Soft-tissue sarcomas are a heterogeneous group of tumors that are capable of generating host immune responses. Historically the role of antitumor immunity was first studied in soft-tissue sarcomas. Subsequent in vitro studies, preclinical models, and clinical observations have provided ample evidence for an immunologic approach to sarcoma treatment. Initial clinical trials involving vaccines and adoptive immunotherapy have demonstrated promising results. The continued search for sarcoma tumor-associated antigens as specific targets is central to the clinical translation of effective immunotherapies.
Sarcomas are rare tumors that account for less than 1% of all adult cancers. The role of the immune system in cancer was first studied in sarcomas as immune responses to a bacterial toxin challenge were observed in metastatic bone sarcoma patients. Instances of spontaneous sarcoma regression and the observation of immune responses in human immunodeficiency virus (HIV) patients with Kaposi sarcoma undergoing antiretroviral therapy further suggest an immune-mediated antisarcoma mechanism. The development of sarcoma cell lines in preclinical animal models has allowed for further investigation of immune-related therapies, leading to clinical trials in sarcoma vaccines and cellular adoptive immunotherapy. Important to this burgeoning field, the discovery of tumor-associated antigens (TAAs) specific to sarcoma has been critical for the continued development of targeted therapies.
This review highlights the early studies of the immune system and its relationship to sarcomas, preclinical models designed to study the immunology of sarcomas, and the most current immunotherapy trials in the field. As a group, these tumors are generally thought of as aggressive, with high rates of mortality secondary to metastatic disease. As the prognosis for metastatic sarcoma is generally poor, an improved understanding of and the ability to manipulate the immune system is emerging as a viable treatment modality.
Historical perspective
In an indirect fashion, immune responses to cancer were first noted in sarcoma, dating back to 1891, by the bone sarcoma surgeon, William B. Coley. Coley conducted a series of experiments based on his clinical experience of patients with metastatic sarcoma and reports of infections causing tumor regression. Searching for better treatment options, he became aware of a sarcoma patient who had previously failed multiple surgical resections and who then developed a high fever due to an erysipelas infection followed by an unexpected regression of his large tumor burden. Therefore, Coley proposed and performed the injection of live streptococcal organisms directly into the tumors of patients with bone sarcoma in the hope of recapitulating the previously observed findings. These initial treatments proved to be fatal in two patients, though some decrease in primary tumor size was noted. Further experiments were completed using heat-inactivated streptococcal organisms, along with Serratia marcescens ; the combination was named Coley’s Toxin or Erysipelas Toxin. Over the next 20 years, Coley’s Toxin was used to treat patients who had mainly inoperable sarcomas of the bone or soft-tissue sarcomas (STS). Not only was shrinkage of the primary tumor observed, but metastatic lesions also were found to be similarly responsive. This finding led Coley to hypothesize that a systemic phenomenon was responsible. During this period, Coley’s work was criticized by a skeptical medical community because of the inconsistencies related to patient follow-up, method of administration of the toxin, and different available preparations. Coley’s daughter, however, continued this line of investigation and analyzed more than 1000 cases treated by Coley’s Toxin, with approximately 500 partial to complete responses. Subsequently a controlled study was undertaken in 1962, showing a response in 20 of 93 (21.5%) patients. By this time, the use of Coley’s Toxin had fallen out of favor for the treatment of sarcomas, due to the emergence of radiation as a standard therapy with more predictable toxicities.
Coley’s contributions to cancer immunotherapy were profound, albeit indirect, and laid the groundwork for ongoing immunologic approaches. For example, the underlying mechanism of Coley’s Toxin was found to be primarily related to bacterial endotoxin effects on the tumor-bearing host. Discovered in the 1970s, it was thought that tumor necrosis factor α (TNF-α) may have been the major host mediator of Coley’s Toxin. While TNF-α demonstrated overlapping toxicities to Coley’s Toxin, it did not produce the same degree of antitumor activity. Furthermore, the use of TNF-α to generate antitumor responses required the presence of tumor-sensitized immune cells. Similarly, Coley’s Toxin appeared to work best in patients with immunogenic tumors and in patients with preexisting immunity. As a multitude of cytokines have been discovered since that time, there are data to support interleukin-12 (IL-12) as a likely mediator of Coley’s Toxin. Unfortunately, systemic use of IL-12 is associated with prohibitive toxicities, so efforts to localize delivery of IL-12 to tumors are currently being explored.
