1
Introduction
Diabetic retinopathy (DR) is the leading cause of visual impairment and preventable blindness, representing a significant socioeconomic cost for healthcare systems worldwide [ ]. The global prevalence of DR in the population with diabetes is around one-third and approximately one-tenth of these patients have the vision-threatening states typified by diabetic macular edema (DME) or proliferative diabetic retinopathy (PDR) [ ]. In addition, given that DR is the most common complication of diabetes, and diabetes is expected to increase from 366 million in 2011 to 552 million in 2030, DR will become an even more serious problem in the future [ ]. The potentially substantial worldwide public health burden of DR highlights the importance of searching for new approaches beyond current standards of diabetes care.
The tight control of blood glucose levels and blood pressure is essential in preventing DR development or arresting its progression [ ]. However, we are doing nothing specifically addressed to the eye until very advanced stages when laser photocoagulation, intravitreal injections (IVIs) of corticosteroids or anti-VEGF agents and vitreoretinal surgery are implemented [ ]. All these treatments are expensive, require a vitreoretinal specialist, and have a significant number of secondary effects. Therefore, new treatments for the early stages of DR (ESDR) are needed [ ].
In recent years, a growing body of evidence clearly shows that neurodegeneration is an early event in the pathogenesis of DR that could be linked to the development of microvascular abnormalities [ ] ( Fig. 8.1 ). In fact, the American Diabetes Association in its last position statement on this issue defines DR as a highly specific neurovascular disease [ ] and, consequently, it is no longer acceptable to consider DR as merely a microvascular complication. In order to integrate retinal microangiopathy and neuropathy into the definition of eye disease in diabetes, the term “diabetic retinal disease” (DRD), instead of DR has been proposed for a more comprehensive definition of the disease.
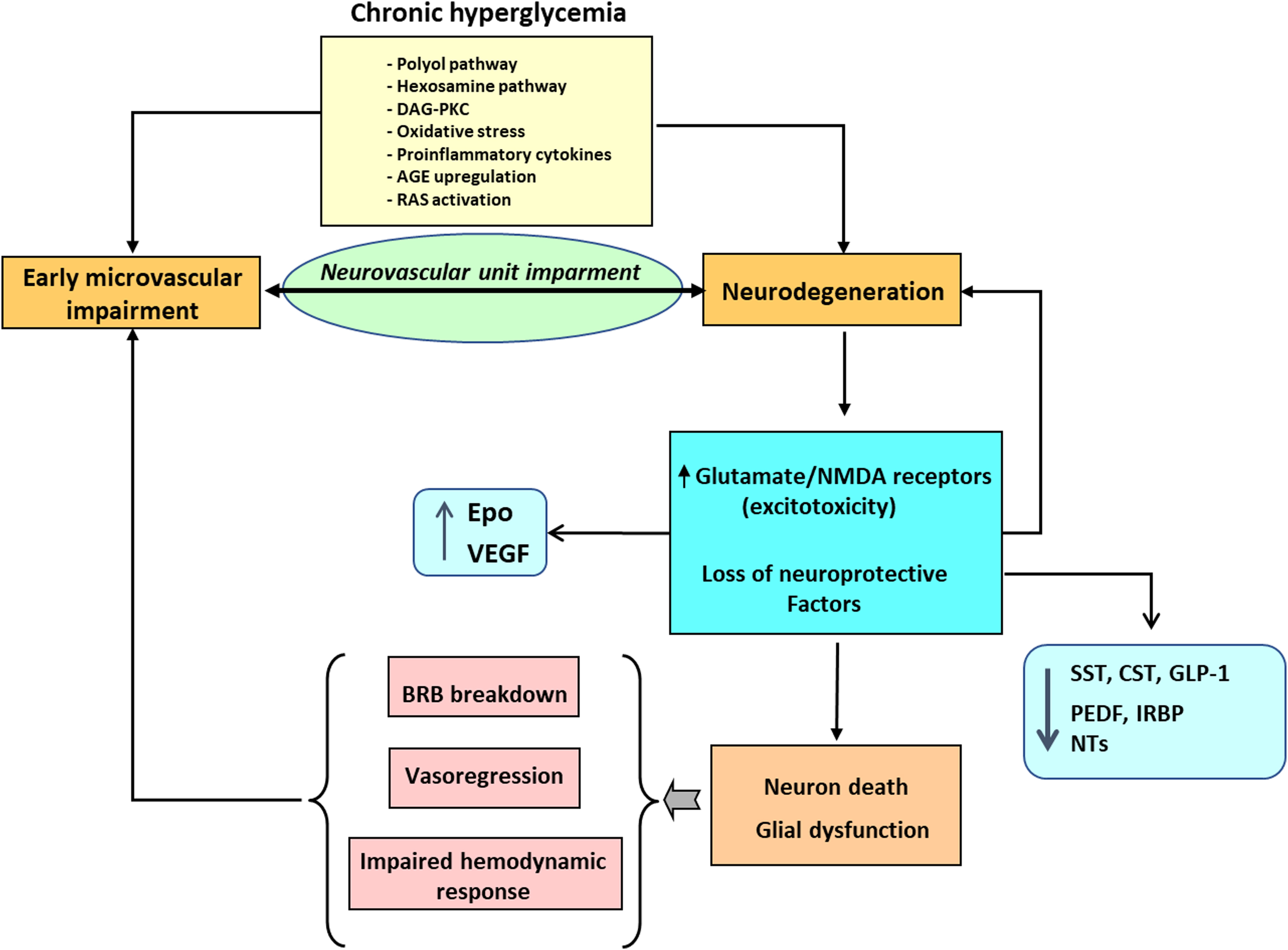
On this background, it is not surprising that the study of the underlying mechanisms leading to neurodegeneration and the identification of the mediators in the cross-talk between neurodegeneration and microangiopathy has emerged as essential not only for the better knowledge of the pathophysiology of DR but also for the development of new therapeutic strategies.
