The titre of normal autoagglutinins at 0–2°C does not usually exceed 64 using a tube technique with a 2% cell suspension and reading the results microscopically (Dacie 1962, p. 460), but is much higher with more sensitive methods, or when using microplates. In about one in four cases the titre of normal cold autoagglutinins is enhanced two- to four-fold if the serum is titrated in 22% bovine serum albumin instead of saline (Haynes and Chaplin 1971).
Normal cold autoagglutinins almost always have the specificity anti-I (Tippett et al. 1960) but, occasionally, may have other specificities: a mixture of anti-I and anti-i (Jackson et al. 1968); anti-IT (Booth et al. 1966); anti-Pr (Garratty et al. 1973; Roelcke and Kreft 1984); anti-A, -B, -A1I and -BI (for references, see seventh edition, p. 292); anti-M and -N (Moores et al. 1970; Tegoli et al. 1970; Sacher et al. 1989). Anti-LW occurring as a cold autoantibody is described in Chapter 5.
Anti-i was found in 10 out of 47 patients with cirrhosis of the liver, but active only at low temperatures and with a titre of 32 or less in 7 out of the 10 cases (Rubin and Solomon 1967).
Anti-I cold agglutinins can usually be demonstrated in cord blood (second edition, p. 252). They are IgM and presumed to be synthesized by the fetus in utero (Adinolfi 1965a).
An exceptional high-titre cold agglutinin that agglutinated cells at 37°C, but which was not associated with red cell destruction in vivo, has been described (Sniecinski et al. 1988).
Autoagglutinins Inhibited by Ionized Calcium
Some examples of cold autoagglutinins react only in the absence of ionized calcium. The first example was described by Parish and Macfarlane (1941) as an autoagglutinin reacting in citrate but not in saline. Many examples have since been published, most with anti-HI or anti-H specificity. In all cases agglutination has been inhibited by Ca2+ and has depended on the presence of citrate or EDTA (for references, see previous editions of this book). Yasuda and colleagues (1997) describe an auto-anti-B whose reactivity depended on the concentration of ionized calcium being inhibited in concentrations of calcium chloride above 0.5 mM.
An autoagglutinin demonstrable only against borate-suspended red cells, with anti-A specificity, has been described (Strange and Cross 1981).
An autoagglutinin enhanced by sodium azide, with anti-I specificity, has been reported (Reviron et al. 1984).
Normal incomplete cold ‘antibody’ (n.i.c. antibody), which binds complement to red cells at low temperatures (Dacie 1950; Dacie et al. 1957), has anti-H specificity (Crawford et al. 1953) but is not an immunoglobulin (Adinolfi et al. 1963) and, in its properties, has some resemblance to properdin (Adinolfi 1965b).
Harmful Cold Autoantibodies (see Table 7.1)
By definition, harmful cold autoantibodies are those associated with increased red cell destruction and/or vascular occlusion on exposure to cold. Autoimmune haemolytic anaemia (AIHA) is less commonly associated with cold autoantibodies than with warm ones. In several published series, each of more than 100 cases of AIHA, about 15–20% have been due to cold autoantibodies (Dausset and Colombani 1959; Dacie 1962; van Loghem et al. 1963; Petz and Garratty 1975). A similar percentage (16%) was found in a series of 2000 patients with red cell autoantibodies (Engelfriet et al. 1982). A slightly higher percentage (about 35%) was observed in two other series (Vroclans-Deiminas and Boivin 1980; Sokol et al. 1981).
Harmful cold autoantibodies may be (1) cold autoagglutinins and haemolysins or (2) biphasic haemolysins (Donath–Landsteiner antibodies).
Cold Haemagglutinin Disease with Autoimmune Haemolytic Anaemia
Two clinical syndromes may be distinguished, one chronic and one transient (reviewed by Petz 2008). In both, the pathological effects of the autoantibodies – vascular occlusion and accelerated red cell destruction – are exacerbated when the patient is exposed to cold. Vascular occlusion is seen particularly in the exposed parts of the body; accelerated red cell destruction may lead to haemoglobinuria.
The chronic syndromes are nearly always, if not always, associated with IgM paraproteins (monoclonal) with cold agglutinin activity. Only a small proportion of IgM paraproteins have cold agglutinin activity, for example 11 out of 99 in the series of Pruzanski and co-workers (1974). Of patients in whose serum IgM paraproteins are found, the majority have chronic lymphocytic leukaemia or some form of lymphoma and a minority have so-called Waldenström’s macroglobulinaemia (Mackenzie and Fudenberg 1972); intermediate disease states are not uncommon (Tubbs et al. 1976). In some of the patients the paraproteinaemia is of the benign kind. Sometimes the cold autoagglutinins are detected before the paraproteinaemia becomes manifest.
In the transient syndromes, the cold haemagglutinins are polyclonal. The syndromes occur following infectious diseases, particularly mycoplasma infection and, less commonly, infectious mononucleosis. When the antibodies are active at 30°C or higher there may be an associated immune haemolytic anaemia.
In children, following infectious disease, high-titre cold agglutinins are only rarely observed (Habibi et al. 1974). A striking inverse correlation has been found between the titre of IgG anti-F(ab′)2 antibodies and the titre of anti-I cold agglutinins in cold haemagglutinin disease (CHAD) not associated with infection (P < 0.0001) but not in CHAD associated with infection. An important role of IgG anti-F(ab′)2 in the regulation of the production of cold anti-anti-I in patients with CHAD unassociated with infection is postulated (Terness et al. 1995).
Thermal Range of Autoantibody
Some examples of pathological cold autoagglutinins have titres of 1 × 106 or more at 0–4°C. At higher temperatures they are markedly less active and often will not agglutinate red cells in vitro above a temperature of about 31°C (Dacie 1962, p. 468). Less commonly, the titre at low temperatures is only moderately increased but the antibody has a very wide thermal range and may then cause moderately severe anaemia (Schreiber et al. 1977; Rousey and Smith 1990). It should be emphasized that the clinical significance of a cold antibody is determined entirely by its ability to combine with red cells at, or near, body temperature rather than by its titre at some lower temperature.
Factors Enhancing Agglutination
In many cases the titre of cold autoagglutinins is enhanced by using an albumin solution rather than saline as a medium; 22% albumin is distinctly better than 12% (Haynes and Chaplin 1971).
In testing 28 examples of anti-I associated with AIHA, at 30°C 14 failed to agglutinate cells suspended in saline but all 28 agglutinated them in albumin. In tests at 37°C, only two examples agglutinated cells suspended in saline, but 19 agglutinated cells suspended in albumin (Garratty et al. 1977). In some cases it is necessary to use an increased ratio of serum to cells to demonstrate clinically significant cold autoantibodies.
At any given temperature, enzyme-treated red cells take up more cold autoantibody than untreated cells and are agglutinated by potent anti-I up to about 38–40°C (Evans et al. 1965).
