactive Oxygen Species (ROS)
ROS are endogenously produced from molecular oxygen in several organelles, especially mitochondria, which is the main place where oxygen is utilised. ROS is the generic name for three chemical entities: the superoxide radical O2 – ●, the hydroxyl radical OH● and hydrogen peroxide H2O2. Superoxide ions are formed by reduction of molecular oxygen during a reaction catalysed by several enzymes, especially the oxidoreductases of the mitochondrial electron transport chain and membrane NADPH oxidases (NOX):
Superoxide ions are detoxified by superoxide dismutases (SOD), Cu2+-Zn2+−SOD in the intermembrane mitochondrial fluid and Mn2+−SOD in the mitochondrial matrix. The dismutation reaction generates hydrogen peroxide as follows:

