Respiratory Diseases: Aeromedical Implications
Jeb S. Pickard
Gary W. Gray
And the Lord God formed man of the dust of the ground, and breathed into his nostrils the breath of life … Genesis 2:7.
With their elastic support structure, the lungs are the organ most susceptible to distortion and damage from radial accelerative forces, and have been described as the “Achilles’ heel” of man in the gravito-inertial environment (1). The primary function of the lungs is gas exchange, the efficiency of which depends on appropriate matching of inspired gas with pulmonary perfusion. The distribution of both ventilation and perfusion is affected by gravitational and radial accelerative forces even in healthy lungs. Life support equipment for new generation agile fighters incorporates positive pressure breathing (PPB) to increase G tolerance [positive pressure breathing for G protection (PPG)], and to maintain alveolar oxygen tension during decompression (PPB), adding additional stress to pulmonary tissue. Along with the skin, the lungs form the primary interface to the gaseous and particulate environment, and are susceptible to acute and long-term damage from environmental contamination, an increasing concern as space travel extends from low earth orbit to lunar and interplanetary destinations.
Diseases which affect respiratory structure and function increase the susceptibility to damage and dysfunction in the aviation environment, increasing the aeromedical concern with respect to acute incapacitation, hypoxia, acceleration atelectasis, and potential compromise of G tolerance (see also Chapter 4). In the high-G environment of military fighter operations, marked ventilation-perfusion ([V with dot above]/[Q with dot above]) mismatching occurs due to both gravitational and local/regional factors with resultant impairment of gas exchange. Small airway closure occurs even in the tidal breathing range and, with high-inspired oxygen tensions, can lead to rapid absorption distal to closed airways resulting in “acceleration atelectasis.” Diseases which affect airway function (primarily obstructive lung disease—asthma, chronic bronchitis, and emphysema) are likely to aggravate [V with dot above]/[Q with dot above] mismatching, further compromising gas exchange, potentially reducing G tolerance and increasing susceptibility to acceleration atelectasis. Because of the ubiquitous potential for hypoxia in the aviation environment, aggravation of hypoxia with resultant performance decrements or incapacitation is a more likely consequence of respiratory disease. Respiratory diseases may aggravate the risk and magnitude of hypoxia.
With the transition of space initiatives to lunar and in the longer term, interplanetary travel, the lungs may again become the “Achilles heel”. The lung is the primary filter for lunar dust and other particulate matter to which space travelers are likely to be exposed. The physical, chemical, and potentially even biological properties of lunar and planetary dust are still largely unknown (see Chapter 10).
In this chapter, we will discuss the aeromedical implications of obstructive lung diseases including asthma and chronic obstructive pulmonary disease (COPD), pulmonary cysts and bullae, pneumothorax, and interstitial/restrictive lung diseases including sarcoidosis. Because the dominant sleep disorder is obstructive sleep apnea (OSA), sleep medicine is commonly managed by pulmonary specialists, and therefore key clinical sleep disorders will also be reviewed here. Sleep physiology, fatigue countermeasures, and circadian adaptation are discussed in Chapter 11 and Chapter 23.
ASSESSING AIRCREW WITH RESPIRATORY DISEASE
The standard assessment of individuals with respiratory problems should include pulmonary function testing and lung imaging. The assessment is geared toward defining the risk for hypoxia and for incapacitation due to respiratory distress. Much information may be derived from readily available, well standardized, and relatively noninvasive tests. The evaluation of sleep disorders, on the other hand, is more elaborate and time-consuming, utilizing diagnostic procedures that are not as well standardized across different laboratories.
Pulmonary Function Testing
Although a variety of esoteric tests can be performed in a fully equipped pulmonary function laboratory, a respiratory evaluation almost always begins with the forced vital capacity (FVC) maneuver, which is routinely, though somewhat imprecisely referred to as spirometry. When properly performed, spirometry can provide information about restrictive and obstructive diseases; a normal FVC essentially rules out restriction, while a reduced 1 second forced expiratory volume (FEV1) expressed as a fraction of FVC (FEV1/FVC) establishes the presence of obstruction. Obstruction may have a variety of causes, including the normal effects of aging, but repetition of the test may provide further information. A significant change in FEV1 after administration of a bronchodilator, or after an interval of time, establishes reversible obstruction, which, in the absence of another identifiable cause, strongly suggests asthma.