In studies with 131I-labelled potent cold autoagglutinins maximally sensitized cells took up 8.9 mg of antibody/ml red cells, corresponding to rather more than 500 000 molecules per cell (Evans et al. 1965). The same authors found that the rate of association and dissociation of anti-I was unaffected by the presence of complement, suggesting that complement did not affect the binding of this antibody. On the other hand, Rosse and co-workers (1968), using the C1 transfer test, found that complement did affect the binding of some examples of anti-I. For example, in the presence of C1, fewer red cells were required to absorb the same amount of antibody (for further discussion see Chapter 3).
Complement Binding
When normal red cells are sensitized in vitro with fresh serum containing anti-I some cells may be lysed (see below); unlysed cells react with anti-C3c and anti-C4c as well as with anti-C3g, anti-C3d and anti-C4d. On circulating red cells the only C3 and C4 components detectable are C3d and C3g (Lachmann et al. 1982; Voak et al. 1983), and C4d (and, possibly, C4g). Serum containing potent autoagglutinins is always capable of producing some lysis of normal red cells at 20°C, although it may be necessary to adjust the pH of the serum to 6.8 to produce this effect (Dacie 1962, p. 468). Potent cold autoagglutinins that are readily lytic may be confused with biphasic haemolysin (Donath–Landsteiner antibody), but the latter antibody is non-agglutinating and almost always has anti-P specificity.
When the possibility of confusion between anti-I and biphasic haemolysin arises, the following comparison is useful in distinguishing between them (H Chaplin, personal communication): in one test, two samples of serum, one untreated and one acidified to pH 6.8, are incubated continuously (with red cells) at 20–25°C for 1 h and in the other, serum and red cells are first kept at 0°C for 30 min, then at 37°C for 30 min. Biphasic haemolysin gives maximal lysis under these latter conditions and is unaffected by acidification; on the other hand anti-I haemolysins are maximally lytic when incubated continuously at 20–25°C, especially with acidified serum.
At low temperatures the agglutination caused by anti-I is very intense and when agglutination is dispersed some lysis may be observed due, apparently, to mechanical damage during dispersal of the agglutinates (Stats 1954). Although it has been claimed that potent cold autoagglutinins directly lyse red cells without the aid of complement (Salama et al. 1988), the effect may be a laboratory artefact.
Acquired Resistance to Complement-Mediated Lysis
When normal red cells are incubated at 20–30°C with anti-I serum and then warmed to 37°C, anti-I is eluted but complement components remain bound to the red cell surface (Harboe 1964; Evans et al. 1965). As described in Chapter 3, bound C3b is very rapidly converted to iC3b, which is then cleaved relatively slowly, leaving only C3dg on the cell surface. Normal red cells exposed to anti-I and complement in vitro under conditions that are suboptimal for producing lysis become coated with α2D (i.e. C3dg) and become resistant to lysis (Evans et al. 1968; De Wit and van Gastel 1970) and take up little or no β1A (i.e. C3b) upon renewed exposure to anti-I and complement (Engelfriet et al. 1972; see also Jaffe et al. 1976).
Red cells made resistant to lysis by anti-I in the way just described are partially protected against lysis by anti-Leb (Engelfriet et al. 1972) and by anti-Lea (M Contreras and PL Mollison, unpublished observations). These findings are explained, presumably, by the fact that I and Lewis antigen sites are on molecules in close proximity and thus bring about the accumulation of C3dg molecules on closely similar areas of the red cell membrane. Cells coated with complement by exposure to serum at very low ionic strength are not protected against lysis by anti-I (De Wit and van Gastel 1970), possibly because the C3dg molecules are dispersed over the red cell surface and thus present in too low concentrations in the critical areas round I sites.
Circulating red cells of patients with CHAD are strongly coated with C3dg and are relatively resistant to red cell destruction. Thus, if a sample of red cells from a patient with CHAD is labelled with 51Cr and re-injected into the circulation, the rate of red cell destruction is uniform and relatively slow. On the other hand, when red cells from a normal donor are injected, some 50% are destroyed in the first hour, although subsequently the rate of destruction is far slower (Evans et al. 1968); see below and also Chapter 10.
The question of transfusion in CHAD is discussed below.
Specificity of Cold Autoagglutinins Associated with Autoimmune Haemolytic Anaemia
Ii
In patients with CHAD the antibodies most commonly have I specificity, reacting with determinants on branched type 2 chains and occasionally have i specificity, reacting with determinants on linear type 2 chains (see Chapter 4). Antibodies (anti-j) reacting with both branched and linear type 2 chains have also been described (Roelcke et al. 1994). I specificity can be verified by demonstrating that the serum reacts more strongly with adult than with cord red cells; several samples of cord cells should be tested, as some react relatively well with anti-I. The stronger reaction of anti-I with adult than with cord cells is sometimes more marked at 30°C than at lower temperatures (Burnie 1973). Occasionally, the antibody in CHAD has other specificities, as described below.
Pr and Sa
Unlike I, these antigens are expressed in equal strength on the red cells of adults and newborn infants. Most Pr antigens are destroyed by both proteases and sialidases. Anti-Pr and -Sa recognize the sialo-O-glycans of glycophorins. The reactivity of anti-Pr, as of some examples of anti-I, is greatly enhanced in low-ionic-strength solution (LISS), a fact that must be remembered when interpreting tests performed in this medium.
Anti-Pr has been found in patients, including newborn infants, with rubella (Geisen et al. 1975; König et al. 1992, 2001) and in patients with varicella (Northoff et al. 1987; Herron et al. 1993). Life-threatening autoimmune haemolytic anaemia occurring in a 6-week-old infant 5 days after receiving a diphtheria-pertussis-tetanus vaccination was attributed to an IgM cold-reactive autoantibody with probable anti-Pr specificity (Johnson et al. 2002). The infant survived after receiving transfusions from a donor who was homozygous for the extremely rare Mk phenotype and another individual who was homozygous for the very rare MiVII phenotype.
Sialidase-Susceptible Antigens
Sia-11, Sia-b1 and Sia-lb1 (formerly Vo, F1 and Gd) are differentiation antigens (like I and i), created by sialylation of linear and branched type 2 chains (for a review of the biochemistry and serology of the above-mentioned specificities, see Roelcke 1989).
Other Specificities
Rarely the specificity may be anti-A (Atichartakarn et al. 1985), anti-type 2 H (Uchikawa and Tokyama 1986), anti-P (von dem Borne et al. 1982), anti-M-like (Sangster et al. 1979; Chapman et al. 1982), anti-D (Longster and Johnson 1988), anti-Sdx, later named anti-Rx (Marsh et al. 1980; Bass et al. 1983) or anti-Ju (Göttsche et al. 1990a).
Anti-I and Anti-I Associated with Acute Infections
Following infection with Mycoplasma pneumoniae there is commonly a transient increase in the titre and thermal range of anti-I cold autoagglutinins. When the thermal range is high enough, the patient may develop an episode of haemolytic anaemia, which may be severe.
The fact that the titre of anti-I is increased following infection with M. pneumoniae suggests the possibility of the presence in that organism of I-like antigen. Although intact M. pneumoniae do not inhibit anti-I, lipopolysaccharide prepared from these organisms does. Furthermore, the cold agglutinins that develop in rabbits following the injection of M. pneumoniae are inhibited by these organisms (Costea et al. 1972). The erythrocyte receptors for mycoplasma are long-chain oligosaccharides of sialic acid joined by α (2–3) linkage to the terminal galactose residues of polyN-acetyllactosamine sequences of Ii antigen type (Loomes et al. 1984).
Anti-I in very high titre has been found following infection with Listeria monocytogenes, which carries an I-like antigen: the patient had transient haemolytic anaemia (Korn et al. 1957). A transient increase in anti-I titre has also been described in a patient with systemic leishmaniasis associated with haemolytic anaemia (Kokkini et al. 1984) and following an acute cytomegalovirus infection not clearly associated with haemolytic anaemia (Pien et al. 1974).
In infectious mononucleosis, anti-i is frequently present as a transient phenomenon (Jenkins et al. 1965; Rosenfield et al. 1965). The antibodies rarely react in vitro above 24°C. Fewer than 1% of patients with mononucleosis develop a haemolytic syndrome (Worlledge and Dacie 1969).
In a few cases in which haemolytic anaemia complicated infectious mononucleosis and in which the patient’s serum contained a potent cold agglutinin, the agglutinin was an IgG–IgM complex, the antibody being IgG and the IgM an anti-IgG antibody (Goldberg and Barnett 1967; Gronemeyer et al. 1981). In an exceptional case, the antibody although IgM, behaved serologically as a biphasic haemolysin: the agglutinin was active in vitro only up to 22°C, but exposure of red cells to the serum at 4°C, followed by warming of the mixture to 37°C, resulted in lysis (Burkart and Hsu 1979). In one, apparently unique, case anti-N was formed instead of the expected anti-i in a patient with infectious mononucleosis (Bowman et al. 1974).
Persistent anti-I and anti-i cold agglutinins not associated with haemolytic anaemia have also been found in the serum of patients with acquired immune deficiency syndrome (AIDS) or AIDS-related complex (Pruzanski et al. 1986). The thermal range of the antibodies was not established.
Is It Necessary in Clinical Practice to Determine the Titre of Cold Agglutinins?
The serological diagnosis of CHAD is made by demonstrating the stronger reactivity at low temperatures and the wide thermal range of the antibody; activity can usually be demonstrated in vitro up to a temperature of 31°C. Determination of the titre is not worthwhile as a routine, although an association between a low titre and a response to corticosteroids has been observed (see below).
Red Cell Transfusion in Patients with Cold Haemagglutinin Disease
When red cells from a normal (I-positive) donor are transfused to a patient with CHAD due to anti-I, there is a phase of destruction that lasts until the cells have acquired resistance to complement-mediated destruction; during this phase, a proportion of the transfused population is destroyed within minutes; an identical phenomenon is observed with complement-activating alloantibodies (see Chapter 10). In CHAD, therefore, transfusion should be avoided if possible. If a transfusion is judged to be essential, the blood should be prewarmed, although a more important step is to nurse the patient in a warm room (see below). Red cells from ii adults have been shown to survive normally in patients with CHAD due to anti-I both in the chronic form (van Loghem et al. 1963) and in the transient form (Woll et al. 1974), but ii donors are seldom available. Unless the patient fails to respond to transfusion of red cells from random donors and it is known that ii donors are available, there is no point in determining the specificity of the cold agglutinins.
The presence of cold agglutinins complicates pretransfusion testing (see Chapter 8).
Most patients with CHAD are not severely anaemic; if a haemolytic crisis does develop, red cell destruction can usually be arrested by putting the patient in a really warm environment (40°C). In cases in which the cold autoagglutinins have a low titre but a wide thermal range, treatment with corticosteroids has been successful (Lahav et al. 1989). Treatment with alpha-interferon resulted in a prompt clinical response and a considerable decrease of the titre of the cold agglutinins in two patients with severe CHAD (O’Connor et al. 1989; Fest et al. 1994). The successful use of rituximab, a monoclonal anti-CD20, in the treatment of CHAD is reported by Berentsen and co-workers (2004) who monitored 37 courses of rituximab given prospectively to 27 patients. Fourteen patients responded to their first course of treatment and 6 out of 10 responded to re-treatment. These authors conclude that rituximab is an effective and well-tolerated therapy for CHAD. Schollkopf et al. (2006) report a response to rituximab in 45% patients with CHAD but conclude for most patients the effect is transient.
In a patient with scleroderma associated with severe haemolytic anaemia due to cold agglutinins, treatment with danazol (a gonadotrophin-release inhibitor) was followed by a rapid rise in Hb concentration (Lugassy et al. 1993). Roth et al. (2009) describe successful treatment of an elderly male patient with CHAD with eculizumab, a monoclonal antibody to complement component 5 used for the treatment of PNH. Continuous therapy with eculizumab resulted in improvement in anemia, fatigue and overall quality of life.
Immunoglobulin Structure of Cold Agglutinins
In chronic cold haemagglutin disease most examples of anti-I and anti-i are IgMκ, although a few IgMλ examples have been described (Pruzanski et al. 1974; Roelcke et al. 1974). All cold agglutinins with specificity for the I or i antigens are encoded by a single VH gene segment, VH4–34 (synonym: VH4–21; Silberstein et al. 1991; Pascual et al. 1992). The three-dimensional structure of the Fab of an anti-I (KAU) has been solved (Cauerhff et al. 2000). Potter and co-workers (2002) analysed this structure and proposed that I antigen interacts with antibody KAU via a hydrophobic patch in FR1 and the outside surface of CDRH3 rather than the conventional antigen binding site (Figure 7.1). This raises the intriguing possibility that the conventional binding site of antibody KAU, and by inference of other cold agglutinins directed at I and i antigens, bind to additional as yet unidentified antigens. These authors draw an analogy between the binding of Ii carbohydrate structures to the hydrophobic patch on KAU and the way in which oligosaccharide chains of antibody molecules bind to a hydrophobic patch on the Cγ2 domains of IgGFc. This alternative carbohydrate antigen-binding region also provides a possible explanation for the cold agglutinin activity of other V4–34 encoded antibodies including monoclonal anti-Ds (Thorpe et al. 1998).
Figure 7.1 Crystal structure of cold agglutinin KAU, showing the hydrophobic patch in FR1 in relation to the conventional Ab combining site. (a) Space-filling representation of the surface of the VH and VL domains of KAU (Brookhaven Protein Data Bank code 1DN0). The hydrophobic patch (HP) and the CDRH3 are depicted in black, the main body of the Ab is white, and the rest of the Ag binding site is grey. The arrow indicates the VH:VL axis and the location of the conventional Ag binding site. (b) View almost perpendicular to (a), looking down the axis of the VH:VL domain pair onto the conventional Ag binding site. Colour codes are as in (a). Figures were produced using INSIGHT II.
(Source: Potter et al. 2002. Reproduced with permission of the American Association of Immunologists.)
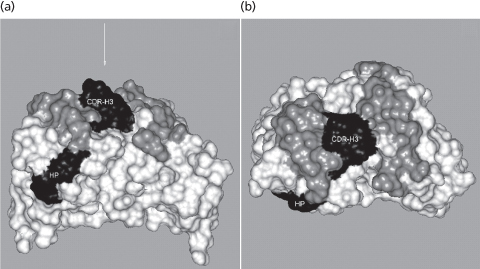
The possibility that IgM anti-I is always accompanied by at least traces of IgG and IgA autoantibodies is raised by the finding of Hsu and co-workers (1974). Using a PVP-augmented antiglobulin test in the autoanalyzer they found that, in patients with typical anti-I cold agglutinins, IgG and IgA could always be detected on the patient’s red cells in addition to C3 and C4. Similarly, Ratkin and co-workers (1973) prepared eluates from 19 sera from patients with cold agglutinin disease and regularly found an excess of IgG and of IgA, both having agglutinating activity of relatively low titre. They interpreted their observations to mean that in patients in whom IgM autoantibodies predominated, autoantibodies of classes IgG and IgA were also regularly present, although in lower titre.
In mycoplasma infection, when a patient develops potent cold autoagglutinins of anti-I specificity as a transient phenomenon the antibody is made of heterogeneous IgM and contains both κ and λ light chains (Costea et al. 1966), although the heterogeneity is restricted (see Feizi 1977).
Production of Cold Autoagglutinins Following Repeated Blood Transfusions
The production of cold autoagglutinins in humans, following alloimmunization and in association with a delayed haemolytic transfusion reaction, has been observed only occasionally (see Chapter 11).
Cold (Biphasic) Autohaemolysins
In the syndrome of paroxysmal cold haemoglobinuria (PCH) the patient’s serum contains a cold, complement-fixing antibody (reviewed by Petz 2008). This antibody, often referred to as the Donath–Landsteiner antibody after its discoverers, produces haemolysis both in vitro and in vivo when the blood is first cooled (to allow the binding of antibody) and then warmed (to provide optimal conditions for complement-mediated haemolysis). Because of this behaviour, the antibody is described as a ‘biphasic haemolysin’.
Although biphasic haemolysin was originally described in a patient with tertiary syphilis, the majority of cases seen nowadays are associated with viral infections, particularly in children. In one series of 11 cases, only three were definitely syphilitic; of five which were definitely non-syphilitic, one followed measles and one mumps (Worlledge and Rousso 1965). Biphasic haemolysin may also occur transiently following chickenpox, influenza-like illness and prophylactic immunization with measles vaccine (Bird et al. 1976a).
Of 19 patients with biphasic haemolysin reported by Sokol and co-workers (1982, 1984), 17 were children. All patients were non-syphilitic. In 10 of the children the biphasic haemolysin developed after an upper respiratory tract infection. The other patients had infections with adenovirus type 2, influenza A virus or Haemophilus influenzae; one had chickenpox. The authors stressed the fact that in the acute form that typically occurs in children, the onset of the haemolytic anaemia is sudden, usually with haemoglobinuria, prostration and pallor. In the chronic form haemolysis is only mild; this form occurred in only two patients, one a child and the other an adult. Biphasic haemolysin in an adult patient with pneumonia due to Klebsiella was described by Lau and co-workers (1983). An adult patient with a rare chronic form of PCH refractory to steroid therapy was successfully treated with rituximab (Koppel et al. 2007).
In one series, all of 22 patients with biphasic haemolysins were children, who developed the antibodies after infection, usually of the upper respiratory tract (Göttsche et al. 1990b).
It has been suggested that for the prevalent non-syphilitic form of the syndrome the term Donath– Landsteiner haemolytic anaemia should be used rather than PCH, as the clinical manifestations are rarely paroxysmal, seldom precipitated by cold and not necessarily characterized by haemoglobinuria (Wolach et al. 1981).
Several estimates of the relative frequency of biphasic haemolysin in AIHA are available. In one series of 347 cases of AIHA, the antibody was found in six, i.e. fewer than 2% (Petz and Garratty 1980, p. 54). Similarly, of red cell autoantibodies from 2000 patients, 48 (2.4%) were biphasic haemolysins (Engelfriet et al. 1982). On the other hand, the antibody was present in four of 34 (12%) acute cases of AIHA in children in one series (Habibi et al. 1974) and in 17 out of 42 (40%) cases in another (Sokol et al. 1984). The 22 patients with biphasic haemolysins described by Göttsche and co-workers (1990b) were among 599 patients with AIHA, 68 of whom were children.
Although maximum haemolysis is observed when red cells are left with biphasic haemolysin and complement in the cold phase, the requirement for complement in the cold phase is not absolute. Thus when red cells are first left at 0°C with EDTA-treated serum containing fairly potent antibody then washed and incubated at 37°C with fresh normal serum, some haemolysis occurs (Polley et al. 1962). Similarly, Hinz and co-workers (1961a) found that if PNH red cells were used, haemolysis occurred quite readily when complement was supplied only in the warm phase of the reaction. An experiment described by Dacie (1962, p. 553) shows clearly that the reason why much more haemolysis is found when complement is present in the cold phase of the reaction is that, on warming, antibody very rapidly elutes from the red cells so that at higher temperatures there is usually too little antibody on the cells to activate complement. Hinz and co-workers (1961b) showed that optimal lysis occurred even when only C1 was present with antibody in the cold phase of the reaction; C4 could be present either in the cold or warm phases but C2 and C3 were essential in the warm phase.
False-negative results may be observed due to hypocomplementaemia and it may then be necessary to add fresh normal serum to demonstrate the presence of the biphasic haemolysin (Wolach et al. 1981).
In cases in which biphasic haemolysin is associated with syphilis (tertiary or congenital) the antibody is seldom active above 20°C; that is to say, red cells and serum must be cooled to a temperature below 20°C if there is to be haemolysis on subsequent warming. In cases in which the antibody appears transiently in children following infections, the thermal range is greater and the antibody may be active in vitro up to a temperature as high as 32°C (see Bird et al. 1976a). A monophasic haemolysin acting in vitro up to 32°C, in an adult, was described by Ries and co-workers (1971).
As mentioned above, potent cold autoagglutinins which are readily lytic may be confused with biphasic haemolysin, but the latter is usually non-agglutinating, produces substantially more lysis, is IgG rather than IgM and has anti-P rather than anti-I specificity. A test that helps to distinguish unusually lytic anti-I from biphasic haemolysin is described above.
Specificity
Classically, biphasic haemolysin has the specificity anti-P (Levine et al. 1963; Worlledge and Rousso 1965). Very occasionally, the specificity may be anti-‘p’ (see Chapter 4).
Biphasic haemolysins with anti-P specificity are inhibited by globoside: some are more strongly inhibited by the Forssman glycolipid, which contains the globoside structure with an additional terminal GalNAc residue, suggesting that the antibodies are probably evoked by Forssman antigens which are widespread in animal tissues and microorganisms (Schwarting et al. 1979). Chambers and Rauck (1996) described a case of childhood acute haemolytic anaemia following parvovirus infection. In this case the reticulocyte count was low (1.0%, attributed to parvovirus infection, see Chapter 4) despite profound anaemia (haematocrit 14.5%). As P antigen is both the receptor for parvovirus B19 and the target for most biphasic haemolysins the authors speculate that interaction of the virus with P antigen may have triggered an auto-anti-P response.