2
The neurovascular unit and its impairment in diabetic retinal disease
2.1
The NVU in physiological conditions
Retinal neurons and vasculature are organized in a stratified manner, with superficial vascular plexus lies in the nerve fiber layer (NFL), intermediate vascular plexus reside in the boundary between the inner plexiform layer (IPL) and inner nuclear layer (INL), while deep vascular plexus line the outer surface of the INL [ , ].
As is the case in the brain, the neurovascular unit (NVU) in the retina refers to the functional coupling and interdependency of neurons, glia, and the highly specialized vasculature ( Fig. 8.2 ). The components of the NVU include diverse neural cell types (i.e., ganglion cells, bipolar cells, horizontal cells, and amacrine cells), glia (astrocytes and Müller cells) immune cells (microglial cells), and vascular cells (endothelial cells and pericytes) [ , , ]. All these components are in intimate communication and maintain the integrity of the inner blood–retinal barrier (iBRB) while dynamically regulating blood flow in response to metabolic demands [ , ]. Within the NVU, some types of cells, such as glial cells, endothelial cells, and pericytes, can communicate directly through physical interactions, while cells without physical connections communicate at distance via secreted soluble ligands and/or exosomes.
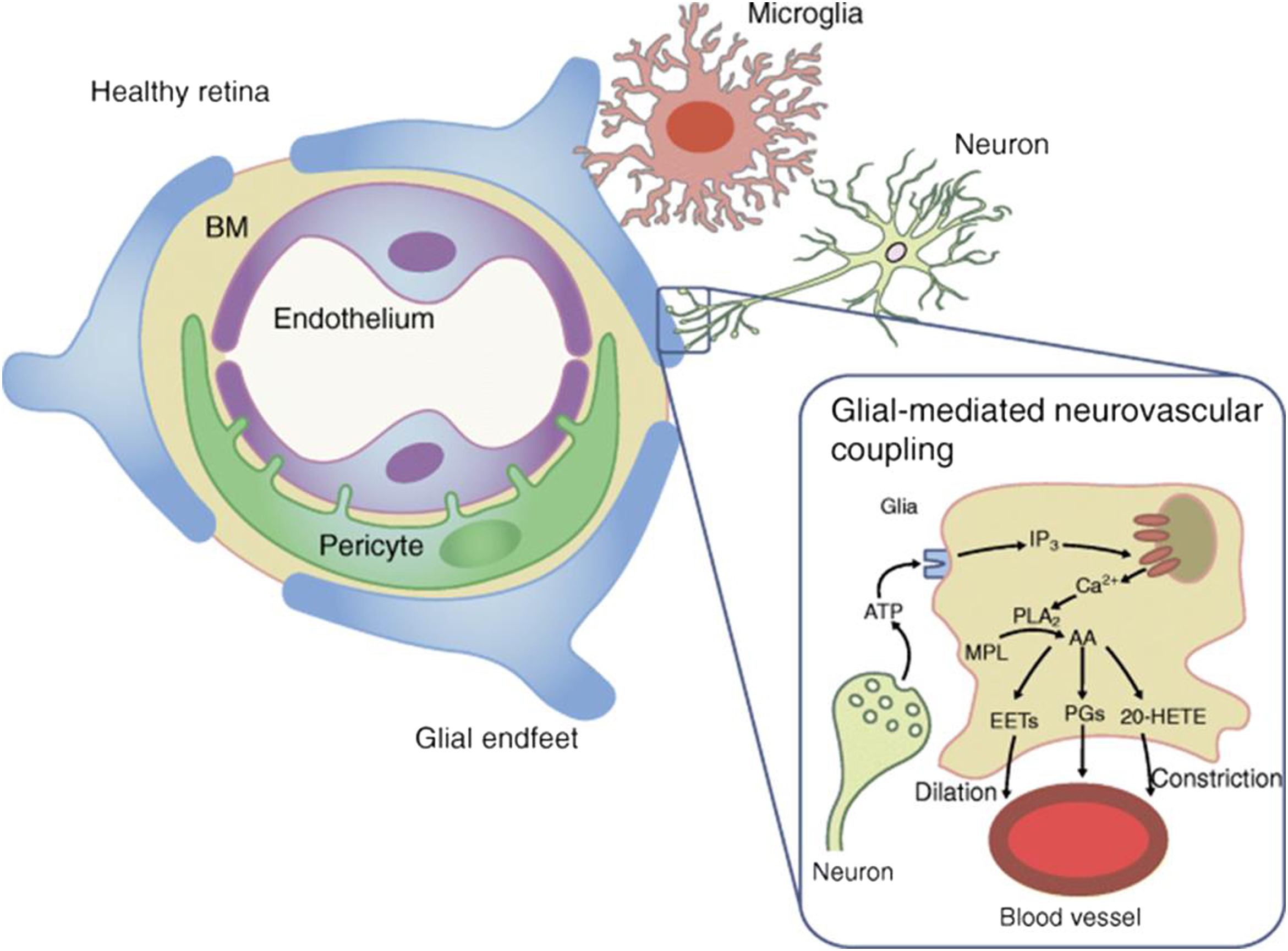
Neurons transmit the electrical impulses converted by photoreceptors from light signals to the brain to form vision. However, they also regulate local blood flow through glial cells and pericytes to maintain their functions [ ].
Müller cells and astrocytes are two types of macroglia in the retina. Müller cells have a wide distribution and the most abundant (90%) glial cells [ ]. They penetrate almost all retinal layers and contacting with a variety of retinal cells, being essential to maintain retinal homeostasis and protection of neurons. Their processes wrap around the blood vessels and coordinate vascular responses to provide nutrients to the outer retina, maintain extracellular pH value, recycle transmitter, and transport metabolites [ ]. Astrocytes are found only in the NFL and GCL [ ]. They encircle the neuronal axons and vessels to form an irregular network to support the integrity of the inner BRB [ ]. They may function partially the same as Müller cells and play a role in the maintenance of vascular tone by autoregulation [ ].
Microglia are resident immune cells that monitor their surroundings [ ]. In physiological conditions, microglia spread over the NFL, GCL, IPL, INL, and OPL of the retina [ ], but in pathological conditions, these cells can be present in the ONL [ ]. Microglial cells are in direct contact with neurons and retinal pericytes [ ]. As highly specialized phagocytic cells, microglia continuously monitor local synaptic activity and sweep away dying cells as well as metabolic debris [ , ]. In physiological conditions and acute inflammation, microglial cells produce anti-inflammatory cytokines and neurotrophic factors that support neural retina health [ ]. However, in a chronic retinal disease such as DR, they will secrete a large number of proinflammatory cytokines, affecting the hemostasis of NVU [ , ].
The main role of endothelial cells is to form the inner BRB by means of the intercellular tight junctions and regulates the flux of fluid and macromolecules between the blood and neural retina [ ]. Pericytes envelope retinal capillaries and given their contractile properties participate in blood flow regulation [ ]. Apart from endothelium, pericytes are intimately associated with neurons glial cells and microglial cells, thus contributing significantly to maintaining NVU [ ].
Finally, extracellular matrix and in particular the constituents of basement membrane (i.e., laminin, nidogen, collagen IV, and fibronectin) plays a critical role in the NVU by providing a beneficial environment between endothelial cells and pericytes and governing the diffusion of ions and neurotransmitters [ ].
Neuronal and glial-mediated neurovascular coupling is essential for the normal homeostatic function of NVU. Neurovascular coupling is the intrinsic physiological mechanism by which neural activity is coupled to blood flow and metabolism, thus enabling the retina to regulate blood flow in response to neural activity or metabolic demands. Visual stimulation is a powerful modulator of retinal and optic nerve blood flow [ ], and flicker light stimulation (intermittent flash) has been used to investigate this process because it increases neural activity. This increase of neural activity leads to retinal arterial and venous dilation [ ].
2.2
The NVU in DRD
Any disruption in the retinal NVU may have pathophysiological influences on each type of component cells, leading to impairment in the structure and function of microvasculature and neurons. In this chapter, we will mainly emphasize the changes in nonvascular components of the NVU. Neural apoptosis and reactive gliosis are the most visible histological features in ESDR ( Fig. 8.3 ).
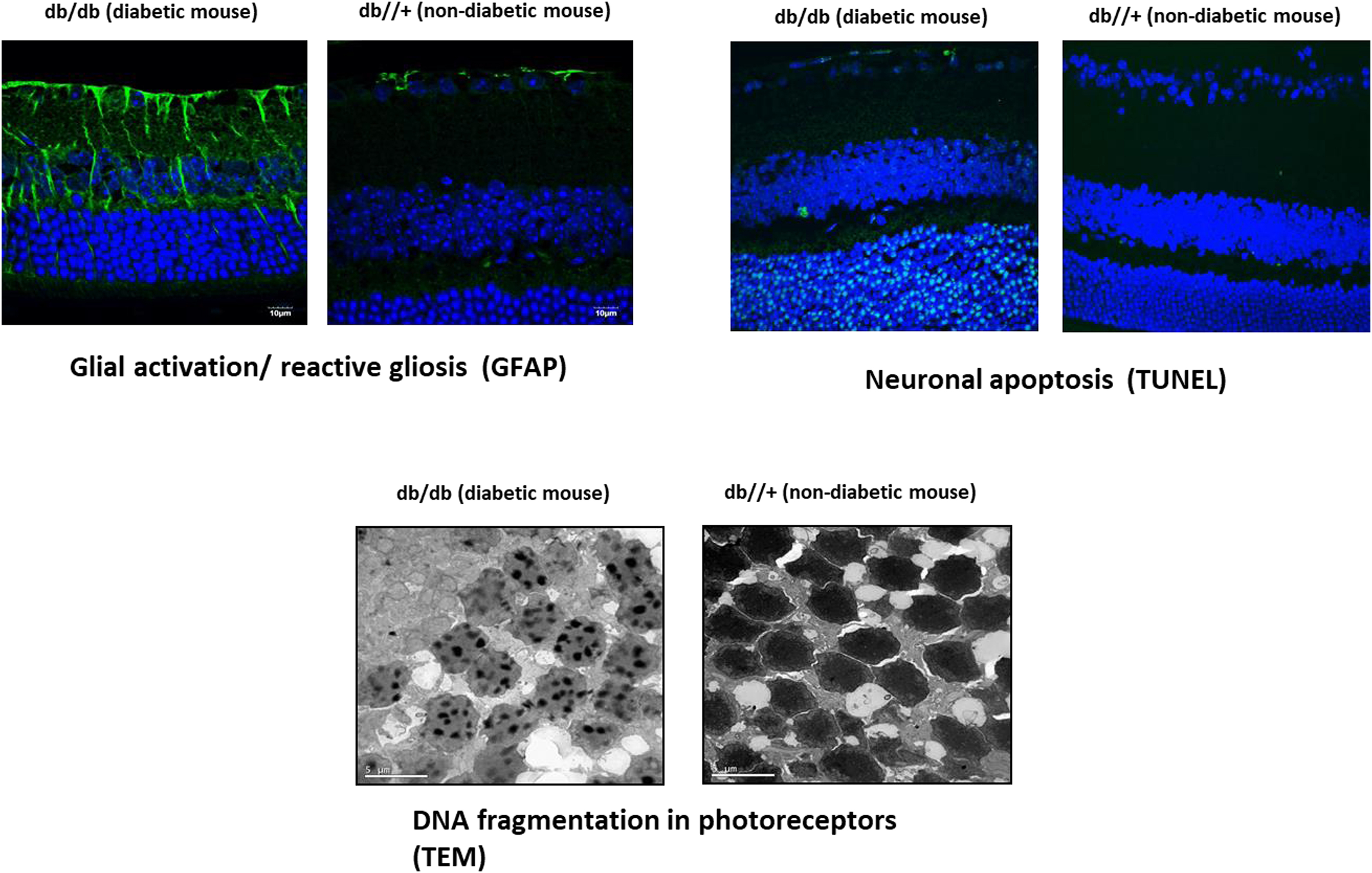
2.2.1
Neural apoptosis
Retinal ganglion cells and amacrine cells are the first components of the neuroretina in which diabetes-induced apoptosis is detected [ ]. This has been observed in both murine models and humans prior overt microvascular lesions can be detected [ , , , ]. Photoreceptors also have an increased apoptotic rate and play an important role not only in the neurodegenerative process but in triggering microvascular impairment [ , ]. A clear imbalance between proapoptotic and survival signaling exists in the neuroretinas of diabetic patients in the early stages of DR [ ].
The mechanisms involved in neural apoptosis are multiple and comprise the general pathways triggered by hyperglycemia (polyol pathway, hexosamine pathway, DAG-PKC, AGEs, oxidative stress, proinflammatory cytokines), and other more genuine in the setting of DRD such as glutamate excitotoxicity, decrease of neurotrophic factors, and downregulation of synaptic and structural proteins essential for axonal transport [ , , , ]. In addition, mediators of brain neurodegenerative disease have been identified in retinas from diabetic donors in ESDR [ ]. All these mechanisms deserve a brief comment.
Glutamate excitotoxicity refers to deleterious effect of the excess of extracellular glutamate on retinal neurons. This increase in glutamate leads to overactivation of ionotropic glutamate receptors, mainly alpha-amino-3-hydroxyl-5-methyl-4-isoxazole-propionate (AMPA) and N-methyl- d -aspartate (NMDA) receptors, which results in an uncontrolled intracellular calcium response in postsynaptic neurons and cell death [ ]. The main mechanisms involved in extracellular accumulation of glutamate in DR are the following: (1) Downregulation of high-affinity l -glutamate/ l -aspartate transporter (GLAST), which is the main transporter of extracellular glutamate into Müller cells [ ]; (2) reduction of the Müller cell specific enzyme glutamine synthetase, which converts glutamate to glutamine [ ]; and (3) decrease in the retinal ability to oxidize glutamate to alpha-ketoglutarate [ ].
Diabetes induces the downregulation of several proteins with neurotrophic activity synthesized by the retina, which are essential for retinal homeostasis. Among these proteins pigment epithelial growth factor (PEDF) [ ], somatostatin [ ], interphotoreceptor retinoid-binding protein (IRBP) [ ], and glucagon-like peptide 1 (GLP-1) [ ] are the most relevant, and its replacement treatment can be considered as a new strategy for treating EASD [ ].
A reduction of retinal content of several presynaptic proteins critical to the exocytosis of neurotransmitters and the maintenance of synaptic connectivity have been found in experimental models of diabetes [ , , ]. In addition, in postmortem human retinas the protein content of synapsin one and other neuronal proteins (i.e., synaptophysin, SNAP-25, and neurofilament) is depleted in comparison with nondiabetic donors [ ].
Several pathways shared with other neurodegenerative disorders, such as Alzheimer’s and Parkinson’s disease, have been identified in retinas from diabetic donors in EASDR [ ]. This finding supports the concept that a common soil exists in the neurodegenerative processes that occur in the retina and brain and suggests that the study of neurodegeneration in the diabetic retina could be useful to further understand the neurodegenerative processes that occur in the brain of persons with diabetes.
All the above mechanisms lead to neuron apoptosis, which leads to a reduction in the thickness of inner retinal layers and the NFL. These structural and functional changes have clinical implications regarding such visual deficiencies as decreased hue discrimination, less contrast sensitivity, delayed dark adaptation/adjustment, and abnormal visual fields, leading to a reduced vision-related quality of life [ ].