Evidence for Immunoediting and an Immune Response in Sarcomas
Theories of the host immune system being capable of shaping the development, outgrowth, and potential responses to growing tumors has been increasingly accepted, and are applicable to sarcomas ( Table 1 ). The initial theory of immunosurveillance was formally introduced by Burnet and Thomas in 1957, stating that one of the main functions of cellular immunity was to protect from neoplastic change. In recent years, Schreiber has further championed and expanded this theory of “immunoediting” and is supported by a growing body of experimental results. As an encompassing term, immunoediting is responsible for eliminating neoplastic cells and determining the immunologic phenotypes of tumors that form. There are 3 accepted phases of immunoediting that occur as a spontaneous cancer cell arises; elimination, equilibrium, and escape. The elimination phase depicts an immune system capable of recognizing and destroying aberrant cancer cells, which is likely a frequent event. In the less common equilibrium phase, cancer cells are able to evade initial destruction and maintain a balance with the immune system under selection pressures to avoid elimination. In the escape phase, tumor growth and metastases are no longer controlled by the immune system. This final phase represents growth of cancer cells that have acquired the ability to avoid immune-system detection by a variety of means that are a compilation of adaptive changes. While it would be intuitive to assume that the process of immunoediting is governed by the adaptive arm of the immune system, there is evidence of innate immunity being capable of sculpting tumor evolution as well. Germane to this discussion, Schreiber had initially demonstrated many of the principles of immunoediting in murine models of sarcoma. Similar to these murine models, there are several lines of clinical evidence showing endogenous host immune responses to have a role in the immunoediting of sarcoma.
Immune Response | Comment | |
---|---|---|
Host response | Lymphocytic infiltration of tumor | Infiltration of primary tumor with lymphocytes associated with improved survival |
Spontaneous regression | Seen in desmoids tumors most frequently, especially in the postpartum period Case reports of spontaneous regression in other sarcoma types including osteosarcoma | |
Unplanned resections | Incomplete resection occurs at first surgery with repeat excision for positive margins yielding no further tumor and improved outcomes | |
Sarcoma-specific antigenicity | Antibody cross-reactivity in sarcoma patients | Antibodies from one sarcoma patient cross-reacts to sarcomas from different patients, with similar and dissimilar histologies |
Immunization with irradiated sarcoma cells | Injection of patients with their own irradiated sarcoma cells results in rising cytotoxic antibody titers | |
Viral | Kaposi sarcoma | In human immunodeficiency virus patients with Kaposi sarcoma (KS), caused by human herpesvirus 8 (HHV8), initiation of highly active antiretroviral therapy is associated with an increase in CD4 + counts, and partial or complete regression of KS lesions, with a decrease in HHV8 viral load |
Lymphocytic infiltration of tumors, indicating an immune response, has been associated with improved patient survival. This observation has been recently reported in patients with Ewing sarcoma, as the number of tumor-infiltrating CD8 + T lymphocytes was directly correlated with improved survival as opposed to CD8 + T lymphocytes found in the surrounding stroma. Furthermore, infiltrating cytotoxic CD8 + T lymphocytes were linked with the detection of proinflammatory cytokine attractants or chemokines expressed by the tumors, suggesting a causal mechanism. Conversely, if high concentrations of T-regulatory cells are found in the bone marrow of Ewing sarcoma patients, this has been associated with metastatic disease. These T-regulatory cells, defined by the phenotype of CD4 + CD25 hi FoxP3 + , are known inhibitors of cytotoxic CD8 + T lymphocytes and likely promote tumor escape with subsequent metastases.