Occasionally, biphasic haemolysins have a specificity outside the P system: anti-IH (Weiner et al. 1964), anti-I (Engelfriet et al. 1968a; Bell et al. 1973a), anti-i, as described above, or anti-Pr like (Judd et al. 1986). In practice, determination of the specificity of biphasic haemolysins is not helpful in diagnosis. On the other hand, in children with antibodies of wide thermal range and severe red cell destruction, confirmation of anti-P specificity may be helpful in treatment, as transfusion of pp red cells is sometimes very successful (see below).
Immunoglobulin Class
Biphasic haemolysin (of specificity anti-P) is composed of IgG (Adinolfi et al. 1962; Hinz 1963). If red cells are incubated at a temperature such as 15°C with fresh serum containing biphasic haemolysin and then washed at room temperature, they react weakly with anti-IgG but strongly with anti-C4 and anti-C3, as expected from the fact that the antibody elutes rapidly as the temperature is raised. During, and for some time after, an attack of haemoglobinuria, the red cells of patients with PCH give a positive direct antiglobulin test (DAT). Only complement components (presumably C3d and C4d) can be detected on the red cells.
Red Cell Transfusion in Patients with Biphasic Haemolysins
Red cell transfusion is seldom required in PCH. When the thermal range of the antibody extends only to 20°C or so in vitro, the patient is not severely anaemic. In patients in whom the thermal range extends to 30°C or more, severe anaemia does occur occasionally but in these patients the disease is usually transient and recovery has usually begun before the question of transfusion has to be considered. The successful use of P-negative red cells (from a bank of frozen blood) has been reported (Rausen et al. 1975) but unwashed, unwarmed P-positive blood has also been used successfully in three affected children (Wolach et al. 1981). In a child with PCH and severe anaemia, who did not respond to transfusion of P-positive blood, the transfusion of P-negative blood resulted in a sustained rise in Hb level (I Franklin and M Contreras, personal observation). The use of plasmapheresis to remove the Donath–Landsteiner antibody and ameliorate severe autoimmune haemolytic anaemia in a child following gastroenteritis is described by Roy-Burman and Glader (2002). The authors consider that because production of the Donath–Landsteiner antibody is transient and relatively brief in post-viral illness, removal of the antibody by plasmapheresis is less likely to be followed by significant rebound antibody production.
Harmless Warm Autoantibodies
IgG Subclass of Harmless Warm Antibodies
The affinity of Fc receptors for IgG4 is very low and subjects with only IgG4 on their red cells are expected to have a positive DAT but no signs of red cell destruction. With IgG2, the situation is more complex because, as explained in Chapter 3, there are two alleles of the gene that encodes the FcRIIa receptor on macrophages. As a result, some subjects have a low-affinity receptor for IgG2 and, in the presence of an IgG2 autoantibody have a positive DAT without signs of red cell destruction; others have a high-affinity receptor and the potential to destroy IgG2-coated cells. Indeed, some patients with only IgG2 on their red cells have haemolytic anaemia (CP Engelfriet, unpublished observations). However, IgG2-mediated destruction depends upon antigen specificity.
Although IgG1 and IgG3 readily adhere to Fc receptors and antibodies of these subclasses are expected to cause red cell destruction, in the case of IgG1, the number of molecules bound per cell must exceed a certain minimum number to bring about attachment to phagocytes and thus to cause red cell destruction (see below). Subjects with a relatively small number of IgG1 molecules per red cell are expected to have a positive DAT without signs of red cell destruction.
Positive Direct Antiglobulin Test in Apparently Normal Subjects
The fact that an apparently normal donor has a positive DAT is often first discovered when the donor’s red cells are used in crossmatching. Sixty-five cases were found in this way in one region during a period in which one million donations were collected. Assuming that for every 10 donors detected one was missed, the frequency of donors with a positive DAT was estimated to be one in 14 000 (Gorst et al. 1980). In another prospective survey donors with a positive DAT were discovered either by antiglobulin testing or by noting autoagglutination of a blood sample in an automated or manual test and then doing an antiglobulin test. The frequency of donors with a positive DAT was one in 13 000 (Habibi et al. 1980). Although the results of these two surveys look very similar there were apparent differences between the two. In the first there was only C3d (and C4d) on the red cells of 28 of the 65 donors. All donors with a positive DAT were haematologically normal; of 32 of the donors followed for many years, 31 remained well and only one, with a strongly positive DAT with anti-IgG, developed AIHA (Gorst et al. 1980).
In the second series, immunoglobulin was detectable on the red cells in all of 69 cases (IgG in 67, IgM in 2). Ten per cent of the donors had subnormal Hb values; a further 29% had reticulocytosis, with or without hyperbilirubinaemia: 61% appeared to be normal haematologically but when Cr survival studies were carried out in a few of these subjects, results were below normal in about 50% of the cases (Habibi et al. 1980). It should be noted that 25% of the donors with a positive DAT were receiving methyldopa, a circumstance that might have debarred them from donation in many countries. In any case, it must be said that the evidence presented for a haemolytic state in many of the donors was rather slight. No donor had a reticulocyte count higher than about 4.5% or a bilirubin value higher than 2.2 mg/dl (37 µmol/l). Slightly reduced Cr survival in haematologically normal subjects is difficult to interpret. Finally, in many of the donors who were followed for a period of 1 year or more, haematological findings became normal.
A very much higher frequency of positive DATs in normal donors than that found in the two series mentioned above was reported by Allan and Garratty (1980), namely one in 1000, but the discrepancy may be more apparent than real, as over 90% of the reactions were only ‘1+’ or less.
In 22 out of 23 normal donors with IgG on their red cells from the series of Gorst and co-workers (1980), the IgG subclass of the antibody was later investigated. In 20 of the cases it was solely IgG1 and the number of IgG1 molecules per red cell varied from 110 to 950; in the remaining two subjects the red cells were coated only with IgG4 (Stratton et al. 1983). In another series of 10 subjects, five had only IgG1, three IgG4, one IgG2 and one both IgG1 and IgG3 (Allan and Garratty 1980).
In normal donors with a positive DAT, the specificity of the autoantibody, as in patients with AIHA, is often related to Rh (Issitt et al. 1976; Habibi et al. 1980) but may be outside the Rh system, for example anti-Jka (Holmes et al. 1976) and anti-Xga (Yokoyama and McCoy 1967).
In normal subjects with IgG on their red cells, the red cells may be agglutinated by anti-complement as well as anti-IgG, although the frequency with which both IgG and complement have been found has varied widely in different series, i.e. 15% (Gorst et al. 1980); 44% (Allan and Garratty 1980) and 70% (Issitt et al. 1976).