A low FVC may be caused by a restrictive or obstructive process (due to air trapping, in the latter instance), and should be evaluated by lung volumes. Gas dilution methods are commonly available, but as with spirometry, air trapping can give rise to falsely reduced measurements of volume. Whole-body plethysmography is a far more accurate method for measuring lung volumes. The evaluation of restrictive disease often includes diffusing capacity (or transfer factor), which is complementary to arterial blood gas (ABG) analysis in evaluating [V with dot above]/[Q with dot above] mismatching. However, diffusing capacity is sensitive to a number of confounding variables, and reproducibility is often problematic.
In an aviator, identification of chronic lung disease involving the lung parenchyma should prompt consideration of ABG measurement. ABG should also be measured in any individual with COPD. (Blood gas analysis is rarely indicated in an asthmatic because, except during a serious exacerbation, arterial saturation will usually be normal in all but the most severe asthmatics.) Aircraft cabin altitude is typically restricted to a maximum of 8,000 ft (2,438 m) for civilian aircraft, although certain military aircraft routinely maintain a cabin altitude of 10,000 ft (3,048 m). At those altitudes, an adult with normal cardiorespiratory status will have a resting arterial partial pressure of oxygen of approximately 60 mm Hg. With preexisting lung disease, the arterial partial pressure of oxygen may be further decreased. Measuring peripheral saturation by oximetry is not a satisfactory substitute for assessing oxygenation, because saturation does not appreciably fall until arterial partial pressure drops below 60 mm Hg. Affected aircrew with mild or moderate disease severity will usually be on the relatively flat portion of the oxyhemoglobin dissociation curve (see Figure 2.4).
The list of restrictive diagnoses outnumbers obstructive diseases, but obstructive disease is more commonly encountered in practice, and one disease in particular accounts for most pulmonary problems in aviation. The identification of obstruction on spirometry that shows a significant (>15%) improvement after a bronchodilator challenge is strong evidence for asthma, but asthma is a variable disease, and may not be evident at the time spirometry is performed. Where asthma is suspected despite normal spirometry, bronchial provocation testing (BPT) can be employed. A variety of provocative agents have been utilized in the laboratory, of which nebulized histamine and particularly methacholine are the best standardized. With methacholine challenge, the usual parameter measured is the PC20, the concentration of methacholine required to provoke a 20% reduction in FEV1. A PC20 of less than 8 mg/mL has typically been considered positive. Alternatively, current American Thoracic Society guidelines recognize a borderline category (2). Under this classification, individuals with a PC20 equal to or greater than 16 mg/mL are considered normal, whereas the development of bronchospasm at concentrations lower than 4 mg/mL is considered hyperreactivity. Those who show a 20% drop in FEV1 at concentrations between these limits are considered to be borderline. Although it is most often employed as a diagnostic maneuver in patients presenting in atypical manner, methacholine challenge testing can also be used to assess response to therapy. Because of the time and expense involved, the latter indication has not found wide acceptance in clinical practice, but demonstrating suppression of reactivity in response to treatment can be very useful in aircrew.
A few caveats are in order. BPT is not useful for screening; the prevalence of airway hyperreactivity considerably exceeds that of clinical asthma, and will fluctuate with other factors such as recent respiratory infections. The results of BPT must be integrated with the clinical picture. Also, methacholine challenge testing is not useful for ruling out subsequent relapse in remitted childhood asthmatics. In one recent study, approximately a third of those with negative bronchoprovocation tests subsequently relapsed (3). This is equivalent to the historical rate of relapse of remitted childhood asthma.
Imaging
The chest x-ray is indispensable in the evaluation of respiratory disease. The reason is simple enough; for many diseases, the lungs provide their own contrast medium. At full inspiration, the chest cavity is predominantly filled with air, and disease processes such as edema, inflammation, neoplasia, and fibrosis are associated with water density. The standard chest film is much less useful in the evaluation of obstructive diseases. The disruption of alveolar membranes typical of emphysema and the narrowing of endobronchial lumina seen in bronchitis and asthma do not appreciably affect the radiodensity of the lung.
Computed tomography (CT) of the chest significantly increases resolution, and better delineates adenopathy, early fibrosis, small nodules, and numerous other abnormalities. It provides much clearer imaging of bullae than does routine chest x-ray, and the use of a high-resolution format also allows the identification of subpleural blebs, something not possible with a standard roentgenogram.