2.2.2
Glial activation and inflammation
Neural apoptosis is accompanied by changes in glial cells (astrocytes and Müller cells) known as “glial activation” or “reactive gliosis.” At present, it is unknown whether neural apoptosis or reactive gliosis is first in the neurodegenerative process that occurs in the diabetic retina [ ]. One of the most significant characteristics of reactive gliosis is glial acidic fibrillar protein (GFAP) overexpression. In normal conditions, only retinal astrocytes express GFAP; however, in DR, an aberrant upregulation of this intermediate filament protein is shown in Müller cells, which can be used as a marker of reactive gliosis in the retina [ ]. These activated glial cells fail to perform their physiological functions to maintain tissue homeostasis, especially regulation of retinal blood flow, water balance in the neural parenchyma, and maintenance of barrier integrity [ ]. Therefore, the impairment of glial cells plays a pivotal role in linking neurodegeneration and microvascular impairment in the diabetic retina [ ]. Moreover, activated Müller cells secrete various inflammatory cytokines resulting from the overexpression of innate immune-related pathways [ ]. These increased levels of inflammatory mediators may lead to an early, persistent chronic inflammatory condition in the diabetic retina resulting in leukocyte activation, adhesion to the vascular endothelium (leukostasis), and alteration of the BRB [ ]. Among the proinflammatory cytokines/chemokines involved in the pathogenesis of DR the IL-1b, IL-8, TNF-α, monocyte chemotactic protein-1 (MCP-1), stromal cell-derived factor-1 (SDF-1), and the adhesion molecules (ICAM-1, VCAM-1, sVAP-1) play a relevant role [ ]. It is worth mentioning that the concentration within the vitreous fluid of diabetic patients of some of these proinflammatory cytokines (i.e., MCP-1, IL-8) has been reported as being within the same range as that observed in the pleural effusions of patients with pneumonia or tuberculosis, and correlated with PDR activity [ ], thus underscoring its role in the pathogenesis of DR.
Among the glial cells, microglia attracted the attention of researchers in last decades. Microglia are the resident immune cells in the retina, and their main function is immune surveillance [ ].
The activation can be observed by the morphological changes, from ramified to ameboid morphology. At the early stage of DR, the major effects of activated microglia are phagocytosis of apoptotic cells, clearance of debris, and secretion of neurotrophic molecules. Nevertheless, as DR progresses, persistently activated microglial cells become detrimental to retinal neurons and vasculature via release of inflammatory cytokines [ , ].
Pyroptosis is an emerging type of inflammatory cell death inextricably linked with inflammation [ ]. The caspase-1 mediated pathway, which is activated by NLR family pyrin domain containing 3 (NLRP3) and NLRP1 inflammasomes, is the canonical inflammasome pathway that triggers pyroptosis. Recent studies have indicated that these inflammasomes are associated with NVU dysfunction may play a crucial role in the changes in retinal cells in the DR environment [ ].
2.2.3
Microvascular impairment
A diminished vasodilation capacity has been found in very early stages of DR without overt morphological changes [ ]. In terms of histopathological alterations at the early stage of DR, vascular basement membrane thickening, as well as the demise of pericytes and endothelial cells, has been observed [ ]. When endothelial cells die, retinal capillaries become acellular. This so-called vasodegeneration or vasoregression is a central pathogenic response to chronic hyperglycaemia and initiates the progressive ischemia characteristic of DR [ ].
Recent research has revealed that in hyperglycemic conditions, pericytes detached from the underlying endothelial cells, migrated off the vessels and formed bridges across two or more capillaries [ ]. Increased pericyte bridges were observed in diabetic animal models several months before loss of any pericytes or endothelial cells, suggesting that this is associated with the subsequent pericyte loss and can be used as an indicator of vascular damage [ ].
3
Methods to assess neurodegeneration
The diabetes-induced changes of NVU cause retinal damage in terms of functional and/or structural abnormalities, which have been well-documented in patients with no or minimal microvascular retinopathy. In this chapter, the changes related to the nonvascular components will only be commented.
3.1
Examinations addressed to assess retinal neurodysfunction
Retinal neurodysfunction can be detected by a lot of methods even earlier that morphological changes can appear. In this chapter, we will focus on two of most relevant: electroretinography and microperimetry.
3.1.1
Electroretinography
The electroretinogram (ERG) is a noninvasive, objective technique to evaluate neural dysfunction in patients with diabetes. There are several methods such as the full-field ERG, multifocal ERG (mfERG), and pattern ERG. Perhaps, the two most important findings that have come from ERG studies in diabetics over the last 20 years are: (1) the early involvement of photoreceptors and bipolar cells and (2) the power of the mfERG in predicting the developing and progression of vascular abnormalities [ ]. The mfERG is well-suited to evaluate neural function in diabetes, given that early vascular abnormalities such as microaneurysms, dot hemorrhages, and infarcts often occur in spatially localized patches. The main abnormalities detected in patients with diabetes are a delayed implicit time and the amplitude reductions in oscillatory potentials and scotopic b-waves [ ]. The problem of mfERG is that it is time-consuming and cumbersome for general application, and, therefore, its use has been limited to clinical research studies.
The flicker ERG is a highly promising approach that has gained popularity over the last 2 decades. This is due in part to the recent availability of a portable ERG instrument that bases its quantification of a DR score on the flicker ERG. Furthermore, a series of articles has suggested that the high-frequency flicker ERG may be a sensitive tool for assessing neural dysfunction in diabetics, although these studies remain to be replicated in large clinical samples. Thus, the flicker ERG appears to be a promising protocol for use in future clinical studies, and there is hope that this approach will soon be evaluated in large clinical trials [ ].
3.1.2
Microperimetry
Fundus-driven microperimetry has emerged as a simple, noninvasive, and rapid test (takes around 6–7 min to complete) that can be used in clinical practice [ , ]. Microperimetry measures retinal sensitivity in terms of the minimum light intensity that patients can perceive when spots of light stimulate specific areas of the retina.
Retinal sensitivity assessed by microperimetry has been correlated with ganglion cell layer-inner-plexiform layer (GCL-IPL) thickness and ganglion cell count in subjects with diabetes. A total reduction of 27 μm in total retinal thickness results in approximately 1 dB of sensitivity loss in subjects with mild nonproliferative DR [ ]. Some recent studies have suggested that microperimetry is even more sensitive than multifocal electroretinography in detecting early functional changes of the retina [ , ].