Positive Direct Antiglobulin Test (IgG) in Hypergammaglobulinaemia
An association has been observed between hypergammaglobulinaemia and a positive DAT. Of 50 patients with an increased concentration of IgG in their serum, 25 had a positive DAT without signs of increased red cell destruction. The eluates from the red cells were unreactive (Huh et al. 1988). In another study of 20 patients with an increased serum IgG and a positive DAT, there were no signs of increased red cell destruction. These eluates were also unreactive (Heddle et al. 1988). In a prospective study of 44 patients with increased serum IgG, the DAT was positive in the three patients with the highest IgG concentrations. The DAT became positive in two other patients who were treated with high-dose intravenous immunoglobulin and, again, the eluates were unreactive (Heddle et al. 1988). In patients with a positive DAT but with an unreactive eluate, a significant correlation has been observed between the strength of the DAT reaction and the serum IgG concentration (Clark et al. 1992).
C3d (and C4d) Alone on Red Cells
In 40–47% of normal donors with a positive DAT, only complement is detected on the red cells (Allan and Garratty 1980; Gorst et al. 1980). C3d can be demonstrated on all normal red cells by using a sufficiently potent anti-C3d serum (Graham et al. 1976) and both C3d and C4d can be demonstrated by using the sensitive PVP-augmented antiglobulin test (Rosenfield and Jagathambal 1978). The presence of these fragments on red cells is taken as evidence of continuing low-grade activation of complement (see Chapter 3). There is no reason to believe that autoantibodies of any kind are responsible for this activation and it is therefore not logical to discuss this subject under the general heading of ‘harmless warm autoantibodies’, but it is nevertheless convenient.
The amount of C3d on the red cells of normal adults has been estimated by using rabbit IgG anti-C3d and 125I-labelled goat anti-rabbit IgG; in 174 normal adults there were estimated to be between 50 and 200 C3d molecules per red cell, i.e. too few to be detected in the ordinary DAT. There was no difference between males and females and no evidence of any change in the number of molecules per cell over the age range 20–65 years. There was also no evidence that the number was different in children (Chaplin et al. 1981). Other estimates of the number of C3d molecules per cell in normal adults are 207–427 (Freedman and Barefoot 1982) and 280–560 (Merry et al. 1983).
Weakly positive DATs due to increased amounts of C3d on the red cells appear to be relatively frequent in subjects who are ill. Dacie and Worlledge (1969) found that 40 out of 489 (8%) patients in hospital gave weakly positive antiglobulin reactions due to complement. Similarly, Freedman (1979) found that of 100 EDTA samples from hospital patients, taken at random, seven gave positive reactions with anti-C3d and anti-C4d; all seven patients were seriously ill. Again, in 8% of random hospital patients values greater than 230 C3d molecules per cell were found by Chaplin and co-workers (1981), who also noted that in random patients in hospital 33% had values for the numbers of C3d molecules per red cell that were above the range found in more than 90% of healthy adults.
In testing red cells with anti-C3d and anti-C4d, freshly taken EDTA blood should be used whenever possible, as the amounts of C3d and C4d on red cells in ACD blood may increase slightly during brief storage at 4°C (Engelfriet 1976); after 21 days of storage, the increase of C3d and C4d may be two-fold (H Chaplin, personal communication).
Positive Direct Antiglobulin Test Associated with Various Diseases, But Without Signs of Increased Red Cell Destruction
Malaria
A positive DAT has been found in 40–50% of West African children with falciparum malaria (Topley et al. 1973; Facer et al. 1979; Abdalla and Weatherall 1982). In most cases, only C3d is detected on the red cells but in some both C3d and IgG are present and, in a few, IgG alone. Although in one series there was a relationship between a positive DAT and anaemia (Facer et al. 1979), in the others there was not. It was suggested that a positive test might be associated with the development of immunity to malaria (Abdalla and Weatherall 1982).
On the other hand, some patients with falciparum malaria, with antibodies against triosephosphate, associated with a positive DAT, have a prolonged haemolytic anaemia (Ritter et al. 1993).
Kala Azar
The presence of complement on the red cells of patients with this disorder was reported by Woodruff and co-workers (1972). Of 67 patients with kala azar, 33% tested prior to antimonial therapy had a positive DAT (Vilela et al. 2002).
Patients on α-Methyldopa and Other Drugs
The development of a positive DAT without any evidence of a haemolytic process is very common in patients taking α-methyldopa and is found occasionally in patients taking a variety of other drugs. The subject is considered in more detail in the section on drug-induced haemolytic anaemia.
Patients with autoimmune haemolytic disease in spontaneous remission without signs of red cell destruction may have a positive DAT (Loutit and Mollison 1946). In a patient reported by Goldberg and Fudenberg (1968), the red cells were initially agglutinated by anti-IgG and anti-C3; the serum contained an IgM antibody reacting with IgG-coated red cells. After treatment with steroids, the patient went into complete haematological remission and the IgM antibody disappeared from the serum; however, the red cells were still strongly agglutinated by anti-IgG and anti-C3.
In a patient reported by von dem Borne and co-workers (1977), who initially suffered from severe AIHA, a long-lasting remission was induced with steroid therapy, and it was then found that the antibody on the patient’s cells was predominantly IgG4; the coated red cells induced only weak rosetting with monocytes in vitro and it was postulated that there had been a switch in the subclass of the autoantibody, with production of a subclass IgG4, which was incapable of producing destruction in vivo.
Harmful Warm Autoantibodies
As mentioned above, antibodies reacting as well, or better, at 37°C than at lower temperatures are found in about 80% of all cases of AIHA. In the warm antibody type of AIHA, the DAT is almost always positive but the indirect test (for antibody in serum) is sometimes negative. AIHA occurs without underlying disease in about 50% of cases. About half of warm antibody cases with underlying disease occur in patients with chronic lymphocytic leukaemia and lymphomas (Packman 2008).
IgG alone has been found in 18.3% (Petz and Garratty 1975), 36% (Worlledge 1978) and 64% (Engelfriet et al. 1982) of warm antibody cases.
IgG alone was found invariably in patients with a positive DAT associated with α-methyldopa in two series (Worlledge 1969; Issitt et al. 1976), although in a third, IgM and complement (Clq), in addition to IgG, were found on the red cells of all patients who developed α-methyldopa-induced haemolytic anaemia (Lalezari et al. 1982), results that could not be reproduced by one previous author (CP Engelfriet) or by Ben-Izhak and co-workers (1985). The detection of the IgM antibodies appears to depend on the anti-IgM serum used. It has been suggested that if the affinity of the anti-IgM for IgM is much greater than that of the IgM red cell antibodies for the red cell antigen, the IgM antibodies are removed from the red cell in the antiglobulin phase of the test (P Lalezari, personal communication).
IgG and complement have been found in 64.5% (Petz and Garratty 1980), 44.4% (Worlledge 1978) and about 34% (Engelfriet et al. 1982) of cases; in the latter series, IgG and complement were found on the red cells of all patients with a combination of IgG incomplete warm autoantibodies and warm haemolysins (see below).