Sleep Studies
Accurate diagnosis of most clinical sleep disorders requires overnight polysomnography (PSG). Although a full night’s
sleep study in a sleep disorders laboratory is cumbersome, simpler methods sacrifice too much information. Typically, PSG will include electroencephalography (two to three leads), electromyography (genioglossus and anterior tibialis), electrooculography, electrocardiography (usually lead II), and oximetry. Airflow and respiratory effort are the two respiratory parameters most commonly measured.
sleep study in a sleep disorders laboratory is cumbersome, simpler methods sacrifice too much information. Typically, PSG will include electroencephalography (two to three leads), electromyography (genioglossus and anterior tibialis), electrooculography, electrocardiography (usually lead II), and oximetry. Airflow and respiratory effort are the two respiratory parameters most commonly measured.
Staging of sleep is reasonably standardized, because most laboratories use the Rechtschaffen and Kales scoring system. Unfortunately, there is considerable variation in the definition of respiratory events, especially hypopneas. Similarly, the definition of what constitutes an arousal is inconsistent from laboratory to laboratory. Scoring of sleep apnea is predominantly based on the apnea-hypopnea index (AHI), which measures the average number of sleep-disordered breathing events per hour. Up to 5 events per hour are considered to be normal, whereas more than 30 events per hour are generally classified as severe, though that classification is also variable.
Multiple sleep latency testing (MSLT) is the most commonly used objective test to quantify daytime sleepiness. Typically performed on the day following PSG, it consists of a total of four or five monitored 20-minute naps at 2-hour intervals, with sleep latency defined as the interval between “lights-out” and the first 30 seconds of scorable sleep, and rapid eye movement (REM) latency as the interval from the beginning of the first epoch scored as sleep to the first epoch of REM sleep. Normal sleep latency is greater than 10 minutes, whereas less than 5 minutes is considered pathologic sleepiness. Normally, REM sleep is not seen during any trial; two or more episodes of sleep-onset REM suggest the diagnosis of narcolepsy.
While MSLT measures the tendency to fall asleep, maintenance of wakefulness testing (MWT) measures the ability to stay awake. The two propensities tend to be inversely correlated, and yet they are distinct, with some subjects showing disparate results on the same day. MWT is performed with the individual instructed to try to stay awake while sitting in a darkened, quiet room. The test consists of four 20- or 40-minute trials performed at 2-hour intervals. Normal latency is 18 minutes, with a lower limit of 11 minutes. MWT is assumed to be more occupationally relevant than MSLT, because it more nearly addresses the issue of concern in the occupational setting. It should be noted, however, that the MWT was validated in subjects who did not have a particular interest in the outcome.
ASTHMA
Asthma is an inflammatory disease of airways in response to various stimuli characterized by paroxysmal or persistent symptoms, such as dyspnea, chest tightness, wheezing, sputum production and cough associated with airway hyperresponsiveness, and variable airflow limitation. The prevalence of asthma is increasing in the industrialized world. The overall prevalence of lifetime asthma in the United States is 11.9% (4). The severity of asthma has also increased, with the death rate due to asthma doubling in the period 1980 to 1999 (5). Asthma is most common in childhood. Remission of childhood asthma may occur, with up to 25% remaining free of symptoms from adolescence onward. Most adult asthmatics have a history of childhood asthma, and positive markers of airway inflammation may be present in the absence of clinical symptoms or pulmonary function abnormalities.
The aeromedical concerns with respect to asthma include the potential for sudden incapacitation with acute attacks (e.g., with a cockpit smoke situation), small airways dysfunction causing [V with dot above]/[Q with dot above] mismatching thereby magnifying hypoxia, acceleration atelectasis and lowered G tolerance, the potential for pulmonary barotrauma with acute decompression, and adverse effects of medications. In the operational military environment, constraints on wearing protective equipment and deployability issues are also concerns.
Asthma represents a spectrum of disease severity and the challenge for the flight surgeon/aeromedical practitioner is to quantify disease severity and to generate an appropriate aeromedical disposition based on an evidence-based risk assessment. The assessment should include detailed history, physical examination, and a pulmonary function assessment.
Evaluation of Asthma in Aircrew
History
Important in the history are careful documentation of the severity of past episodes including requirement for urgent medical care and/or hospitalization, particularly in the last several years. The medications required for acute episodes and for maintenance therapy reflect the disease severity. The use of short-acting β agonists (SABAs) more than three times a week other than for physical activity indicates inadequate control of asthma. The best predictor of severe asthmatic attacks is a history of such episodes.