Additional evidence for a host antitumor immune response includes spontaneous tumor regression. Spontaneous regression has been typically reported in tumors such as cutaneous melanoma and renal cell carcinoma. Although spontaneous regression in sarcoma is rare, it is occasionally seen in desmoid tumors or fibromatosis following hormonal changes associated with the postpartum state. There are also reports of partial spontaneous regression with lymphocyte infiltration of extraskeletal osteosarcoma, supporting a role for the immune system in this process. Of interest is that following unplanned resections of extremity soft-tissue tumors that are found to be a positive-margin STS on final pathology, re-resection yields residual disease in only 35% of patients. These findings further support the possibility of a host immune response to sarcoma. A large analysis of extremity STS patients at Memorial Sloan-Kettering Cancer Center reported by Lewis and colleagues also demonstrates a potential immune-mediated component to sarcoma outcome. A total of 685 primary extremity STS patients who underwent initial definitive resection were compared with 407 similar patients treated with a definitive reexcision following a previous nontherapeutic excision at an outside institution. Unexpectedly, the 5-year disease-free survival for the definitive versus re-resected patients was significantly different, at 70% versus 88%, respectively ( P = .0001). These survival differences could not be explained by a referral bias, which would presume that more advanced or complicated patients would be seen at a tertiary center that could provide a single definitive surgery. In fact, when analyzed according to stage, all re-resected patients trended toward an improved outcome in comparison with the definitively treated group, which was statistically significant ( P = .005) for the stage III group (>5 cm, deep, high grade) These observations extended beyond the local recurrence-free survival, as there was also an improvement in metastasis-free survival for the re-resected group, suggesting a possible systemic effect. Given these observations in extraskeletal osteosarcomas and primary extremity STS, it can be postulated that an incomplete initial excision may prime an immune response against remaining tumor cells, leading to improved residual tumor-cell elimination or equilibrium.
The loss of major histocompatibility complex I (MHC I) on sarcoma cells is seen as a means of escaping immune-system elimination. All nucleated cells express MHC I on their cell surface, which acts as a marker for recognition by the cellular arm of the immune system. Foreign antigens, such as viral proteins, can be presented on MHC I molecules and recognized by CD8 + T cells, leading to efficient killing of the infected cell. In a parallel fashion, unique tumor antigens may be expressed on MHC I molecules found on the tumor cell surface, which can lead to elimination of the tumor cell by CD8 + T cells. There is a substantial body of literature addressing tumor loss of MHC I molecule expression as a means of escaping recognition by the immune system. In a study concerning sarcomas specifically, MHC I was lost or downregulated in 46 of 74 (62%) various histologic types. Patients with osteosarcoma that expressed MHC I had a significantly better overall and event-free survival than those with MHC I–negative osteosarcoma, suggesting the importance of cellular immunologic responses to sarcoma.
Humoral or antibody immune responses to sarcomas were shown by Mitchell and Morton in a series of patients with a variety of soft-tissue and bone sarcomas. Antigenicity appeared to be common and nonspecific among all the sarcomas studied, as fluorescein-conjugated antibodies from patients with one type of sarcoma were found to cross-react in vitro against tumors with both similar and dissimilar histologies. However, an immune response specific to a particular tumor was shown to be generated in the sarcoma-bearing host, as antibodies found in the serum could be used to identify circulating or excreted sarcoma antigens in the same patient’s urine. These observations indicate that a common antigenicity may exist between sarcomas, regardless of histology, and that an immune response specific to a particular sarcoma is identifiable in individual patients.
To test this immune-response concept, Gupta and Morton collected 24-hour urine samples from sarcoma patients, both before and after surgical resection. Serum was also collected 2 weeks after resection when sarcoma antibody titers were found to be highest. The antigenic activity of urine and serum was tested using the ultra-microcomplement fixation test. Urine and serum samples from healthy volunteers did not show any reactivity against tumor cells, whereas urine and serum samples from 12 of 13 sarcoma patients reacted positively against autologous and allogeneic sarcoma serum. Reactivity of the urine to sarcoma sera disappeared after surgical ablation of the tumor mass and reappeared following clinical recurrence of the tumor in 2 patients. This study demonstrated that sarcoma-specific antigens were present in both the serum and urine of sarcoma patients, and that specific antibody titers varied with tumor volume.
Further insight into the immune response to sarcoma can be found in immunosuppressed patients with Kaposi sarcoma (KS). Human herpesvirus 8 (HHV8) or KS-associated herpesvirus (KSHV) is the known causative oncogenic virus for KS, which in general portends a poor prognosis. Clinical KS can be grouped into 4 epidemiologic forms: classic KS affecting men of eastern European Jewish background, endemic KS occurring in Central and East Africa, iatrogenic KS developing in immunosuppressed patients after organ transplant, and epidemic or AIDS-KS, a major AIDS-defining malignancy in the Western world.