Several variables should be considered when interpreting output from microperimetry. These include age, presence of macular edema, area of capillary nonperfusion, presence of cataracts, degrees of macular area analyzed, number of stimulus points and the type of device, and mesopic adaptation. In addition, cognitive impairment can decrease retinal sensitivity, and, therefore, this variable should be considered when interpreting the results [ ]. Other limiting factors are the lack of a normative database stratified by age, gender, and ethnicity, and the scarce information on the association between retinal sensitivity assessed by microperimetry and the diabetic retinopathy severity scale (DRSS).
3.2
Examinations to assess changes in retinal morphology related to neurodegeneration
3.2.1
Spectral domain optical coherence tomography
Spectral domain optical coherence tomography (SD-OCT) permits to obtain a high-quality anatomical image of retinal layers. The progressive loss of retinal ganglion cells leads to a significant reduction of neuronal and axonal layers at macula that can be detected by SD-OCT in patients without overt DR [ ]. This finding support retinal neurodegeneration an early event in the pathogenesis of DR. However, glial activation may precede the onset of neuronal death [ ], thereby obfuscating the detection of retinal NFL thinning. In addition, vascular leakage that occurs in the early stages of DR could also contribute to retinal thickness, thereby also acting as a confounding factor. The segmentate analysis taking into account the different thickness of retinal layers could help in clarifying this issue. However, the normative values are not as well established for thickness of individual retinal layers as they are for total retinal thickness. Therefore, for an individual patient, change in thickness over time may be more clinically relevant than thickness measurement at a given point in time. Among others, age, refractive error, axial length, sex, ethnicity, glaucoma, cognitive impairment, and genetic factors have been reported to impact GC-IPL thickness [ ] and, therefore, should be taken into account in the analysis of the results. There are few prospective studies evaluating the relationship of this parameter to outcomes in DRD, such as vision loss, response to treatment or DRD progression, and, therefore, more research on this issue is urgently needed.
4
Clinical implications
The large body of evidence showing that neuronal impairment is an early event in DRD points to its assessment as important in terms of screening, classification, and therapeutic approaches.
Recent results from the EUROCONDOR study showed that a significant proportion of individuals with type 2 diabetes present with early microvascular disease without detectable neurodysfunction [ ]. Therefore, it seems that neurodegeneration is not always the apparent primary event in the natural history of DR. In this regard, it is possible that neurodegeneration could herald DR in some subsets of patients, but neurodegeneration and microvascular disease could occur independently in others. Therefore, measurements of retinal neurodysfunction/neurodegeneration combined with methods addressed to measure microvascular impairment (e.g., OCTA) will allow a better phenotyping and stratification of patients with DRD. In fact, a new classification of DRD combining microvascular impairment and neurodegeneration has been recently proposed [ , ]. This new evidence raises the question about what examinations should be included in the screening of DRD in order to discriminate those patients in whom neurodegeneration could play a significant pathogenic role. At present, there are no clinical evidence on this issue; however, the portable ERG and SD-OCT seem promising cost-effective examinations to be included in the current screening of DRD.
Clinical recording of the ERG may have implications for the care of diabetic patients. For example, an objective test that is capable of quantifying neural dysfunction prior to the clinical appearance of retinopathy could help set individual goals for glycemic control. Furthermore, work is underway to determine if diabetic patients who have flicker ERG abnormalities are more likely to progress in NPDR stage. If this is the case, then the flicker response may be a useful tool for triaging patients and determining the optimal individualized follow-up intervals for diabetic patients without clinically apparent retinopathy. That is, some patients who have no clinically apparent retinopathy may need to be followed more frequently than others. Although there is much enthusiasm for expanding the use of ERG beyond the research setting into the clinic, uncertainty as to the optimal test strategy and method of analysis for detecting and following the progression of DR have been barriers in wider implementation.
Finally, it should be stressed that apart from its eventual participation in microvascular disease, retinal neurodegeneration has itself clinical implications in terms of deficiencies in sensory capacity, including decreased hue discrimination, contrast sensitivity, delayed dark adaptation, and abnormal visual fields and thus result in reduced vision-related quality of life [ ]. In this regard, it is notable that the loss of neuroretinal thickness (nerve fiber layer, ganglion cell layer, and IPL) in people with diabetes with no or minimal DR is around 0.54 μm per year [ ]. This means a loss of 5.4 μm over 10 years and, remarkably, is equivalent to the loss found in severe glaucoma.
5
Therapeutic implications
At present, there are no drugs recognized by any Academic Society for the treatment of the early stages of DR. Therefore, only general measures, such as tight metabolic control in terms of blood glucose levels and lipids, as well as good control of blood pressure, are recommended. Gene therapy and advanced treatment with organoids are promising but seem still far to be implemented in the setting of randomized clinical trials. Therefore, we will focus on treatments addressed to improve NVU with more probabilities to reach the market in the coming years.
5.1
Systemic administration
Two drugs (fenofibrate and calcium dobesilate) have been shown to be effective and safe in treating DR in several clinical trials, but more research to better understand the mechanisms of action and the clinical outcomes associated with their use is needed [ ]. A study, comprising 910 participants with 4 years of follow-up, is expected to finish in April 2027 and should further enlighten the scientific community regarding the usefulness and safety of fenofibrate for treating early stages of DR ( ClinicalTrials.gov Identifier: NCT04661358). Regarding calcium dobesilate, a single-blind, multicenter, cluster-randomized trial including a total of 1272 Chinese patients with mild-to-moderate NPDR is ongoing ( ClinicalTrials.gov Identifier NCT04283162). However, the planned follow-up of only 12 months seems a serious limitation of this study.
