Keywords
RNA interference (RNAi), short hairpin RNA (shRNA), ABCD nanoparticle, convection-enhanced delivery (CED), O 6 -methylguanine–DNA methyltransferase (MGMT), malignant glioma, temozolomide (TMZ), genetically modified T cell therapy
Introduction
Gene therapies have been developing and evolving during the past two decades and are now being evaluated in clinical trials for the treatment of a number of diseases, including cancer. Therapeutic strategies include transduction of suicide genes, use of immune or tumor suppressor genes, as well as the application of oncolytic viruses. Recently, the focus of gene therapy research has shifted to include messenger RNA (mRNA) targeted strategies of therapy, as well as gene-based strategies. This is so because protein amino acid residue sequences, and hence three-dimensional structures, are defined by genetic coding information that is found within open reading frames (genes) located in the sense strand of double-stranded DNA (dsDNA) duplexes. This information must be transcribed into mRNA before translation and concurrent protein synthesis. Accordingly, control of mRNA levels in cells becomes another direct way to regulate cellular activities at the level of the nucleic acids, and selected mRNAs represent excellent therapeutic targets for gene therapy of diseases including cancer.
During the past few years, there has been an anomaly at the heart of gene therapy, namely that only 1 or 2% of genomic DNA is made up of gene sequences, leaving a substantial percentage of “junk DNA.” Today, we now realize that this “junk DNA” substantially codes not for mRNAs but for other shorter species of RNA known collectively as noncoding RNAs (ncRNAs). In cells, epigenetic mechanisms control the machinery of gene expression at the level of replication and transcription. Post-translational modifications of proteins (phosphorylation, glycosylation, acylation, and alkylation) diversify the behaviors and capabilities of the protein products of gene expression in vivo . This leaves the ncRNAs, which appear to have profound controlling effects in cells post-transcription of the mRNA message . In other words, ncRNAs act as regulators of mRNA message and hence of protein expression levels by controlling the life span, processing, and splicing of mRNA. In so doing, ncRNAs substantially make use of natural RNA interference (RNAi) pathways that developed originally to regulate the levels and use of mRNAs in cells or to eliminate pathogen-derived mRNAs prior to translation.
During the past few years, considerable work has been done to identify ncRNAs capable of the highest levels of specific mRNA selection and destruction, minimizing the likelihood of off-target effects (unintentional mRNA selection and destruction) and other unintended consequences such as RNA-mediated immune effects. The use of ncRNAs as therapeutic agents active against disease at the RNA level may be described as RNAi therapeutics, a new branch of gene therapy . In this chapter, we summarize RNAi therapeutic approaches for the treatment of solid tumors, culminating with reference to our O 6 -methylguanine–DNA methyltransferase (MGMT) RNAi therapeutic strategy for malignant glioma . Subsequently, we describe the perspectives toward therapy improved by using a combination of genetically modified T cell therapy and RNAi therapeutics .
Origins of RNAi Therapeutics
Antisense RNA
Given the central importance of mRNA as an intermediate carrier of genetic information transcribed from the sense strand of dsDNA, for many years there has been a historic mechanistic and pharmaceutical interest in targeting mRNAs for destruction. These approaches relied on two aspects of nucleic structure; namely (1) the Watson–Crick base-pair rules that provide for the stability of DNA duplex structure and provide the means for faithful replication of DNA in times of cell division and (2) the fact that mRNA is composed of a single polynucleotide strand that leaves nucleotide residues free and available for Watson-Crick base pairing with segments of complementary, synthetic RNA supplied by exogenous means. In 1978, Zamecnik and Stephenson reported that oligonucleotides complementary to terminal nucleotides of mRNA inhibited transcription . The Zamecnik and Stephenson reports marked the beginning of a substantial drive toward antisense RNA (asRNA) biotechnology tools.
In 1986, Zamecnik et al. demonstrated proof-of-concept for exogenous asRNA effectors as agents for the treatment of human immunodeficiency virus (HIV) in vitro , thereby bringing asRNA therapeutics firmly under the spotlight . Unfortunately, the perceived potential of asRNAs as antiviral drugs or anticancer agents has not been realized in vivo and in the clinic, despite substantial commercial efforts to develop antisense medicines since the 1980s, partly because significant problems were encountered with the scale-up of nucleotide coupling chemistry and the cost of goods to manufacture. However, the greater problem has been that of medical plausibility. Famously, asRNAs can be demonstrated to bind complementary mRNAs in vitro , forming small regions of RNA duplex that provide a nucleus for mRNA destruction. However, this mechanism has rarely (if ever) been seen to mediate phenotypic therapeutic effects in vivo and in the clinic. Instead, complex off-target biological effects have been observed, involving unexpected mechanisms . Accordingly, the use of asRNAs to control mRNA levels and expression in vivo suffered from a loss in popularity and in medical plausibility.
