Fig. 4.1
Pathologic mechanisms of acute chest syndrome. (From: Gladwin MT and Vichinsky E. Pulmonary complications of sickle cell disease. New England Journal of Medicine.2008;359(21):2254–65. [PMID:19020327]). The acute chest syndrome in sickle cell disease is a lung injury syndrome known to be initiated by three major mechanisms, including infection, bone marrow and/or fat embolization, and direct pulmonary microvascular infarction due to red blood cell sequestration. The mechanisms lead to vaso-occlusion by sickle cells with resulting infarction and lung injury. Ventilation–perfusion mismatch and hypoxemia due to lung injury leads to increased deoxygenation and polymerization of hemoglobin S, and erythrocyte vaso-occlusion. This pathologic mechanism promotes bone marrow infarction and pulmonary vaso-occlusion. NO nitric oxide; VCAM-1 vascular cell adhesion molecule 1
Bone marrow ischemia and necrosis are characteristic of vaso-occlusion in SCD and can lead to the release of fat and bone marrow into the circulation. ACS due to pulmonary fat and bone marrow embolizationhas a distinct and severe clinical course, associated with a high prevalence of bone and chest pain, neurologic symptoms, and decreased time to recovery [29, 32], and may be involved in up to 77 % of ACS episodes in adults [33]. Fat embolization can be diagnosed by the presence of lipid-laden macrophages in bronchoalveolar lavage fluid (BALF) from ACS patients [32–34]. However, due to the requirement of bronchoscopy, this method is not routinely performed and the true incidence of embolization is unknown. Since chest and rib pain are common manifestations of SCD, non-sickle pulmonary conditions such as fat embolism can be difficult to diagnose.
Direct pulmonary vaso-occlusion and infarction due to adhesion of sickled RBCs also contributes to ACS in SCD, though the exact incidence or primary causative nature of these events is unknown. A study in 144 cases of ACS to evaluate pulmonary artery thrombosis by CT–pulmonary angiography found a 17 % prevalence of pulmonary thrombosis without associated peripheral venous thrombosis [35]. These events occurred in patients with higher platelet counts and lower hemolytic rates during ACS, suggestive of in situ thrombosis. It is important to note that other causes of ACS, including infection and fat or bone emboli, as well as hypoxemia, may contribute to the development of pulmonary thrombosis in ACS. Prophylactic therapy for venous thromboembolism with low molecular weight or unfractionated heparin is therefore commonly used in adults with ACS, unless otherwise contraindicated.
In addition, severe acute hemolysis has been identified as a predictor of sudden death due to ACS [36]. The role of hemolysis-derived plasma-free Hb and its byproducts, including hemin (the oxidized prosthetic moiety of Hb), have been proposed as a novel inflammatory mechanism in ACS [37], and this may directly contribute to lung injury in the context of vaso-occlusive crisis. In support of the role of hemolysis in ACS, genetic variants in inducible hemeoxygenase 1 (HO-1), the rate-limiting enzyme in heme catabolism, are associated with ACS incidence in children [38].
Other pulmonary complications of SCD can be modulated by ACS, including pulmonary hypertension [39]. Pulmonary pressures can rise during episodes of ACS, leading to the development of acute right heart failure and increased mortality following the ACS event [17]. Therefore, prevention of ACS may reduce mortality in SCD patients with PH [40].
Epidemiology and Clinical Presentation
ACS is the second most common cause of hospitalization in SCD, second only to vaso-occlusive pain crisis, and is a leading cause of admission to an intensive care unit and premature death [2, 27]. Ten to twenty percent of patients admitted with acute vaso-occlusive pain crisis develop ACS in the first 3 days of hospitalization, and the mean duration of hospitalization for patients with ACS is 10.5 days [6]. While most cases are self-limited, some progress rapidly to acute respiratory failure with high rates of morbidity and mortality [41]. Overall mortality is 3 % in all ACS patients and 9 % in adults [6]. ACS therefore requires rapid management to prevent clinical decompensation and death. Recent increased awareness, the chronic use of hydroxyurea, and the aggressive use of early transfusion therapy, collectively, have led to a decrease in ACS-related mortality [42]. For example, a multicenter trial of inhaled NO for vaso-occlusive crisis found that only 10 % of patients in the placebo and treatment arm developed ACS and none required mechanical ventilation or died [42].