In the HIV-infected population, KS is the most common neoplasm of untreated individuals. Once highly active antiretroviral therapy (HAART) was introduced, a decline in the incidence of KS was observed in the HIV-positive population. The reason for this unexplained decrease in KS incidence was hypothesized to be related to improvement in the host’s immune system, thereby allowing for an immune response against the virally induced sarcoma. To further elucidate the reason for this unexplained decrease in incidence, a prospective study was undertaken to monitor immune responses of HIV-positive patients with identified KSHV infection while receiving HAART. Following 52 weeks of HAART treatment, decreases in both HIV-1 and KSHV titers were noted, which were associated with significant increases in CD4 + T-lymphocyte counts. In a further study, 29 patients with HIV infection, 21 with KS and 8 without KS, were evaluated prospectively during HAART. Nearly half of the patients with KS had a favorable response to HAART, with a complete clinical response/remission in 6 patients and a partial response (defined as shrinkage of the primary KS) in 4 patients. Of the 20 patients with detectable KSHV viral load before the initiation of HAART, 60% had undetectable viral loads with therapy. These studies confirm that sarcoma responses can be directly related to the status of the host’s immune system.
Preclinical Data for Sarcoma Immunotherapy
A series of preclinical studies completed in the 1980s were some of the first to lay the groundwork for immunotherapy for sarcoma in humans. By this time, methylcholanthrene-induced fibrosarcoma (meth A) cell lines had been well established as a model of syngeneic transplantable sarcomas in mice. Meth A–implanted tumors grew progressively and were lethal to the host. However, when meth A tumor cells were injected jointly with a mixture of formalin-killed Propionibacterium acnes bacteria, tumors grew for 9 days and would then spontaneously reject. The tumor rejection was found to be a T-cell–mediated phenomenon, and the antitumor immunity of these cured mice could be transferred into other meth A tumor-bearing mice. To transfer this immunity, the tumor-bearing hosts had to either be lethally irradiated/restored with bone marrow or undergo immunosuppression with cyclophosphamide before receiving donor T cells from the cured mice. The transferable immunity was limited in that it was incapable of treating tumors that were established for more than 9 days. This ability to generate and transfer antitumor immunity in preclinical models is a cornerstone of current adoptive immunotherapy protocols.
In similar animal models developed at the National Cancer Institute, mouse fibrosarcomas were generated by injecting methylcholanthrene (MCA) intramuscularly, and malignant cells harvested for tissue culture. The 2 main tumor types, MCA 105 and MCA 106, were used to dissect issues of adoptive immunotherapy. Similar to the meth A sarcoma studies, adoptive transfer of spleen cells from mice immunized with MCA 105 or MCA 106 tumor cells induced complete regression of existing MCA 105 and MCA 106 fibrosarcomas in host mice, respectively. As a more stringent model, the MCA series of sarcoma tumors were used within the first 5 transplantation passages so that the tumor-associated transplantation antigens were more representative of the initial tumor, without genetic alteration. Further characterization of the effector cells generated after stimulation with MCA 105 cells showed that regression of the preestablished MCA 105 sarcoma was done in an antigen-specific fashion. These studies served as the basis for the process by which lymphocytes from sarcoma-bearing mice could be expanded and sensitized, using an in vitro sensitization procedure and expansion with interleukin-2 (IL-2). The ability to eradicate established MCA sarcoma pulmonary metastases by adoptive transfer of activated and expanded lymphocytes has been well reported.