ncRNAs to RNAi Therapeutics
The concept of RNAs as therapeutic agents was given a substantial boost in recent years with the realization that ncRNAs can make use of RNAi mechanisms to act as therapeutic agents against disease in their own right. Moreover, these mechanisms appear robust and are observable in vitro and in vivo . In other words, ncRNA-based therapies make use of RNAi mechanisms that genuinely operate naturally in vivo anyway. These RNAi mechanisms first surfaced in approximately 1995 when Guo and Kemphues reported that sense strand RNA actually inhibited gene expression in Caenorhabditis elegans to a similar extent as seen with asRNAs . Following this, Fire and coworkers showed that double-stranded RNAs (dsRNAs) homologous to a selected dsDNA target gene region strongly inhibited expression in C. elegans , to an extent approximately 10 times more than alternative single-stranded RNAs (ssRNAs) that were properly homologous with the sense or antisense strands of the selected dsDNA target . This observation led to the revelation of RNAi mechanisms for which a Nobel Prize was awarded in 2006. The award of a Nobel Prize highlights the huge impact that RNAi mechanisms have since had on cell biology and the more recent development of advanced therapeutics. RNAi mechanisms are now known to be universal to most cells of all multicellular organisms and as such provide a focal mechanistic system for the regulation of mRNA function.
RNAi mechanisms were analyzed initially in model species, such as C. elegans and Drosophila , in which long dsRNAs composed of several hundred nucleotide base pairs were shown to induce RNAi effects. After introduction into cells, these dsRNAs were found degraded systematically into short dsRNA segments by a specific enzyme called DICER. Post DICER processing, the resulting dsRNA segments were found to be composed of 20–25 base pairs with a two-base overhang protruding from the 3′ end of each strand . These short dsRNA segments were then strand separated, allowing one strand to become bound to an RNA-initiated silencing complex (RISC) to provide a perfect Watson–Crick base-pair recognition element for the molecular recognition and binding of complementary mRNA sequences followed by selective destruction of the mRNA message in a catalytic manner . These critical short dsRNA segments are now known as small interfering RNAs (siRNAs).
At first, the use of siRNAs as therapeutic agents was difficult. Their potential value to therapy rests with the critical fact that siRNAs might be added exogenously to cells or organisms, leading to the destruction of a complementary mRNA message of interest, gene knockdown, and downregulation of protein levels. However, at first, the exogenous administration of siRNAs appeared to cause only apoptosis or nonspecific inhibition of translation due to RNA-dependent protein kinase (PKR) activity . However, a critical breakthrough occurred with the synthesis and introduction to cells of shorter siRNA species composed of only 19–21 base pairs, resulting in clear RNAi mechanism-mediated effects and an absence of PKR activities . Since this discovery, siRNAs have entered the mainstream of RNA research and have become important new contributors to the development of advanced therapeutic agents acting in cells by RNAi mechanisms almost at will. Indeed, the potential of siRNAs to knock down critical genes involved in tumor development has been well recognized, with the intention of causing reductions or even reversals in tumor mass.
Today, RNAi mechanisms are considered strong, specific gene expression knockdown and protein-level downregulation techniques. Moreover, although RNAi mechanisms were first identified as being mediated by siRNAs, many other biologically significant ncRNAs are now known that operate in cells via RNAi mechanisms equivalent to or related to the mechanism outlined previously. These RNAi effectors include ncRNAs such as microRNA (miRNA), small nucleolar RNAs (snoRNAs), PIWI-interacting RNAs (piRNAs), long double-stranded RNAs (ldsRNAs), and large intergenic noncoding RNAs (lincRNAs) . Outside siRNAs, the therapeutic or target values of these newer ncRNA families are still yet to be adequately understood, but there will be value. At the same time, the potential value of RNAi therapeutics mediated by synthetic siRNAs is rapidly consolidating. Today, siRNAs can be considered the primary class of ncRNAs appropriate for use in RNAi therapeutic approaches for the treatment of cancers and other diseases. Nevertheless, the range of possible RNAi effector types capable of operating alongside siRNAs in RNAi therapeutics is increasing rapidly from historic as well as more modern sources, as we recount in the next section.