Although asthma may arise de novo in adults, most adults with asthma have a history of childhood symptoms of asthma or recurrent bronchitis. Asthmatic children whose asthma remits permanently will almost all be free of symptoms by adolescence. The persistence of asthmatic symptoms into adolescence is of prognostic significance for adult asthma. Asthma symptoms may remit for a period of years, only to return.
Identification of trigger factors is important by providing potential options for nonpharmacologic intervention. These may include environmental allergens including animal dander (cats in particular), dust mites (e.g., in feather pillows and bedding), and pollens such as ragweed. Other common triggers include intercurrent viral infections, occupational triggers, exercise, and cold air. Up to 20% of asthmatics may exhibit aspirin sensitivity. Cigarette smoke is a nonspecific bronchial irritant which can complicate all types of asthma. Asthmatics may demonstrate exercise-induced bronchospasm (EIB), which is of particular concern in fast jet aircrew where strenuous exertion with anti-G straining maneuvers combined with PPB may induce bronchospasm.
Asthmatic episodes may occur in several patterns after exposure to allergens. The immediate response typically occurs within a few minutes of exposure and lasts 30 to 60 minutes, similar to the response after methacholine or exercise. Early responses may be prevented with sodium cromoglycate and generally respond well to inhaled short acting bronchodilators. Late asthmatic responses occur 6 to 8 hours after the inhaled challenge, are typically more severe, and resolve more slowly over 12 to 24 hours. Late reactions respond much more poorly to inhaled bronchodilators. Documentation of delayed or late asthmatic responses is of aeromedical significance. Nocturnal asthma (awakening at night with asthmatic symptoms) usually signifies heightened disease activity.
Careful documentation of the history from childhood onward provides crucial information for appropriate aeromedical disposition. In the case of candidates for aircrew training or licensure, minimizing past symptoms is common and historical information should be corroborated with additional data, for example, from family physicians.
Pulmonary Function Assessment
Pulmonary function testing provides objective data on lung function and airway reactivity. Unfortunately, there is no test specific for asthma, and during clinical remission asthmatics may have normal or near-normal pulmonary function. Assessment should include inspiratory and expiratory flows, lung volumes, airway resistance, and BPT. In individuals with histories suggesting exercise-induced asthma, exercise testing with airflow assessment before and after exercise may be helpful.
Flow-volume loops will generally show evidence of expiratory airflow limitation, although as mentioned, during clinical remission, some asthmatics may not have airflow limitation. Occasionally, inspiratory airflow limitation may be discovered as a manifestation of tracheomalacia.
Lung volume assessment may show evidence of gas trapping with increased residual volume, functional residual capacity (FRC) and/or total lung volume (see Chapter 2). Body plethysmographic lung volumes greater than helium dilution also suggest gas trapping, reflected in an FRC measured by the body box as 10% greater than the FRC measured by helium dilution.
FVC maneuver and lung volumes should be repeated after administration of a bronchodilator. An increase in flow rates suggests increased bronchomotor tone, and a significant decrease in lung volumes is an indicator of gas trapping. A 12% increase in FEV1 (at least 180 mL) from baseline 15 minutes after inhalation of a β agonist is considered significant and an indication of asthma. BPT provides objective information about airway reactivity and should be performed as part of the aeromedical assessment of aircrew being evaluated for asthma. However, the prevalence of bronchial hyperreactivity far exceeds that of clinically manifest asthma, and some persons with hyperreactive airways do not have asthma. Additionally, a negative BPT, while indicating a clinical remission, does not predict long-term resolution of asthma. The results of BPT should be integrated with the clinical picture.
Even during clinical remission, asthmatics may have ongoing airway inflammation. Individuals whose asthma is triggered by inhaled allergens usually have atopic disease. Atopic individuals may show peripheral blood eosinophilia, increased immunoglobulin E (IgE) levels, and positive wheal-and-flare reactions to intradermal testing. Markers of airway inflammation include sputum eosinophils and increased levels of exhaled nitric oxide (eNO). eNO assessment is a noninvasive test that is a measure of airway inflammation. When available, elevated eNO levels documented with standardized testing reflect ongoing airway inflammation, even in the absence of clinical symptoms or pulmonary function test (PFT) abnormalities. Such testing may provide additional corroborative information in assessing aircrew for asthma.