A natural history study by the CSSCD in both adults and children found that 29 % of all SCD patients will have at least one episode of ACS, and approximately half of those patients will have more than one ACS episode [28]. In patients with ACS, up to 78 % of episodes are associated with vaso-occlusive pain crisis, and the majority of patients also have chest pain, cough, and fever [33, 36]. The incidence of ACS in SCD is dependent on the subtype present. ACS episodes can affect patients with any type of SCD, but is most prevalent in individuals with HbSS (12.8 per 100 patient-years) compared to those with HbS-β0-thalassemia (9.4 per 100 patient-years), HbSC (5.2 per 100 patient-years), or HbS-β+-thalassemia (3.9 per 100 patient-years) [28]. The presence of α-thalassemia does not alter the ACS incidence rate in HbSS patients [28].
There are significant differences in the incidence and clinical presentation of ACS between adults and children with SCD, likely reflecting the differing etiologies in these age groups (Table 4.1). The incidence of ACS is higher in children (2–4 years of age) than adults (>20 years of age) with HbSS, with 25.3 events vs. 8.8 events per 100 patient-years, respectively [28, 36]. The course of disease tends to be milder in children, with higher rates of infectious causes. Adult cases of ACS are more severe, associated with pain, and have higher rates of mortality, with a higher incidence of pulmonary fat and bone marrow emboli [6, 29]. Adults with greater ACS incidence have a higher rate of all-cause mortality than those with low ACS incidence, and this increased rate of mortality may contribute to the decline in ACS incidence with age [28]. Despite these important differences between age groups, the clinical management is similar in both children and adults.
Table 4.1
Characteristics of patients with acute chest syndromea
Characteristic | All patients (N = 537 l | Age at first episode of acute chest syndrome | P valueb | ||
---|---|---|---|---|---|
0–9 Years (N = 264) | 10–19 Years (N = 145) | ≥20 Years (N = 128) | |||
Sex (%) | |||||
Male | 58 | 63 | 57 | 48 | 0.02 |
Female | 42 | 38 | 43 | 53 | |
Hemoglobinopathy (%) | |||||
SS | 82 | 83 | 81 | 83 | 0.94 |
Other | 18 | 17 | 19 | 17 | |
Mean age at first episode of acute chest syndrome (year) | 13.8 | 5.4 | 14.6 | 30.2 | <0.001 |
Medical history (%) | |||||
Vaso-occlusive event | 80 | 72 | 85 | 89 | <0.001 |
Transfusion | 74 | 63 | 84 | 86 | <0.001 |
Acute chest syndrome or pneumonia | 67 | 63 | 66 | 76 | 0.03 |
Prophylactic antibiotic therapy | 57 | 81 | 51 | 13 | <0.001 |
Major surgery | 40 | 26 | 48 | 61 | <0.001 |
Neurologic disease | 16 | 10 | 17 | 29 | <0.001 |
Sleep apnea | 14 | 14 | 17 | 11 | 0.41 |
Red-cell antibodies | 12 | 7 | 14 | 21 | <0.001 |
Aseptic necrosis or fracture | 12 | 5 | 11 | 26 | <0.001 |
Asthma | 11 | 14 | 9 | 5 | 0.01 |
Smoking | 10 | 0 | 4 | 36 | <0.001 |
Renal disease or urinary tract infection | 7 | 3 | 7 | 14 | <0.001 |
Chronic transfusion therapy | 5 | 5 | 5 | 7 | 0.64 |
Cardiac disease | 4 | 1 | 3 | 12 | <0.001 |
Chronic lung disease | 4 | 3 | 4 | 5 | 0.46 |
Pulmonary edema | 4 | 1 | 3 | 9 | <0.001 |
Clubbing | 2 | 1 | 0 | 5 | 0.002 |
Deep-vein thrombosis | 1 | <1 | 0 | 3 | 0.01 |
Tuberculosis | <1 | 0 | 0 | 1 | 0.20 |
Reason for current admission (%) | |||||
Acute chest syndrome | 52 | 61 | 46 | 42 | <0.001 |
Otherc | 48 | 39 | 54 | 58 | |
Symptoms at diagnosis of acute chest syndrome (%) | |||||
Fever | 80 | 86 | 78 | 70 | <0.001 |
Cough | 62 | 69 | 58 | 54 | 0 006 |
Chest pain | 44 | 27 | 67 | 55 | <0.001 |
Tachypnea | 45 | 47 | 47 | 39 | 0.27 |
Shortness of breath | 41 | 31 | 44 | 58 | <0.001 |
Pain in arms and legs | 37 | 22 | 44 | 59 | <0.001 |
Abdominal pain | 35 | 38 | 36 | 29 | 0.24 |
Rib or sternal pain | 21 | 14 | 26 | 30 | <0.001 |
Reactive airway disease | 13 | 17 | 12 | 6 | 0.01 |
Neurologic dysfunction | 4 | 3 | 3 | 7 | 0.11 |
Cyanosis | 2 | 1 | 3 | 3 | 0.24 |
Heart failure | 1 | 2 | 1 | 1 | 0.49 |
Numerous risk factors are associated with increased frequency of ACS. In children, asthma has been identified as an important modifiable risk factor. A CSSCD study in 291 African American children with HbSS followed beyond age 5 years found that asthma was significantly associated with more frequent ACS episodes (0.39 vs. 0.20 events per patient-year) and painful episodes (1.39 vs. 0.47 events per patient-year) [43]. Other clinical events that increase the risk of ACS include major surgical procedures, hypoxemia, a vascular necrosis of the hips, acute rib infarcts, pregnancy, use of narcotics, acute anemic events (aplastic crises), and previous pulmonary events [28, 36]. Active cigarette smoking or environmental smoke exposure are associated with more than twice the rate of ACS episodes, though no dose effect has been determined [44]. Significantly higher rates of ACS have also been reported following influenza infection [30, 31] and in winter months [36], findings most evident in children but present in all age groups.