Potential RNAi or RNAi-Like Effectors for RNAi Therapeutics
Antisense RNA Effectors
Although asRNA data in vivo have been disappointing, the field may yet reemerge given new chemistries and potential new opportunities in RNAi therapeutics. Currently, asRNAs are still being evaluated in cancer therapy. For instance, various clinical trials to treat glioma have been conducted using asRNAs specific for the mRNA of insulin-like growth factor-1 (IGF-1) or transforming growth factor-β 2 (TGF-β 2 ), both of which are interesting targets. Activation of the IGF signaling pathway is involved in tumor growth and proliferation of gliomas . On the other hand, TGF-β 2 isoform is specifically overexpressed in malignant gliomas, thereby suppressing the immune system . However, asRNAs are less efficient than siRNAs when compared directly against similar mRNA targets. Undoubtedly, the different mechanisms involved will play a role in these differences . On the other hand, there has been an interesting shift of understanding related to the realization that certain ncRNAs, such as miRNAs, can be targeted for therapy by asRNAs. This is especially important given the roles that many intracellular miRNAs are showing in the promulgation of certain cancers . In such respects, asRNAs constructed from chemically modified locked nucleic acid (LNA) mononucleotide building blocks appear to be exceedingly promising . LNA oligonucleotides appear to be stable, potent, and capable of durable responses, and so they are currently under evaluation as potentially very powerful anti-miRNA effectors (antimiRs or antagomiRs) for a variety of indications .
Ribozymes
Ribozymes are RNA composite molecules that are capable of specific phosphodiester link cleavage either of a complementary exogenous RNA fragment or of self. Therefore, like asRNAs, ribozymes have potential anti-mRNA activities that could be classified on the fringes of RNAi mechanisms. Self-cleaving ribozymes were identified first at the beginning of the 1980s in the form of an intron with self-splicing activity. Heterogeneous cleavage was discovered at a later date. Typically, ribozymes possess nucleotide sequences that are complementary to a target RNA of interest; other sequences adopt a three-dimensional fold (e.g., hammerhead or hairpin) that positions a catalytic machinery close to a fissile bond in the target RNA sequence (exogenous or self). Ribozyme turnover is frequently slow in vitro , but lack of anti-mRNA potency is matched by duration of effects compared with selected siRNAs chosen to target the same mRNA .
Small hammerhead ribozymes are often used in ribozyme studies. These consist typically of 30–40 nucleotides that specifically cleave target RNA at phosphodiester links between nucleoside pairs defined by UN (where N is A, C, or U nucleosides). Until recently, synthetic ribozymes, such as anti-human EGFR-related-2 (HER2) ribozyme and anti-vascular endothelial growth factor receptor 1 ribozyme, were widely studied; however, the performance of ribozymes in vivo has been less encouraging, and problems first seen with asRNA are resurfacing with ribozymes. Recently, DNAzymes—oligodeoxynucleotides with ribonuclease (RNase) activity—were discovered . They were shown to efficiently block the replication of human papilloma virus and so may have clinical applications with medical plausibility ensured .
siRNAs
The basic theory behind siRNA was previously discussed. However, the real potential for siRNA therapeutics has only become clear following substantial work building on the initial work from the Tuschl laboratory with synthetic siRNAs. Most important, a fundamental reality is now well-established that any one mRNA target may be addressed by any number of siRNA effectors, but only very few will be capable of mediating substantial RNAi effects in vitro and in vivo . Much of this variability is a function of siRNA nucleotide sequence and structure.
However, a combination of well-developed bioinformatics tools based on a firm understanding of RNAi biology and siRNA physical behavior are capable of leading to the identification of “best-of-the-best” siRNA sequences that may operate with minimal off-target effects . As a rule, such sequences are also designed such that the antisense strands of siRNAs are those sequestered preferentially by RISC. Furthermore, careful chemical modifications to such siRNA structures can be used to improve the duration of siRNA-mediated effects, through improved intracellular stability and improved binding to RISC. Chemical modifications can also minimize adverse innate immune reactions to siRNAs that have been reported to be induced by G/U-rich regions in siRNA sequences . Chemical modifications of particular current utility are primarily 2′-OMe, 2′-F, and 2′-deoxy adaptations of U, or sometimes C, nucleotide residues, in siRNA sequences . Otherwise, the integration of locked nucleic acids into siRNA could also add value in both regards . Several clinical applications using siRNA are currently being developed, and some of our recent studies using siRNA for brain cancer treatment are described later in this chapter .