5.2
Topical (eye drops) administration
Although fenofibrate and calcium dobesilate have found to be effective and safe in a few clinical trials, the systemic administration of drugs to block the main pathogenic pathways involved in DR have two main general problems. First, they can hardly reach the retina at pharmacological concentrations. Second, systemic administration could have serious adverse effects and lead to pharmacologic interferences with other drugs used for the treatment of diabetes and its co-morbidities. Furthermore, when the early stages of DR are the therapeutic target, it would be inconceivable to recommend an aggressive treatment, such as frequent intravitreal injections. In addition, topical administration of drugs limits their action to the eye and minimizes the associated systemic effects. For all these reasons, topical treatment has been postulated as new strategy for treating early stages of the disease [ ]. In recent years, several reports have demonstrated the effectiveness of eye drops containing small molecules in treating early stages of DR in experimental models, and it seems that they are reaching the retina by the trans-scleral route [ ]. A recent systematic review found a few clinical studies using topical eye drops of corticosteroid or nonsteroidal anti-inflammatory drug (NSAID) reporting favorable effect on DME [ ]. In addition, two studies [ , ] found neuroprotective agents able to arrest further development in patients with pre-existing signs of neurodegeneration and one found such an agent to induce changes in retinal vascular caliber in mild DR. It is reasonable to expect that the improvement of drug delivery by nanotechnology could increase the efficiency and delay the frequency of topical administration. Taken together, topical eye drops open up a new strategy for treating DR, but there is still a need of large randomized clinical trials with longer follow-up periods.
5.2.1
Treatment with neurotrophic factors downregulated in the diabetic retina
One of the more rational strategies for treating diabetes-induced retinal neurodegeneration is the “replacement” treatment of the neurotrophic factors downregulated in early stages of DR such as pigment epithelial derived factor, somatostatin, and GLP-1R agonists.
Pigment epithelial derived factor (PEDF). This is a protein mainly synthesized by retinal pigment epithelial with neuroprotective and antiangiogenic properties and the most important natural inhibitor of angiogenesis, which is downregulated in DR [ ]. These two essential properties place PEDF as a suitable candidate for new therapeutic approaches to treat DR. However, its application is restricted because of its instability and short half-life. In addition, PEDF size may limit its utility as a topical therapeutic agent, and, therefore, some synthetic PEDF-derived peptides containing biologically active fragments are needed. In this regard, the topical administration (eye drops) of antiangiogenic PEDF60–77 and neuroprotective PEDF78–121 derivatives reduced neurodegeneration and microvascular leakage in the Ins2(Akita) mouse [ ]. Furthermore, eye drop delivery of PEDF-34 promoted ganglion cell survival and axon regeneration after optic nerve crush injury in rats [ ]. Gene therapy using AAV-mediated PEDF [ ] or the engineered episomal vector pEPito (pEPito-PEDF) [ , ] has also exhibited promising results in experimental in vivo models.
SST (somatostatin). This is a peptide abundantly produced by the retina, RPE being the main source [ , . Apart from neuromodulation SST participates in vessels stabilization and in the regulation of ion–water transport systems [ ]. A downregulation of SST production occurs in early stages of DR and becomes more pronounced in advanced stages [ , ]. Consequently, SST replacement can be considered a therapeutic target not only for preventing the neurodegenerative process but also for more advanced stages of DR, such as DME and PDR. The promising pilot studies published at the beginning of this century showing the effectiveness of SST analogs administered by intramuscular injections for treating advanced DR [ , ] have not been confirmed in larger studies. The main reasons why the systemic administration of SST analogs failed to arrest the progression of DR were recently reviewed [ ]; one of the most important was their inability to cross the BRB. By contrast, topical administration of SST prevented retinal neurodegeneration and neurodysfunction assessed by ERG in streptozotocin (STZ)-induced diabetic rats [ ] and in db/db mice [ ]. This preclinical evidence triggered the implementation of the EUROCONDOR study, the first in humans using neuroprotective drugs for treating early stages of DR. This phase II-III clinical trial was performed in type 2 diabetes using two neuroprotective agents (SST and brimonidine) administered by eye drops. The main result was that SST administered by eye drops was able to arrest the progression of neurodysfunction, but no effects were observed in preventing or arresting microvascular disease [ ]. The short follow-up (2 years), the inclusion of a high proportion of patients with no or very mild microvascular disease, and the excellent metabolic control during follow-up could explain the absence of beneficial effects in arresting microvascular progression. Nevertheless, topical treatment with either brimonidine or SST caused retinal arteriolar and venular dilation in those patients with preexisting early DR [ ]. Therefore, this study demonstrated, for the first time, that long-term topical neuroprotection-induced retinal vascular changes in patients with diabetes, thus opening up a new strategy for treating early stages of DR.