More recent preclinical studies have used cytokines historically associated with sarcoma regression to target the tumor microenvironment and enhance endogenous immune responses. Using the MCA 205 sarcoma line, TNF-α and IL-12 delivery by a slow-release mechanism has demonstrated significant antitumor effects. The mechanism of action seems to be dependent on cytotoxic T cells and natural killer (NK) cells. Intratumoral treatment of a primary MCA 205 tumor with TNF-α and IL-12 led to growth inhibition of synchronous subcutaneous tumors or pulmonary metastases, which was abrogated by the depletion of endogenous T cells or NK cells. Furthermore, the antitumor lymphocytes generated from this approach were able to be transferred into mice bearing similar MCA 205 tumors and to mediate significant tumor regression of established pulmonary metastases. The ability of IL-12 to enhance immune responses has also been demonstrated in murine models of sarcoma vaccines. In addition, gene-transfer protocols for the delivery of IL-12 have been developed in murine sarcoma models, and show antitumor synergy with radiation. These preclinical studies demonstrate the ability to localize cytokines or deliver synergistic doses, which are normally toxic when given systemically, to create antisarcoma immune responses. Collectively these studies strongly support the clinical application of cellular immunotherapy for sarcoma, and are a basis for clinical trials.
In terms of a humoral approach, the development of monoclonal antibodies (MoAb) led to the theory that immunotherapy by passive administration of antibodies to patients with sarcomas might be a simple and effective therapy. This concept was tested through a series of preclinical studies in a murine sarcoma model. MoAb directed against Moloney sarcoma cells (MSC) were produced by fusion of spleen cells to antibody-producing cells. BALB/c mice with existing sarcomas were given 3 schedules of antibody injection; MoAb injected prior to sarcoma tumor-cell inoculation, on the same day of inoculation, and 4 days after the establishment of sarcoma tumor. MoAb injection was found to significantly prolong the life span of all mice, regardless of the injection regimen. However, MoAb given to mice with very large tumors (>10 days after implantation) did not prolong survival significantly. This model also was used to test factors affecting passive MoAb therapy. In evaluating the mechanism underlying the improved survival in MSC-bearing mice treated with MoAb, nu/nu mice, those depleted of complement, and mice that were irradiated before treatment all showed a response to MoAb treatment. This finding indicated that the MoAb therapy effect was independent of complement, B cells, or cytotoxic T-cell responses, and may indicate direct antibody-mediated cell lysis.
Clinical data for sarcoma immunotherapy
Vaccines
Vaccines directed against tumor-specific peptides, proteins, and DNA have shown varying degrees of success in the treatment of cancer. Vaccine strategies for the treatment and prevention of sarcoma recurrence are intriguing as they represent a targeted, tumor-specific, nontoxic therapy. Sarcomas are ideal vaccine targets, as tumor tissue can often be obtained in abundance for the generation of a variety of vaccines. The potential for repeated and late sarcoma recurrence, particularly in the case of retroperitoneal liposarcomas, also makes a preventive-vaccine approach attractive.
In clinical terms, vaccine treatment for sarcoma dates back to the 1960s with a whole-cell and cell-lysate sarcoma vaccine for osteogenic sarcoma. In postamputation patients, encouraging responses to cell-lysate vaccine therapy were noted, with the primary end point being a delay in the development of pulmonary metastases. Although this early study was weakened by its reliance on historical controls for comparison, it highlighted a standardized method for vaccine generation and clinical delivery. A more recent study of a vaccine derived from an autologous tumor cell line demonstrated the feasibility of establishing a short-term culture of human sarcoma cells followed by irradiation and cryopreservation for use in a subcutaneous vaccination protocol. Delayed-type hypersensitivity (DTH) reactions were monitored during the vaccination period, which consisted of weekly injections for 3 weeks followed by monthly injections for 5 months. In this trial vaccine was successfully generated from 56% of the patients, and DTH responses were noted in half of the vaccinated patients by week 3. Although no objective antitumor responses were observed, this study provided encouraging findings regarding vaccine generation, safety, and potential immune readouts in sarcoma patients.
Some novel vaccine approaches have already been investigated for tolerability and immune responses. For example, osteosarcoma overexpresses a glycoprotein designated CD55, which protects from immune complement attack. This overexpression was exploited as a potential target for a clinical trial of immune therapy in patients with metastatic osteosarcoma or those having a high risk of recurrence. Following 1 to 6 months of myelosuppressive chemotherapy, patients were vaccinated with an anti-idiotypic antibody to mimic CD55 in an attempt to generate immunity against tumor-expressed CD55. The majority of patients in this trial showed measurable T-helper cell responses as evidenced by increased T-cell proliferation and interferon-γ secretion, while no significant toxicity was noted.