Short Hairpin RNA
Short hairpin RNA (shRNA) is an alternative way to prepare siRNA sequences for delivery to cells that can be expressed in situ from plasmid DNA (pDNA) or from virus-derived constructs. The effectiveness of shRNA was first reported by Paddison and Hannon in 2002 . In cells, DICER converts the hairpin structure of in situ shRNA into in situ siRNA that then engages with RISC in an optimal way for RNAi processing. A potentially beneficial effect of in situ shRNA expression may be to ensure that RNAi effects are more sustained (months) than might usually be expected postdelivery of synthetic siRNAs (few weeks maximum) . For both of these reasons, shRNA is considered a useful RNAi therapeutic agent with future potential .
MicroRNA
Of the many emerging new classes of ncRNA, miRNAs appear to play major roles in many intracellular epigenetic events. All miRNAs are small ncRNAs (18–24 nucleotide residues in length) that are cleaved from a hairpin-shaped precursor by an RNase, such as Drosha or indeed DICER. These miRNAs are conserved in evolution and so far number approximately 1,100 in human cells. These appear to have the ability to modulate gene expression at the RNA level by binding to the noncoding 3′ end regions of target mRNAs. Curiously, however, whereas siRNAs and RISC require substantial sequence complementarity between the AS strands of siRNAs on RISC and target mRNA sequences (typically two or three Watson–Crick base-pair mismatches are obviously tolerated, although a higher number of mismatches may be possible) , interactions between miRNAs and their corresponding target mRNAs are much less stringent. Indeed, only partial sequence complementarity seems to be required to inhibit protein translation. Functionally, miRNAs actually play a variety of different roles in physiological processes in many species, and more than 30% of human mRNAs are now thought to be controlled by miRNA binding events .
However, given the less well-defined nature of miRNA–target interactions, many in the field consider that miRNAs per se are not yet ready to form another general class of RNAi effector to rival the siRNAs. Clearly, further research is required. On the other hand, several miRNAs that have been discovered do appear to function as oncogene or tumor suppressors, with roles in tumorigenesis. For instance, miR-19 has been found to promote neoplastic transformation by targeting the expression of the tumor-repressor gene PTEN . On the other hand, high levels of the miRNA let-7, which targets the RAS oncogene, correlate with a good prognosis for survival and recovery from lung cancer . Similarly, miR-34a acts in human colon cancer cells to inhibit cell proliferation. The implication of such data is that miRNAs can be potential RNAi therapeutic agents as well as RNAi targets in their own right. In order to target miRNAs, chemically modified asRNAs have been developed recently (also known as antagomiRs or antimiRs) that have been shown to be active against a variety of miRNA targets .
Delivery Systems in RNAi Therapeutics
Whereas the technical problems in the design of effective and functional RNAi effectors have been convincingly solved or are near solution, one further problem requires urgent solutions—the problem of functional, efficient delivery in vivo . RNAi effectors such as siRNAs, miRNAs, and even antagomiRs and antimiRs are biopharmaceutical agents and as such can be capable of astonishingly high levels of specificity for identified disease-specific pathways. Consequently, as indicated previously, well-chosen sequence selection and chemical modification should result in high levels of efficacy and side effects that are either minimal or absent. However, in common with many other biopharmaceutical agents, RNAi effectors are essentially too high in molecular weight, too hydrophilic, and too metabolically labile to reach sites of disease easily in a functionally active form without the assistance of a delivery system or vector .
Therefore, delivery systems should be regarded as indispensible for functional delivery of RNAi effectors in vivo for almost every mode of delivery, such as intraocular , intravenous administration for access to the brain , or per oral administration for access to the systemic circulation from the gut . Only intranasal administration may pose an exception to this rule with regard to access to lung cells . In the field of RNAi therapeutics, delivery systems are derived largely from synthetic chemistry, chemical biology, or synthetic biology, although systems are being identified from other sources as well , and virus vector delivery systems also have a role to play.
Viral Vector Delivery Systems
Adenovirus, retrovirus, and lentivirus vectors have been widely used in gene therapy research and can be applicable to RNAi therapeutics approaches where in situ expression of shRNAs, miRNAs, antimiRs, antagomiRs, or asRNAs of interest is required. Adenovirus is one of the most commonly researched viral vectors. Most serotypes use the coxsackie- and adenovirus receptor for attachment and α v integrins for internalization, resulting in broad tissue tropism . The adenovirus genome is not dependent on host DNA polymerases, so it can infect both dividing and nondividing cells . Adenovirus can be manipulated relatively easily, produced at high titers, and its safety record in human populations is excellent . The adenovirus genome persists in the cell nucleus predominantly as an extrachromosomal episome and is absent from the progeny of transduced cells, resulting in transient gene expression and the need for repeat dose administration. Some RNAi studies using adenovirus vectors have been reported, mainly using shRNA.