In individuals with a history suggestive of EIB, an exercise test with flow-volume curves before and after a maximum exercise stress test may be helpful. A fall in FEV1 greater than 12% within the first 30 minutes of exercise is considered significant.
Treatment of Asthma in Aircrew
Treatment of asthma in aircrew should follow established clinical guidelines (see Recommended Readings). Treatment considerations in aircrew should include avoidance of environmental allergens. Immunotherapy is generally not recommended in the treatment of asthma. Smoking cessation is an important therapeutic intervention and should be considered a requirement for continued aircrew duties.
SABAs are used for short-term control but may cause significant adrenergic side effects, and are not recommended before flight duties. Except for preexercise prophylaxis in aircrew with EIB, the requirement for SABAs on more than a very infrequent basis (e.g., in association with an intercurrent respiratory infection) suggests inadequate asthma control. Inhaled corticosteroids (ICS) attack the primary inflammatory mechanism of the asthmatic response, and should be considered early in the control of asthma for aircrew. The goal is to obtain full remission with inhaled steroids and to taper the dose to that required to maintain full control as assessed by history, self-monitored peak flow rates, and by repeat pulmonary function testing including normal bronchial provocation assessment. ICS are safe from an aeromedical perspective, and do not require flying restrictions. For asthmatic aircrew in whom good control is not achieved with maximum recommended doses of inhaled steroids, additional treatment with a long-acting β agonist (LABA) and/or a leukotriene inhibitor may be considered. Asthma requiring further intervention such as oral steroids or methotrexate is not considered compatible with aircrew duties.
Aeromedical Disposition
Aircrew Candidates
Because of the considerable expense associated with aircrew training, and potential adverse effects of even mild degrees of airways dysfunction in the high-G environment, a history of asthma is generally considered disqualifying for military aircrew selection. However, because of the increased tendency to label as asthmatic those children with a mild degree of wheezing from an intercurrent viral infection, a careful history is important, including data obtained from the pediatrician or family physician. For candidates with a history of mild childhood asthma with no requirement for urgent care or hospitalization following puberty, a full pulmonary function assessment including bronchial challenge testing should be carried out. If available, a standardized eNO test may provide further objective information about airway inflammation. Candidates with no clinical history of asthma following puberty, and normal pulmonary function assessment including normal bronchial reactivity may be carefully considered for aircrew training.
Experienced Aircrew
Aeromedical disposition for experienced aircrew with asthmatic symptoms will depend on their aircrew duties or type of license, results of the assessment as outlined, and medications used for control of their symptoms. Generally, because of the possibility of persistent small airways dysfunction even in the absence of symptoms, asthma is generally disqualifying for fast jet aircrew. Non-fast jet military or civilian aircrew, whose asthmatic symptoms are mild and are well controlled with inhaled steroids alone, and who have normal or near normal pulmonary function assessment including bronchial reactivity may be fit for continued aircrew duties. A normal eNO assessment also reflects good control of asthmatic inflammation. Such aircrew are unlikely to have an acute episode, even if medication is unavailable for several weeks. Additional requirement for a LABA or leukotriene inhibitor suggests a more severe degree of asthma, but may be compatible with some aircrew duties. In such individuals, there is an increased risk of developing increasing asthmatic symptoms in a shorter period without medications. Apart from preexercise prophylaxis, and the occasional exacerbation related to a viral infection, the use of SABAs is generally a reflection of poorly controlled asthma and is disqualifying for aircrew duties.
CHRONIC OBSTRUCTIVE PULMONARY DISEASE
COPDs occur less commonly in aircrew than asthma, primarily because the time course for development of clinically significant COPD spans the normal aircrew career. The Global Initiative for Chronic Obstructive Lung Disease (GOLD) defines five stages of COPD (see Recommended Readings). Except in unusual circumstances, COPD in aircrew would be anticipated to be GOLD stage I (mild). Clinically manifest disease (GOLD stages II-IV) would be expected only in the latter years of a military or commercial flying career or in retirement. However, since the pathophysiologic processes that lead to COPD are active during the flying years, aeromedical physicians are in an ideal position for primary prevention interventions, which may ameliorate these processes and prevent the development of COPD.