Several laboratory parameters are associated with the development of ACS. Rates of ACS are positively correlated with non-vaso-occlusive crisis, steady-state white blood cell counts and Hb concentration and inversely correlated with fetal Hb (HbF) levels [28]. Though higher steady-state Hb levels are associated with ACS, during acute hospitalization for vaso-occlusive crisis, the development of ACS is often preceded by an abrupt drop in Hb levels (mean decrease of 0.78 g/dL from steady-state levels) and increases in markers of hemolysis, such as lactate dehydrogenase (LDH) [6, 27]. A drop in platelet count also has been shown to precede ACS, with levels <200,000 cells/mm3, and is an independent predictor of neurologic complications during hospitalization and need for mechanical ventilation [6, 45]. Understanding the epidemiology and complex etiologies of ACS in SCD, which differ among age groups, is critically important to diagnosing and treating this devastating disease manifestation.
Diagnosis
ACS is currently defined as an acute illness in SCD patients presenting with radiographic evidence of a new pulmonary infiltrate, involving at least one complete lung segment, that is accompanied by fever, chest pain, tachypnea, wheezing, or cough [6, 24–26] (Fig. 4.2a). Abdominal, limb, rib, or sternal pain is also common, along with intercostal retractions, nasal flaring, the use of accessory muscles for respiration, and decreased SpO2 (>2 % from steady-state). The clinical presentation, epidemiology, risks, and outcomes of ACS are variable, and the syndrome can often be diagnosed as pneumonia due to the number of shared features. Many of the clinical features of ACS are also age-dependent, reflecting differences in etiology among age groups, with adults having more causative fat embolization and children with a higher proportion of infectious causes and reactive airway disease.


Fig. 4.2
(a–c): Radiographic and pathologic findings in acute chest syndrome. (From: Vij R and Machado RF. Pulmonary Complications of Hemoglobinopathies. Chest. 2010;138(4):973–983. [PMID:20923801]). (a) Chest CT scan of a 27-year-old woman with multifocal bilateral consolidations due to acute chest syndrome. (b) Post mortem specimen of a patient who died during an episode of vaso-occlusive crisis and acute chest syndrome depicting bone marrow emboli within a small pulmonary artery (hematoxylin and eosin stain, original magnification 40×). (c) Lipid inclusions within alveolar macrophages obtained by sputum induction in a patient with acute chest syndrome (oil-red O stain, original magnification 40×)
The diagnosis of ACS in SCD patients requires a high index of suspicion, and there are a number of recommended diagnostic approaches. Since the development of ACS often occurs 24–72 h after the onset of severe vaso-occlusive pain symptoms, it is critical that vigilance be kept during hospitalization of SCD patients , as they may be in the prodromal stages of ACS [25]. A thorough history and physical exam is required for all patients with SCD who present clinically with suspected signs of ACS, along with assessment of vital signs including oxygen saturation. Respiratory rate, pulse rate, and body temperature values have been shown to be higher in children than adults with ACS [36]. Patients with evidence of oxygen desaturation should have arterial blood gas measurements obtained to confirm the hypoxia [46], since pulse oximetry can be inaccurate in the presence of significant anemia. It is important to note that hypoventilation due to pain crisis or over-sedation can contribute to hypoxemia and exacerbation of ACS.