Retroviruses and lentiviruses belong to the retroviral (Retroviridae) family of sense strand RNA viruses, which stably incorporate their own genomes into the chromosomes of host cells. Several characteristics of these vectors are attractive for gene therapy. For instance, they can be manipulated relatively easily, and they can accomplish efficient gene transfer in several mammalian cells. Their capacity to integrate genes into the host genome provides for long-term gene expression . In addition, these delivery systems may be used as RNAi vectors for in situ shRNA and miRNA expression. However, retroviral vectors can only transfect dividing cells due to their requirement for use of host DNA polymerase for the completion of the initial reverse transcription stage . Furthermore, the induced genes are incorporated at random positions on the chromosomal DNA, which is thought to carry an inherent risk of oncogenesis. Indeed, severe combined immunodeficiency patients who received gene therapy with γ-retroviral vectors developed leukemia . Lentiviruses can transfect nondividing cells and infiltrate nuclear membranes spontaneously without causing inflammation. Due to their stability in expression, transduction versatility across several cells, and long-term expression capabilities, lentiviral vectors have become the most studied and widely used vectors for gene therapy and RNAi therapeutics research in recent years.
Lipid-Based Nanoparticles: Nonviral Delivery Systems
Synthetic nonviral vectors represent potentially less expensive and more flexible delivery systems than viral delivery systems, and they are also amenable to simple, large-scale production. In addition, they are safer because of their tendency to elicit lower innate immunity and toxicity responses. Cationic lipid-based gene transfer was first reported in 1987 . Felgner and coworkers detailed the ability of cationic liposomes formulated from the cationic lipid N -[1-(2,3-dioleyloxy)propyl]- N , N , N -trimethylammonium chloride (DOTMA) to mediate both DNA and RNA delivery into mammalian cell lines . However, although cationic liposome–nucleic acid nanoparticles (lipoplex nanoparticles) have been evaluated in gene therapy clinical trials for the functional delivery of therapeutic nucleic acids, results have been disappointing . Primarily, lipoplex nanoparticles are unstable with respect to colloidal aggregation in biological fluids and quickly lose function unless delivered directly to a target site . Therefore, we are of the opinion that the best way forward for lipid-based nanoparticle delivery systems is to embrace the formation of lipid-based nanoparticles that conform to the synthetic, self-assembly ABCD nanoparticle paradigm .
ABCD nanoparticles consist of a core lipoplex nanoparticle ( AB nanoparticle) protected against immune surveillance and colloidal instability by a stealth/biocompatibility polymer layer ( C layer; typically polyethylene glycol). On top of this, an optional biological targeting ligand layer ( D layer) composed of receptor-specific ligands may also be attached if required. Using toolkits of different chemical components, a range of functional nanoparticles that conform to the ABC and ABCD nanoparticle paradigm have been described in the literature during the past few years, with a distinct focus on the delivery of siRNAs for RNAi therapeutics of viral infections and cancer . Several of these systems have entered clinical trials for a range of such indications (see http://www.rnaiweb.com/RNAi/RNAi_Web_Resources/RNAi_Therapy___Clinical_Trials ). Recently, we have used a novel cationic liposome system, LipoTrust EX Oligo (Hokkaido System Science, Hokkaido, Japan), which can form siRNA–lipoplex nanoparticles with some potential for in vivo use without recourse to the ABCD nanoparticle paradigm . Sato et al . reported on the use of vitamin A-coupled LipoTrust cationic liposomes to achieve functional delivery of anti-gp46 siRNA to fibrogenic hepatic cells for the treatment of liver cirrhosis .