When complement and IgG are found on the red cells of patients with incomplete warm autoantibodies, it does not follow that complement has been fixed by autoantibody. Some of the evidence for this assertion is as follows: (1) neither IgG warm autoantibodies present in the serum nor those detectable in an eluate from the red cells are capable of fixing complement in vitro; (2) in at least 50% of patients with IgA warm autoantibodies alone, complement is detectable on the red cells; and (3) the frequency with which both IgG and complement are found on the red cells is much higher in patients suffering from a typical immune complex disease such as systemic lupus erythematosus (SLE), than in other cases of the warm type of AIHA. Thus, in SLE, both IgG and complement were found on the red cells in all cases by Chaplin (1973) and Worlledge (1978), in virtually all cases by Petz and Garratty (1980) and in 81% of cases by Engelfriet and colleagues (1982).
IgG Subclass of Warm Autoantibodies
IgG warm autoantibodies are IgG1 in the vast majority of patients (Engelfriet et al. 1982). IgG1 alone was found in 72% of patients and IgG1 with antibodies of another subclass in 25%. In only 23 out of 572 patients was no IgG1 detectable. IgG2 and IgG4 antibodies were found the least frequently. Table 7.2 shows the frequency with which IgG autoantibodies of only one subclass were detected, and the relation of the subclass of the autoantibodies to increased red cell destruction. Determination of the IgG subclass of autoantibodies in eluates can readily be achieved using commercially available gel tests (Fabijanska-Mitek et al. 1997).
Table 7.2 Presence or absence of increased red cell destruction in patients with IgG warm autoantibodies of only one subclass.
As mentioned above, subjects whose red cells are coated with not more than 950 IgG1 molecules per cell show no signs of red cell destruction. On the other hand in patients with AIHA with only IgG1 on their red cells, the number of molecules per cell was found to be 1200 or more (Stratton et al. 1983). This finding agrees well with the observation that at least 1180 IgG1 anti-D molecules must be bound per cell for adherence to monocyte receptors to occur in vitro (Zupanska et al. 1986). There is a clear relationship between the number of IgG1 molecules per cell and the severity of haemolytic anaemia (van der Meulen et al. 1980). The role of IgG2 autoantibodies is uncertain; in subjects with high-affinity FcRIIa receptors, alloantibodies with A specificity are lytic but those with Rh specificity are not (Kumpel et al. 1996). IgG3 antibodies mediate lysis by monocytes even when present on red cells at too low a concentration to be detected in the normal DAT, which explains why the test is negative in some patients with AIHA. IgG4 autoantibodies do not cause red cell destruction.
The ability of different IgG subclasses to effect lysis of red cells is related to the nature of their interaction with Fc receptors and their ability to activate complement. The IgG Fc receptor family consists of several activating receptors and a single inhibitory receptor. Two activating receptors (FcγRI, FcγRIIIa) are common to humans and mice. Two additional receptors (FcγRIIa, FcγRIIIb) are found in humans but not in mice. The inhibitory receptor (FcγRIIb) is common to mice and man. Experiments carried out in mice lacking different Fc receptors have demonstrated that absence of activating receptors ablates tissue destruction in models of autoimmune disease, whereas inactivation of FcγRIIb exacerbates existing autoimmunity (reviewed in Hogarth 2002). Studies using transgenic mice expressing FcγRIIa show that crosslinking this receptor with antimouse platelet antibody results in a severe immune-mediated thrombocytopenia not found in transgene negative mice (McKenzie et al. 1999). Fossati Jimack and colleagues (2000) injected different IgG subclass switch variants of a low-affinity auto-anti-red cell antibody (4C8) into mice to induce AIHA and compared the pathogenicity of the different antibodies. They found the highest pathogenicity with IgG2a (20- to 100-fold more potent than IgG1or IgG2b) and IgG3 was not pathogenic at all. By comparing the results with wild-type mice and FcγR-deficient mice they could show that the differences in pathogenicity were related to the ability of the switch variants to react with the low-affinity FcγRIII. In a subsequent study, Azeredo da Silveira and co-workers (2002) compared subclass switch variants of a high-affinity anti-red cell autoantibody (34–3C) with those obtained with the low-affinity antibody. They found that the high-affinity antibodies (IgG2a = IgG2b > IgG3) activated complement, whereas the low-affinity antibodies (and high-affinity IgG1) did not activate complement. The pathogenicity of high-affinity IgG2b and IgG3 isotypes was more than 200-fold higher than the corresponding low-affinity isotypes. This study in the mouse illustrates very clearly that a high density of cell-bound IgG is required for efficient binding and activation of C1, with complement activation being related to antibody affinity and the density and distribution of antigen.
Complement alone was found in about 10% of cases in two series (Worlledge 1978; Petz and Garratty 1980), although no cases of this kind were found in another series (Issitt et al. 1976). Only complement was found on the red cells of all patients with cold autoagglutinins, biphasic haemolysins or warm haemolysins without the simultaneous presence (see below) of warm autoantibodies (von dem Borne et al. 1969; Engelfriet et al. 1982).
As in all patients on whose red cells complement is bound in vivo, C3d (actually C3dg, see Chapter 3) is the subcomponent of C3 present on circulating red cells, and similarly C4d (possibly C4dg) is the only subcomponent of C4 present.
IgA warm autoantibodies may be solely IgA (Engelfriet et al. 1968b). IgA alone was found in 3 out of 291 cases in one series (Worlledge 1978), in 2 out of 102 cases in another series (Petz and Garratty 1980), and in 11 out of 1374 patients in a third series (Engelfriet et al. 1982). One example of an IgA autoantibody with Rh specificity (anti-e) has been described (Stratton et al. 1972). An IgA autoantibody with specificity for the third extracellular loop of band 3 has also been described (Janvier et al. 2002). For optimal conditions for detecting bound IgA in the antiglobulin test, see Chapter 8. In about 50% of patients with IgA autoantibodies, complement as well as IgA can be detected on the red cells.
The clinical course of patients with IgA incomplete warm autoantibodies is very similar to that of patients with IgG antibodies. Destruction of red cells by IgA antibodies is brought about by adherence to Fc receptors for IgA on monocytes and macrophages. It has been shown that adherence to this receptor leads to cytotoxic damage (Clark et al. 1984) or phagocytosis (Maliszewski et al. 1985). The FcR for IgA(FcαRI,CD89) belongs to the immunoglobulin superfamily and contains an extracellular region of 206 amino acids, a transmembrane domain of 19 amino acids and a cytoplasmic region of 41 amino acids. The extracellular region consists of two Ig-like domains, EC1 and EC2, and six potential sites for N-glycosylation. The receptor binds IgA1 and IgA2 with an equal affinity (Ding et al. 2003).