GLP-1. GLP-1 is expressed in human retinas, mainly in the ganglion cell layer (GCL), and is downregulated in diabetes. By contrast, no differences in GLP-1R expression exist in either the RPE or neuroretina between donors with and without diabetes [ ]. The neuroprotection of GLP-1RAs in terms of preventing ERG abnormalities and morphological features, such as glial activation and neural apoptosis, by using intravitreal injections has extensively been reported [ ]. However, as previously mentioned, in the early stages of DR, intravitreous injections are inappropriately invasive. In this regard, the topical administration of GLP-1 or GLP-1R agonists (i.e., semaglutide) was found to be able to prevent the retinal neurodegenerative process in a spontaneous model of type 2 diabetes (db/db mouse) [ , ]. Recently, it has been reported that topical administration of GLP-1 not only prevented but also reverted reactive gliosis and restored the number of neuron cells in db/db mice, thus suggesting a neurogenic effect [ ]. The main mechanisms involved in neuroprotection conferred by GLP-1RAs as follows [ ]: (1) Anti-inflammatory action; (2) inhibition of the excitotoxicity and neuron death mediated by glutamate accumulation in the extracellular space; (3) antiapoptotic action; and (4) Antioxidant properties. In addition, GLP-1RAs also exert a direct microvascular protection, thus ameliorating the disruption of the BRB and vascular leakage. In conclusion, the topical administration of GLP-1 reverts the impairment of the NVU by modulating essential pathways involved in the development of DR. These beneficial effects on the NVU could pave the way for clinical trials addressed to confirm the effectiveness of GLP-1 in early stages of DR.
Dipeptidyl peptidase IV (DPP-IV) inhibitors. It is well-known that GLP-1 is extremely susceptible to the catalytic activity of the enzyme dipeptidyl peptidase IV (DPP-IV), which rapidly degrades GLP-1. Since GLP-1 is produced by the retina and is downregulated by the diabetic milieu, it is reasonable to hypothesize that the inhibition of DPP-IV could enhance GLP-1, thus preserving its autocrine/paracrine neuroprotective effects. In addition, this strategy could also prevent the degradation of serum-derived GLP-1 that reaches the retina through the blood–retinal barrier. This hypothesis has recently been confirmed in db/db mice treated with eye drops of sitagliptin and saxagliptin (two DPP-IV inhibitors) [ ]. The results, in terms of preventing neurodegeneration, were similar to those obtained by using GLP-1RAs and revealed that topical administration of either saxagliptin or sitagliptin induced an increase in the retinal content of GLP-1. In addition, both DPP-IV inhibitors were able to significantly increase the levels of exchange protein activated by cAMP-1 (EPAC-1) [ ]. This protein is a downstream cAMP signaling mediator and plays an important role in the maintenance of the endothelial barrier and neuronal functions.
Although the main actions of DPP-IV inhibitors seem mediated by an increase in retinal GLP-1 levels, the simultaneous activation of other mechanisms unrelated to GLP-1/GLP-1R cannot be ruled out. In addition, DPP-IV inhibitors are cheaper and more stable than GLP-1. Taken together these characteristics might be perceived as advantages over GLP-1RAs when planning a clinical development.
Neurotransmitters. Diabetes reduces the retinal content of several presynaptic proteins critical to the exocytosis of neurotransmitters and synaptic maintenance [ ], as well as proteins involved in axonal transport [ ]. Therefore, a replacement treatment or therapeutic strategies addressed to avoid the downregulation these neurotransmitters induced by diabetes would exert a beneficial effect. However, further research in this field is needed.
5.2.2
Blocking endothelin-1
ET-1 and its receptors (ETA-R and ETB-R) are upregulated in ESDR [ , ]. The activation of ETA-R mainly mediates vasoconstriction and vasoregression [ ], while the activation of ETB-R induces retinal neurodegeneration [ , ]. Topical (eye drops) administration of Bosentan, by blocking ETA-R and ETB-R, exerted a beneficial effect on both the microvasculature and neurons and prevented retinal neurodegeneration and vascular leakage in db/db mice [ ]. These promising results suggest that therapeutic strategies based on blocking ET-1 should be tested in clinical trials.
5.2.3
Targeting inflammation
Treatments based on targeting specific pro-inflammatory cytokines such as TNFα [ ], IL-1β [ ], or the stimulation of suppressors of cytokine signaling (SOCS) [ ] have had successful results in experimental research. In addition, the administration of general anti-inflammatory treatments, such as corticosteroids or nonsteroid anti-inflammatory drugs (NEAIDs) by topical route, have been useful for treating DME in humans [ , ]. However, a lack of effect in reducing retinal thickness after 1 year of the topical administration of nepafenac has also been reported in patients with non-central-involved DME [ ]. The systemic administration of aspirin and salicilates and the inhibition of several mediators involved in the inflammatory response, such as COX-2 and NFkB, have proved useful in reducing the retinal microvascular abnormalities induced by diabetes in experimental models [ ]. However, clinical evidence for the effectiveness of anti-inflammatory treatment either through eye drops or systemic administration is lacking, and, therefore, further research on this issue is required.
6
Concluding remarks
Neurovascular unit impairment plays an important role in the pathogenesis of DR and is a main therapeutic target in the early stages of DR. Our knowledge of the underlying mechanisms linking diabetes-induced retinal neurodegeneration with microvascular disease is still limited but is seems that neurodegeneration is not always the apparent primary event in pathogenesis of DR. Therefore, a better phenotyping and stratification of patients with DR is needed. In fact, the assessment of neurodegeneration and/or neurodysfunction is now emerging as an essential determinant, besides microvascular impairment, of the final visual outcome of patients with diabetes [ ]. At present, we have several methods for the assessment of neurodysfunction (i.e., ERG, microperimetry) or for measuring neurodegeneration (i.e., retinal thinning assessed by SD-OCT). All these methods seem appropriate nor only for identifying those patients in whom neurodegeneration play a relevant role but also for monitoring the effects of new treatment approaches in the early stages of DR ( Fig. 8.4 ). However, standardization and cost-effectiveness studies are still required before their widespread use in clinical practice can be recommended.