RNAi Therapeutics with Anti-Mgmt siRNA for the Treatment of Malignant Glioma
Therapies for Malignant Glioma
Malignant glioma is one of the most formidable solid tumors currently known. Despite current multidisciplinary treatments, including surgery, radiotherapy, and chemotherapy, no significant prognostic improvement has been obtained during the past four decades. Also, despite the development of surgical and diagnostic technologies, total resection of the tumor cannot be achieved because of the infiltrative nature of glioma cells. In recent years, neuro-oncology has become one of the most rapidly developing fields. Temozolomide (TMZ), a small-molecule, oral alkylating agent, showed improvements in prognosis in a phase III randomized controlled study. However, the median survival time of patients with glioblastoma multiforme treated with a combination of 60-Gy radiotherapy and TMZ was reported to be 14.6 months—an extension of only 2.5 months compared to radiotherapy alone. This rather poor prognosis associated with malignant brain tumors treated with conventional therapies (e.g., surgery, radiotherapy, and chemotherapy) has led to efforts to develop new strategies for more effective advanced therapies. Gene therapy is one of the most promising approaches that may help overcome the limitations of these conventional treatments.
Glioma has unique characteristics compared with other cancers. It is separated from other organs by the blood–brain barrier (BBB), it rarely metastasizes to extracranial organs, and its neighboring normal neural cells do not usually divide. These characteristics are advantages for gene therapies because the induced genes or injected delivery systems are less likely to influence other organs. Gene therapy for malignant glioma has been studied from an early stage. To date, various approaches have been investigated , and several clinical trials of gene therapy for malignant glioma are currently underway involving several different agents at different phases ( Table 27.1 ).
Approved Year | Phase | Gene Type | Gene | Vector | Admin. Route | Principal Investigator | Country |
---|---|---|---|---|---|---|---|
1992 | I | Suicide | HSV-TK | Retrovirus | i.c. | Edward H. Oldfield | USA |
1993 | I | Suicide | HSV-TK | Retrovirus | i.c. | John C. Van Gilder | USA |
I | Suicide | HSV-TK | Retrovirus | i.c. | Corey Raffel | USA | |
I | Antisense | Antisense IGF-1 | Lipofection | s.c. | Joseph Ilan | USA | |