Chest radiography is required for all suspected ACS cases presenting with chest pain and/or fevers, though the findings may differ between adults and children. The CSSCD found that young children had more frequent infiltrates in the upper and middle lung lobes, while adults had more frequent lower and multiple lobe involvement and pleural effusion. However, another large, multicenter study found similar radiographic findings between adults and children with ACS [6, 36]. Radiologic signs are often absent early in development of ACS and may underestimate the severity of hypoxia [47], therefore treatment should not be delayed in the absence of significant findings and repeated chest X-rays should be obtained in suspected ACS cases.
Obtaining complete blood counts with white blood cell (WBC) differential, reticulocyte counts, and cultures of blood and sputum are also recommended for workup of suspected ACS cases. As mentioned above, an abrupt decrease in Hb concentration and platelet number often precede the development of ACS and are markers of disease severity [6, 45]. Reticulocyte counts can be used to assess for adequate bone marrow function and exclude red cell aplasia due to erythrovirus B19 infection [48]. ACS is associated with a systemic inflammatory state, with a mean peak temperature of 38.9 °C during hospitalization and a mean WBC count of 23,000 cell/mm3 [6]. Biochemical testing for C-reactive protein (CRP) can be useful in monitoring the inflammatory state of ACS patients, especially given the risk of multiorgan failure syndrome (discussed below), though the sensitivity and specificity of this test may be decreased if an infectious agent is present. Elevation in secretory phospholipase A2, a potent inflammatory mediator, occurs early in the course of ACS, before radiographic changes are detected, and has been shown to predict ACS onset [49].
Serological testing for infectious agents should also be considered in patients with ACS, both acutely and throughout illness, including detection of atypical respiratory organisms associated with ACS, such as Chlamyophila pneumoniae, Mycoplasma pneumoniae, and Legionella [48]. Pneumococcal and Legionella antigens may be detected in the urine. Testing for viral agents within sputum and nasopharyngeal samples via PCR and immunofluorescence staining, including influenza, respiratory syncytial virus, and erythrovirus B19, may also be indicated in ACS based on clinical and hematological findings [6, 48]. Identification of infective organisms can guide therapeutic treatment and/or the use of isolation facilities.
Bone marrow ischemia and necrosis lead to systemic fat and marrow embolization and contribute to a multiorgan failure syndrome with high clinical overlap and association with ACS (Fig. 4.2b). Patients with this syndrome can present with acute dysfunction of the lung, kidney, or liver, along with fever, alterations in mental status, seizures, decreased hemoglobin levels, thrombocytopenia, and coagulopathy. The similar presentation of multiorgan failure syndrome and ACS demonstrates a shared underlying etiology, with ACS predominantly affecting the lung [50]. Since the clinical manifestations of pulmonary fat embolization can be indistinguishable from other ACS causes, the diagnosis requires the identification of lipid-laden alveolar macrophages using Oil Red O staining (Fig. 4.2c). Bronchoscopy with BALF collection can be used for diagnosis, including identification of bacterial and viral agents, however this technique has been associated with an increased risk of requiring mechanical ventilation [6]. A significant correlation between results from the bronchoscopy method and induced sputum sampling of alveolar macrophages has been reported, and ACS patients with the presence of lipid-ladenalveolar macrophages in induced sputum have more extra-thoracic pain, neurological symptoms, thrombocytopenia, and elevated transaminase levels [51].
Other diagnostic procedures for ACS could include chest CT, ventilation-perfusion scanning, and angiographic imaging techniques to evaluate for pulmonary emboli, which have been shown to occur in the lungs due to both in situ vaso-occlusion of sickled erythrocytes and also secondary to pulmonary artery thrombosis in ACS [6]. Pulmonary artery filling defects are expected in these studies due to sickle cell vaso-occlusion [47, 52]. Though the extent of vascular occlusion identified by chest CT has been shown to correlate with clinical severity and degree of hypoxia in contrast to chest X-ray [47], it is not routinely recommended due to the high radiation exposure and the recurrence rate of ACS [48]. A recent study comparing the diagnostic performance and reproducibility of CT and bedside chest radiography during ACS found that CT more frequently identified lung consolidation patterns predominating in the lung bases and correlated with severity and disease course, however bedside chest radiography using CT as a reference had high sensitivity but low specificity [53].