Contemporary vaccine immunotherapies have used dendritic cells (DC), which act as antigen-presenting cells in vivo. DCs can sensitize T cells to tumor-specific antigens and induce cytotoxic T lymphocytes. This approach was applied in a study of pediatric patients with relapsed solid tumors, a large percentage of which were sarcomas. DCs were generated from peripheral blood samples from these patients and were pulsed with tumor-cell lysates ex vivo. Tumor-cell lysates are thought to prime DCs to present a variety of tumor-associated antigens. Intradermal injection of 1 × 10 6 or up to 1 × 10 7 tumor-lysate pulsed DCs was performed every 2 weeks for a total of 3 injections. Pediatric patients with Ewing sarcoma, osteosarcoma, and fibrosarcoma were treated, and significant tumor regression was noted in the metastatic fibrosarcoma patient. The study demonstrated that a DC vaccine protocol could be completed safely and with minimal toxicity in an outpatient setting.
As an example of targeting a specific translocation mutation found in sarcoma, a DC vaccine protocol was used in an 11-year-old patient with relapsed synovial sarcoma. DCs were generated from peripheral blood and were pulsed with a peptide from the SYT-SSX fusion protein commonly found in synovial sarcoma cells. The vaccination with sensitized DCs was well tolerated and arrested the growth of lung metastases for 4 months. In a pilot study of 52 pediatric patients with metastatic or recurrent Ewing sarcoma or alveolar rhabdomyosarcoma, patients underwent immune cell harvest, standard chemotherapy, and immune therapy involving autologous T cells and DCs pulsed with peptides derived from tumor-specific translocation break points. Toxicity was minimal and the 5-year overall survival rate was 43%, comparing favorably with results in the literature.
Use of Adjuvants
The use of adjuvants, or agents designed to enhance the activity of vaccines without any antigenic effect itself, have also been investigated. Recent developments in this area include the concept of costimulation, whereby the immune response from a vaccine can be made more effective by providing stimulation of immune-specific T cells. In vitro studies indicate that granulocyte macrophage-colony stimulating factor (GM-CSF) is a powerful immunostimulant, and that the host response to a tumor can be amplified by vaccination with tumor cells altered to express GM-CSF. In a phase I study of GM-CSF gene-transfected autologous sarcoma cells, the technique was deemed safe and feasible in a vaccine protocol. Unfortunately only a small number of patients, 4 of 17 (23.5%), had a clinically elevated level of gene expression. A comparative phase II trial of autologous tumor vaccine with either adjuvant interferon-γ or adjuvant GM-CSF for solid tumors included STS patients with advanced or metastatic disease. Both adjuvants were well tolerated. Five-year overall survival was 25%, with minimal differences between the two arms. In another vaccine study of patients with recurrent Ewing sarcoma or alveolar rhabdomyosarcoma, immature dendritic cells were pulsed with peptides derived from break-point regions of tumor-specific fusion proteins. As an adjuvant, patients received a concurrent intravenous infusion of IL-2. Toxicity was mild and was mostly related to the known adverse effects of IL-2. The peptide vaccination and IL-2 combination in this study did not alter the clinical outcomes for these patients, as only one patient showed evidence of an immunologic response.
The search for natural adjuvants to enhance vaccine responses has generated interest in heat-shock proteins (HSPs). Various forms of cellular stress, such as hypoxia, pharmacologic agents, or heat, can cause increased synthesis of HSPs, which act as intracellular chaperones to bind, assemble, and disaggregate proteins. When released extracellularly, HSPs are potent immune stimulators, as they enhance antigen processing and presentation. Although clinical trials using HSPs as adjuvants in sarcoma have yet to be realized, preclinical literature to support this approach is growing. The use of HSPs derived from tumors enhances immune responses to MCA-induced sarcomas and protects against tumor establishment in mouse models. HSPs can serve as immune stimulators or as potential targets, as their expression may be unique to certain tumor types. In a series of in vitro experiments, HSP72 was predominantly found in tumor cells in culture, specifically Ewing sarcoma and osteosarcoma cells, in comparison with normal cells from healthy human volunteers. After a nonlethal heat stress at 41.8°C and a recovery period at 37°C, HSP72 was found in these cell lines to be localized to the cell membrane rather than within the cells. The heat-shock–inducible tumor-specific HSP72 cell-surface expression was associated with an increased sensitivity to lysis mediated by NK cells stimulated by IL-2, and was not dependent on tumor cell expression of MHC I. In general, it is thought that because particular HSP expression is limited to tumor cells in vitro, the ability to induce an antitumor immune response resulting in cell death in vivo may be a possibility.