I | Suicide | HSV-TK | Retrovirus | i.c. | Larry E. Kun | USA | |
1994 | I | Cytokine | IL-2 | Retrovirus | s.c. | Robert E. Sobol | USA |
1995 | I | Suicide | HSV-TK | Retrovirus | i.c. | Michael Fetell | USA |
I | Cytokine | IL-4 | Retrovirus | s.c. | Ian Pollack | USA | |
I/II | Suicide | HSV-TK | Retrovirus | i.c. | L. Mariani | CHE | |
I/II | Suicide | HSV-TK, LacZ | Adenovirus | i.c. | Seppo Yla-Herttuala | FIN | |
1996 | I | Suicide | HSV-TK | Adenovirus | i.c. | Stephen L. Eck | USA |
I | Suicide | HSV-TK | Adenovirus | i.c. | Robert G. Grossman | USA | |
II | Suicide | HSV-TK, NeoR | Retrovirus | i.c. | J. Brotchi | BEL | |
I | Cytokine | IL-2 | N/A | i.c. | Nanno Harrie Mulder | NLD | |
I/II | Oncolytic virus | No transgene | HSV | N/A | Roy Rampling | GBR | |
III | Suicide | HSV-TK | Retrovirus | i.c. | Nikolai Rainov | Multi. | |
1997 | I | Drug resistance | MGMT | Retrovirus | i.v. | James M. Croop | USA |
I/II | Suicide | HSV-TK | Retrovirus | i.c. | Rolf W. Seiler | CHE | |
I | Suicide | HSV-TK | Retrovirus | N/A | Economou | GBR | |
1998 | I/II | Oncolytic virus | No transgene | HSV | i.c. | James Markert | USA |
I | Tumor suppressor | p53 | Adenovirus | i.c. | Frederick F. Lang | USA | |
1999 | I | Cytokine | IFN-β | Adenovirus | i.c. | Stephen L. Eck | USA |
I | Cytokine | GM-CSF | Plasmid | i.d. | Keith L. Black | USA | |
2000 | I | Cytokine | IL-4 | Retrovirus | i.d. | Hideho Okada | USA |
I | Cytokine | IFN-β | Lipofection | i.c. | Jun Yoshida | JPN | |
2001 | I | Receptor | IL-13 scFvFc-ζ TCR | Plasmid | i.c. | Michael Jensen | USA |
I/II | Growth factor | IGF-1 | Plasmid | s.c. | Heliodor Kasprzak | POL | |
2002 | I | Suicide | HSV-TK, Connexin 43, TNF-α | HSV | i.c. | L. Dade Lunsford | USA |
2003 | I | Cytokine | IL-12 | Plasmid | i.c. | Tom Mikkelsen | USA |
2004 | I/II | Oncolytic virus | No transgene | Adenovirus | i.c. | Frederick F. Lang | USA |
I | Suicide | HSV-TK | Adenovirus | i.c. | E. Antonio Chiocca | USA | |
I | Suicide | HSV-TK | HSV | N/A | Sunethra Wimalasundra | GBR | |
II/III | Oncolytic virus | No transgene | HSV | N/A | N/A | GBR | |
I | Suicide | HSV-TK | HSV | N/A | N/A | FRA | |
2005 | I | Drug resistance | MGMT | Retrovirus | N/A | Lars Martin Wagner | USA |
I | Oncolytic virus | No transgene | Poliovirus | i.c. | Darrell D. Bigner | USA | |
I | Receptor | Dominant Negative EGFR Mutant | Adenovirus | i.c. | William C. Broaddus | USA | |
I | Antigen | CMV pp65-LAMP | RNA transfer | i.d. | John H. Sampson | USA | |