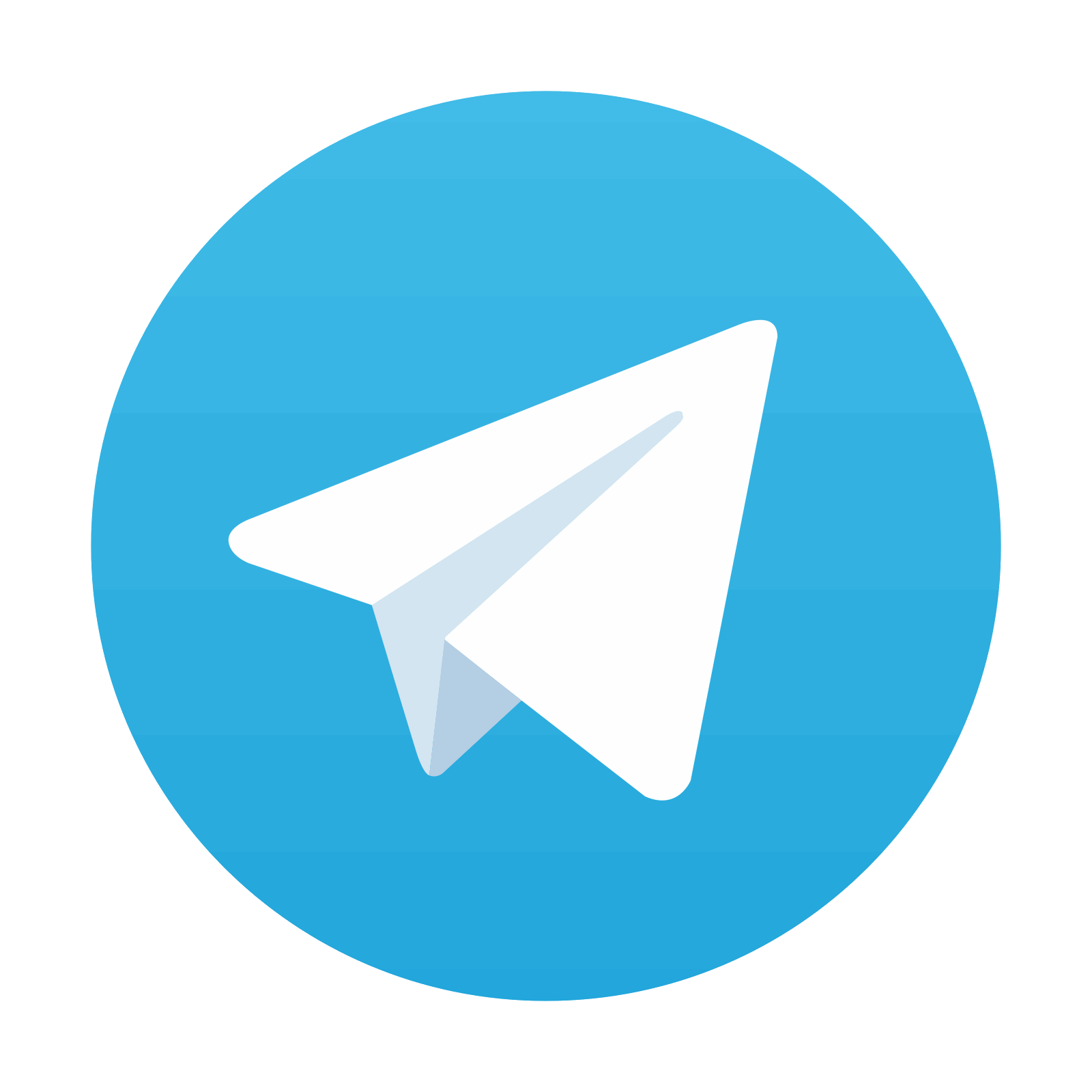
Stay updated, free articles. Join our Telegram channel

Full access? Get Clinical Tree
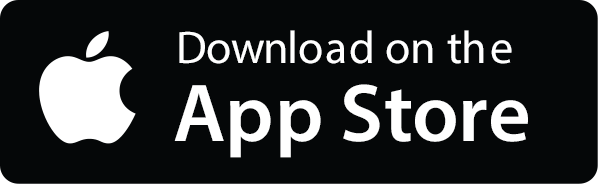
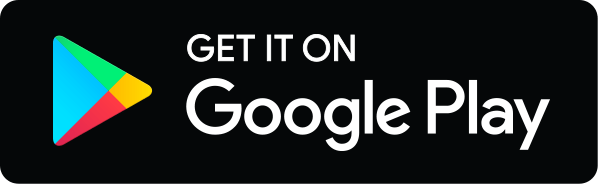