Hyperthermia has shown promise in enhancing the effects of chemotherapy and radiation therapy in the clinical treatment of sarcomas, and is being explored as an adjuvant to immunotherapies. The combination of immunotherapy and hyperthermia was investigated in a preclinical model of MCA 105 sarcomas. Mice were treated with whole-body hyperthermia alone, immunotherapy with tumor-sensitized lymphocytes, or whole-body hyperthermia and immunotherapy. A significant reduction in growth of both primary extremity sarcomas and pulmonary metastases was noted. The use of hyperthermia as an adjunct to immunotherapy for human sarcomas has yet to be fully characterized, but it may ultimately prove to be a nontoxic and effective adjuvant.
Adoptive Immunotherapy
Adoptive immunotherapy, involving the transfer of preconditioned immune reactive cells to a tumor-bearing patient, has been extensively studied. Preclinical data have demonstrated that the transfer of tumor-infiltrating lymphocytes (TIL) expanded with IL-2 into mice with pulmonary or hepatic metastases from MCA 105 sarcoma resulted in a 50% cure rate. A caveat to the transfer of TILs from human tumors, including sarcoma, is that although the culture of TILs could be established, the T lymphocytes are phenotypically heterogeneous and do not remain constant during prolonged time in culture. Considering a different approach in the pediatric population, NK cells were collected from peripheral blood of healthy adult patients and sensitized with Ewing sarcoma, rhabdomyosarcoma, neuroblastoma, and osteosarcoma cell lines. Ewing sarcoma cells appeared to be the most sensitive to the cytotoxicity of expanded activated NK cells.
These findings were recently extended to a clinical application of adoptive immunotherapy in patients with synovial cell sarcoma. Autologous T cells were transduced with T-cell receptors capable of recognizing NY-ESO-1, a cancer/testis antigen (CTA) that is overexpressed in the majority of synovial cell sarcomas. In a clinical trial using these genetically engineered T cells combined with IL-2, two-thirds of patients with synovial cell sarcoma had objective clinical responses. One patient showed a partial response that was durable for 18 months. This study showed that sarcomas might be susceptible to adoptive immunotherapy protocols and that a targeted approach could result in profound clinical responses.
Role of Other Immune-Associated Molecules
Further insight into T-cell activation and function involves an understanding of the role of cytotoxic T-lymphocyte antigen 4 (CTLA-4). Preclinical studies have shown that T cells are inhibited by CTLA-4, which is undetectable on freshly isolated T cells but is detected in significant amounts 48 hours after T-cell stimulation. As a likely feedback loop mechanism, CTLA-4 strongly inhibits T-cell proliferation as well as IL-2 secretion by T cells. The theory that the combination of a vaccine with anti–CTLA-4 antibody would enhance the T-cell response to the vaccine was tested in two mouse models: a meth A sarcoma cell line and a primary prostate tumor cell line. Results of these studies and others indicate augmentation of the immune response when anti–CTLA-4 antibody is added at the time of immunotherapy. Clinical trials involving the anti–CTLA-4 antibody, ipilimumab (Yervoy; Bristol-Myers Squibb, New York, NY), have been completed in melanoma, in which an objective response rate of 15% has been reported to the antibody alone. Concomitant administration of ipilimumab with sarcoma vaccines has not yet been studied in clinical trials, but results from melanoma studies suggest this may be relevant.