I | Suicide | HSV-TK | HSV | N/A | Andreas Jacobs | DEU | |
2006 | I | Antigen | CEA | Measlesvirus | i.c. | Evanthia Galanis | USA |
I | Drug resistance | MGMT | Retrovirus | i.v. | Hans Peter Kiem | USA | |
I | Oncolytic virus | No transgene | HSV | i.c. | William T. Curry | USA | |
III | Oncolytic virus | No transgene | HSV | i.c. | Gareth Cruickshank | GBR | |
2007 | I | Cytokine | GM-CSF | Plasmid | i.d., s.c. | William T. Curry, Jr. | USA |
I | Suicide | HSV-TK | Adenovirus | i.c. | Mark W. Kieran | USA | |
I | Suicide | E. coli cytosine deaminase | Retrovirus | i.c. | Jana Portnow | USA | |
2008 | I | Drug resistance | MGMT | Lentivirus | i.v. | Santon L. Gerson | USA |
I | Cytokine | IL-12 | HSV | N/A | James M. Markert | USA | |
II | Tumor suppressor | CYP1B1 | Plasmid | s.c. | Amy B. Heimberger | USA | |
I | Antigen | Total tumor RNA | RNA transfer | i.v. | Duane A. Mitchell | USA | |
III | Growth factor | TGF-β 2 | Plasmid | s.c. | N/A | USA | |
I/II | Tumor suppressor | E1A deleted | Adenovirus | N/A | N/A | NLD | |
II | Antigen | tyrosinase | Adenovirus | N/A | Ma Ding | CHN | |
2009 | I | Receptor | CAR-HER2-CD28 Endodomain | Retrovirus | i.c., i.v. | Nabil Ahmed | USA |
I | Suicide | yCD 5FC | Retrovirus | i.c. | Manish Aghi | USA | |
I | Suicide | HSV-TK, Flt3L | Adenovirus | i.c. | E. Antonio Chiocca | USA | |
2010 | I | Suicide | yCD | Plasmid | i.c. | Peter Black | USA |
I/II | Antigen | CMV pp65 | Adenovirus | i.v. | Nabil Ahmed | USA | |
I | Receptor | Fas-TNFR Chimera transgene | Adenovirus | i.v. | Patrick Wen | USA | |
N/A | I/II | Suicide | HSV-TK | Lipofection | i.c. | J. Voges | DEU |
I | Suicide | HSV-TK | Retrovirus | i.c. | F. W. Floeth | DEU | |
II | N/A | N/A | Retrovirus | N/A | N/A | DEU | |
I | N/A | N/A | Retrovirus | N/A | N/A | DEU | |
I | N/A | N/A | HSV | N/A | N/A | DEU | |
I/II | Suicide | HSV-TK | Retrovirus | i.c. | Jose Maria Izquierdo | ESP | |
I/II | Suicide | HSV-TK | Retrovirus | i.c. | David Klatzmann | FRA | |
I/II | Oncolytic virus | No transgene | NDV | i.v. | Eitan Galun | ISR | |
I | Suicide | HSV-TK | HSV | i.c. | N. Adachi | JPN | |
I/II | Cytokine | IL-12 | SFV | N/A | N/A | GBR | |
I/II | Suicide | HSV-TK | Retrovirus | i.c. | Nanno Harrie Mulder | Multi. |
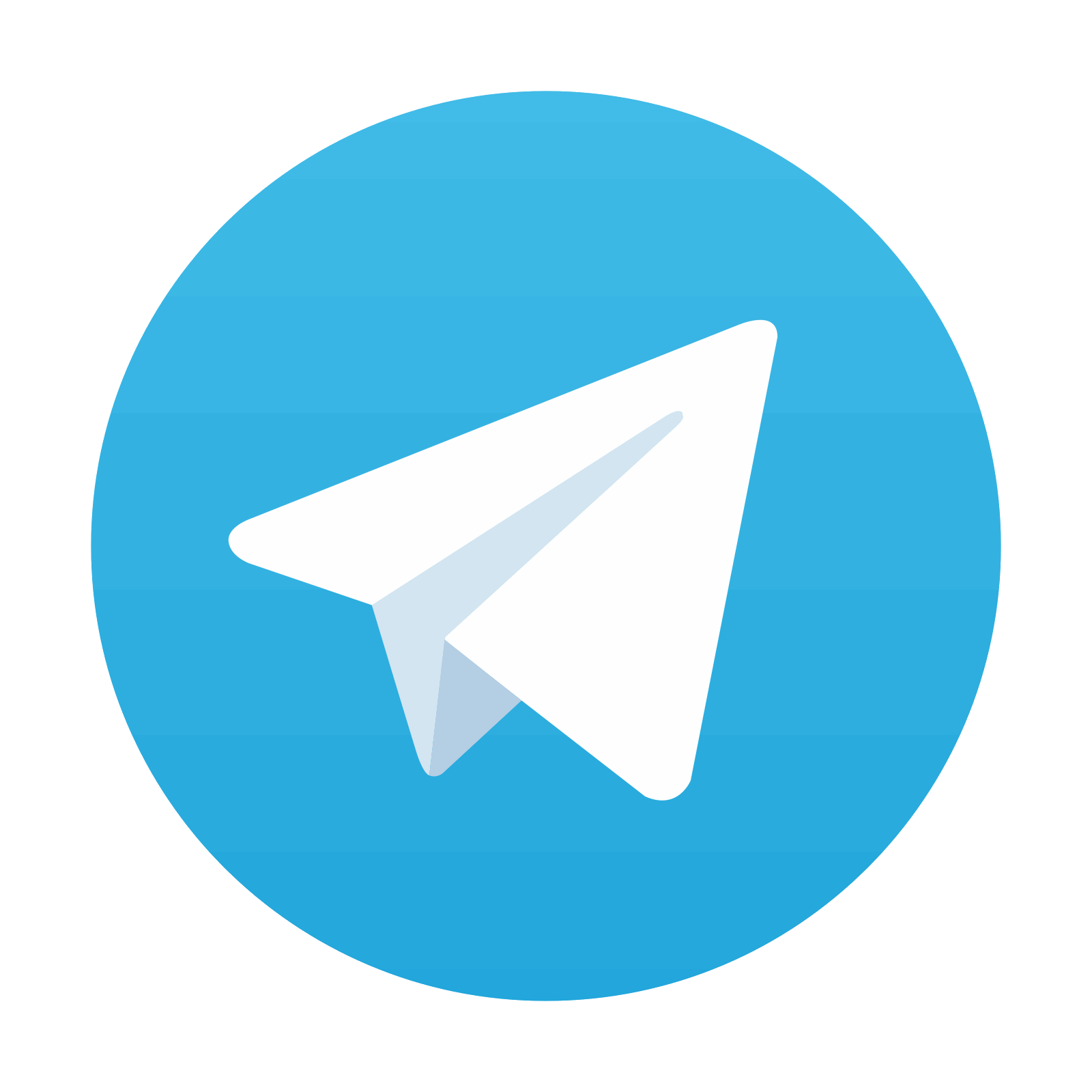
Stay updated, free articles. Join our Telegram channel

Full access? Get Clinical Tree
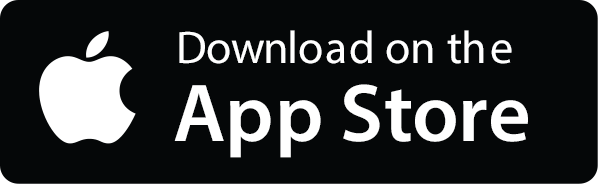
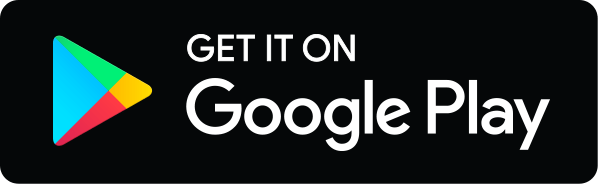