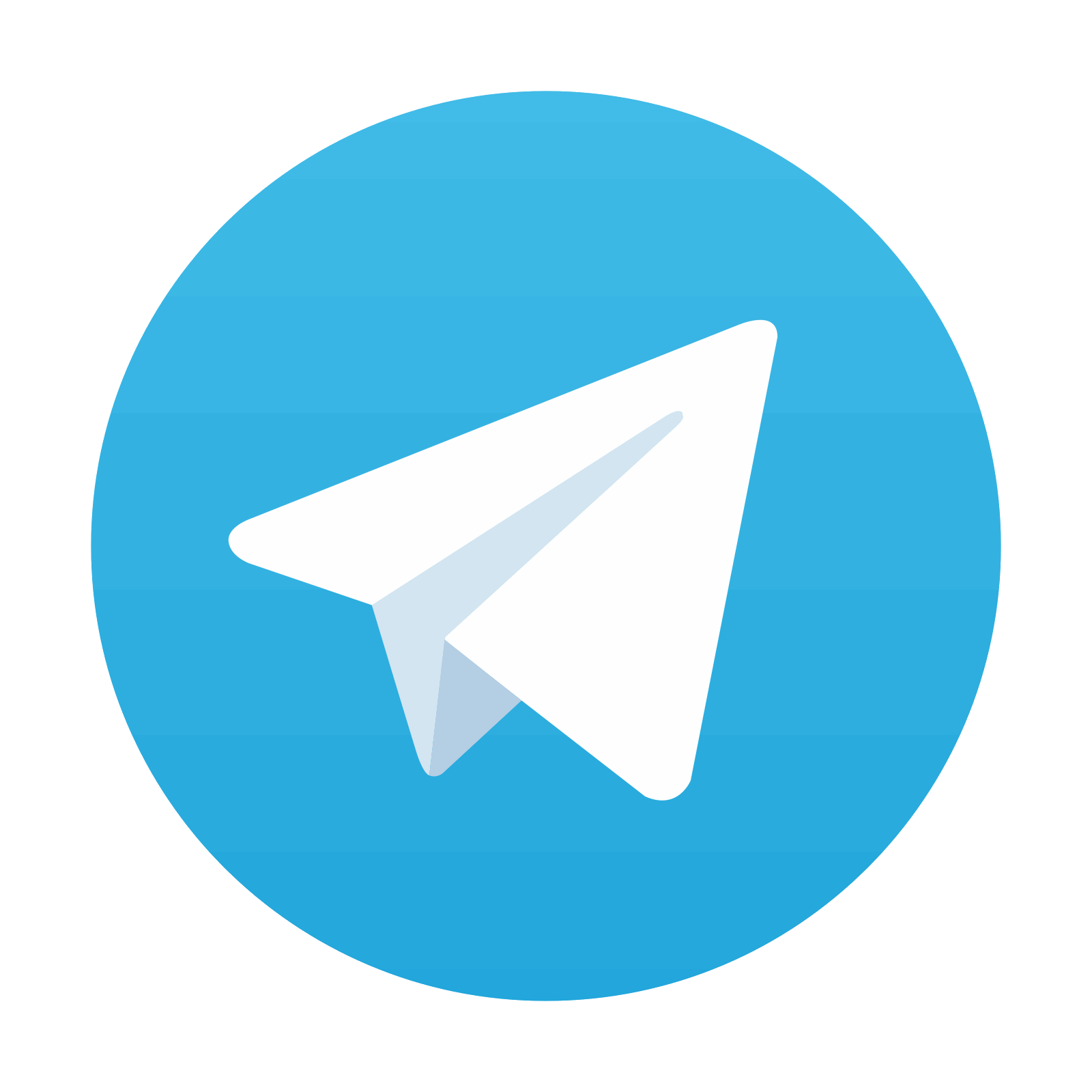
Stay updated, free articles. Join our Telegram channel

Full access? Get Clinical Tree
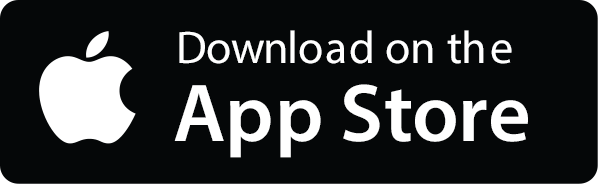
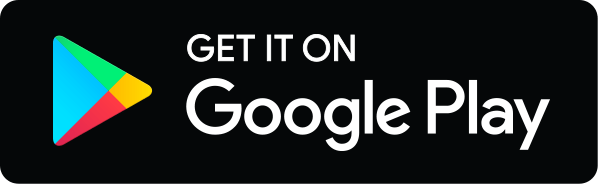