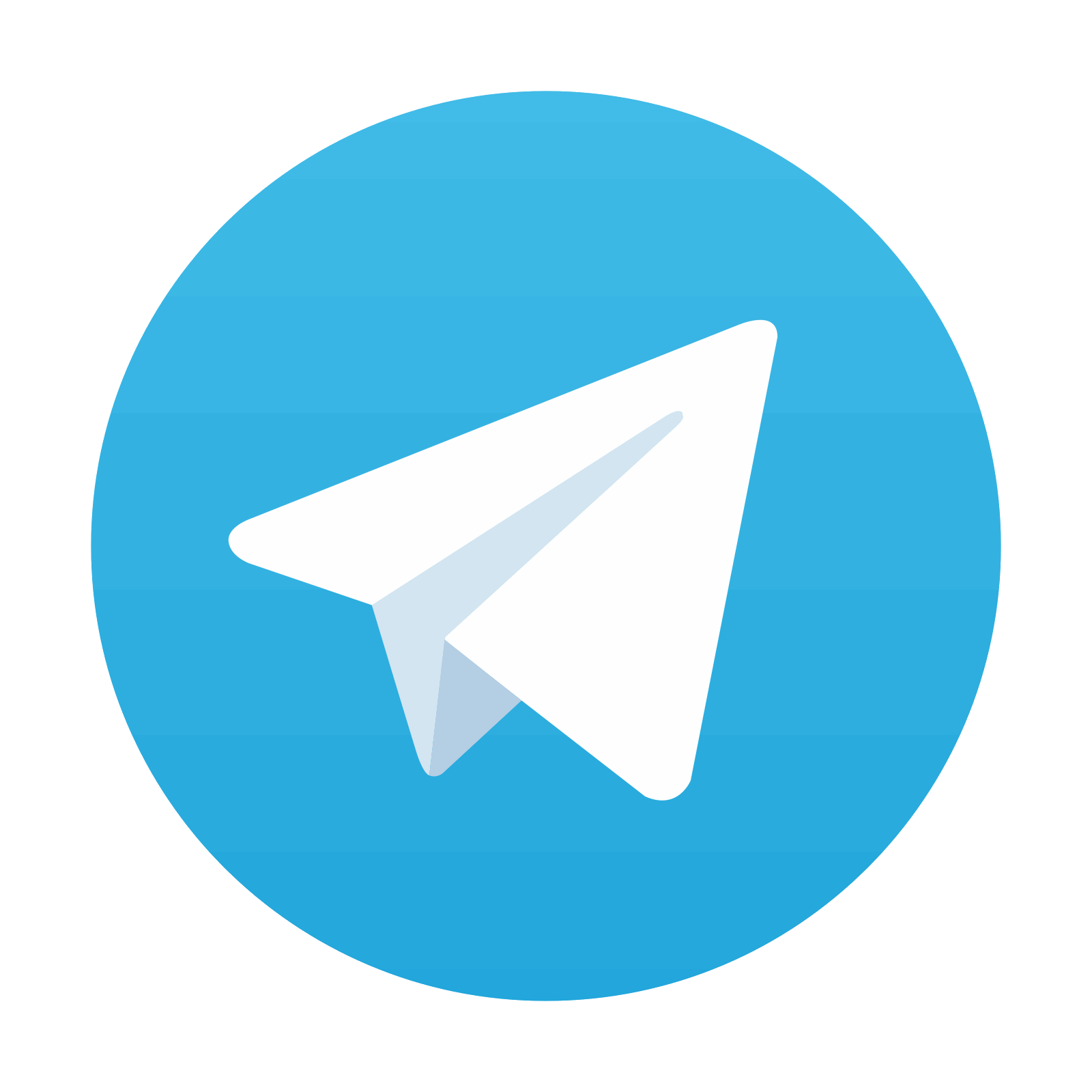
Stay updated, free articles. Join our Telegram channel

Full access? Get Clinical Tree
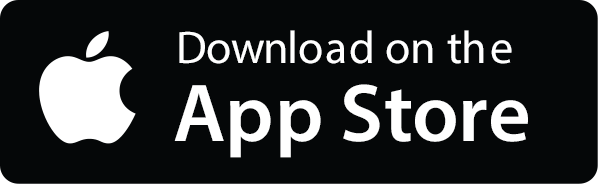
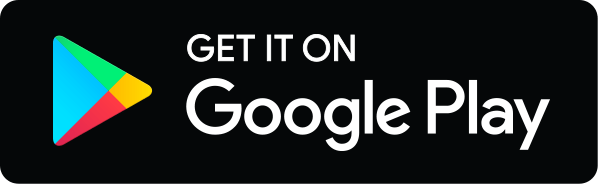