IgM warm autoantibodies occur with about the same frequency as IgA warm autoantibodies, i.e. in about 1% of patients with warm autoantibodies. For example, in one series of 1374 patients, 13 had only IgM autoantibody on their cells (always accompanied by complement), 13 had mixed IgG and IgM warm autoantibodies (and complement), and a single patient had a mixture of IgA and IgM warm autoantibodies together with complement (Engelfriet et al. 1982). The presence of autoantibodies of more than one immunoglobulin class on the red cells is associated with severe haemolytic anaemia (Ben-Izhak et al. 1985). Garratty and co-workers (1997) describe three severe cases (two fatal) of AIHA associated with warm IgM autoantibodies and point out that the specificities of each antibody (Ena, Wrb and Pr) are all associated with glycophorin A. The severity of AIHA caused by antibodies of these specificities may be related to the role of glycophorin A an inhibitor of red cell lysis by autologous complement (Okada and Tanaka 1983; Tomita et al. 1993, see Chapter 6).
Brain and co-workers (2002) obtained evidence that binding of lectins (Maclura pomifera and wheatgerm agglutinin) and antibodies to glycophorin A make the red cell membrane leaky to cations.
Warm Autohaemolysins and Agglutinins
Nearly all warm autohaemolysins react in vitro only with enzyme-treated red cells, although some examples weakly sensitize untreated red cells to agglutination by anti-complement serum. Most warm autohaemolysins react with antigens susceptible to destruction by phospholipase; the rest react with antigens that are hardly, if at all, susceptible; warm haemolysins show no specificity for Ii or Rh antigens (Wolf and Roelcke 1989).
Warm haemolysins, which are nearly always IgM, were the only autoantibodies found in 165 out of 2000 patients with red cell autoantibodies (Engelfriet et al. 1982). When only IgM warm haemolysins, reacting only with enzyme-treated cells in vitro, are demonstrable in a patient’s serum, red cell survival is only slightly shortened (von dem Borne et al. 1969). IgM warm haemolysins also frequently occur together with warm autoantibodies, for example in 138 of the 2000 patients in one series (Engelfriet et al. 1982). Complement is found on the red cells of all patients with IgM warm autohaemolysins.
Rarely, warm autoantibodies are capable of agglutinating and haemolysing untreated normal red cells suspended in saline. Such autoantibodies were described by Chauffard and Vincent (1909), Dameshek and Schwartz (1938) and Dacie (1954), but are very rare. In one series they were found in only three of 2000 patients with red cell autoantibodies; their presence is associated with very severe intravascular haemolysis, which may be directly responsible for the death of the patient (Engelfriet et al. 1982).
Cold and Warm Autoantibodies Occurring Together
Patients with AIHA with both cold and warm autoantibodies in their serum are sometimes found: in one series the combination was recorded in 63 out of 865 patients (Sokol et al. 1981). In 25 of these patients studied in more detail, IgG and complement were detectable on the red cells in every case and anti-I or anti-i cold autoagglutinins, reactive at 30°C or above, were detectable in the serum. All the cases were severe; 56% were secondary, the commonest associated diseases being SLE and lymphoma (Sokol et al. 1983). In another series, a somewhat lower incidence of this kind of AIHA was reported, namely 12 out of 144 patients (Shulman et al. 1985); again, the haemolytic anaemia was severe in all cases and, again, many cases were secondary to SLE or lymphoma. Three of 46 patients with AIHA described by Kajii and colleagues (1991) had both IgGκ warm autoantibodies and IgMκ cold autoagglutinins. One patient had a lymphoma and the other two idiopathic AIHA. Mayer et al. (2008) found both warm autoantibodies and cold agglutinins active above 24’C in only 2 of 2194 patients with warm autoantibodies. These authors argue that these ‘mixed’ types of AIHA are rarer than previously thought. A few other cases have been described in which a patient with AIHA has had both IgG and IgM autoantibodies active at 37°C but in which the features have not been exactly the same as in the series described above. In one of these atypical cases both the IgM and the IgG autoantibodies reacted better in the cold but had a wide thermal range, the IgG antibody lysing enzyme-treated cells at 37°C (Moore and Chaplin 1973). In two other cases both IgM and IgG autoantibodies had anti-I specificity. There were many features that were quite atypical of CHAD; thus, the patients had a very severe haemolytic process unrelated to exposure to cold and responding well to steroids (Freedman and Newlands 1977). A case with many similarities was reported by Dacie (1967, p. 751).
Association of Red Cell Autoantibodies, Autoimmune Haemolytic Anaemia and Carcinoma
Erythrocyte autoantibodies and carcinoma are found together 12–13 times more often than expected from their relative frequencies. In patients with carcinoma, warm autoantibodies were about twice as common as cold ones; about 50% of carcinoma patients with autoantibodies had AIHA (Sokol et al. 1994).
Negative Direct Antiglobulin Test in Autoimmune Haemolytic Anaemia
About 10% of patients with the clinical picture of AIHA have a negative conventional DAT (Garratty 1994). In many of these cases IgG, IgM or IgA autoantibodies can be demonstrated by more sensitive methods (Petz and Branch 1983; Salama et al. 1985; Sokol et al. 1987). In five out of seven patients with a negative DAT on whose red cells an increased amount of IgG was detected with a more sensitive method, the anaemia was corrected by steroid therapy (Gutgsell et al. 1988).
Specificity of Warm Autoantibodies
Rh Related
A few warm autoantibodies are specific for one particular Rh antigen such as e (Weiner et al. 1953) or D (Holländer 1954); others react more strongly with e-positive than with e-negative samples (Dacie and Cutbush 1954) but the commonest pattern, found by Weiner and Vos (1963) in two-thirds of cases is to react well with all cells except for those of the type Rhnull.
Celano and Levine (1967) concluded that three specificities could be recognized: (1) anti-LW; (2) an antibody reacting with all samples except Rhnull; and (3) an antibody reacting with all samples including Rhnull.
Weiner and Vos (1963) classified their cases according to whether they reacted only with normal (nl) D-positive cells or also with ‘partially deleted’ (pdl) Rh-positive cells, for example D– –, or with both these types of cell and also with ‘deleted’ (dl) cells, i.e. Rhnull; of 50 cases tested by Marsh and co-workers (1972), three had specificity involving both Rh and U – about 40% of the antibodies in the series had no recognizable specificity. Anti-dl specificity, or ‘no recognizable specificity’ as some would call it, was found in 23 out of 33 cases associated with α-methyldopa and in 23 out of 30 normal subjects with a positive DAT by Issitt and co-workers (1976).
Subsequent biochemical studies have confirmed that many warm autoantibodies precipitate Rh polypeptides and RhAG from normal red cells, whereas others immunoprecipitate band 3, or band 3 and glycophorin A (Leddy et al. 1993). Iwamoto and co-workers (2001) expressed band 3, Rh polypeptides D, cE, ce, CE and chimeric antigens CE-D and D-CE in the eythroleukaemic line KU812 and tested the autoantibodies from 20 patients with AIHA for reactivity with the cloned transfected cell lines by flow cytometry. Fifteen of the autoantibody eluates reacted with at least one of the Rh expressing cell lines, and seven reacted with the band 3 expressing cell line.
Leddy and Bakemeier (1967) found a relationship between specificity and complement binding; with one exception, antibodies reacting weakly or not at all with Rhnull cells failed to bind complement, whereas 70% of antibodies reacting as well with Rhnull cells as with other cells did bind complement. A similar observation was made by Vos and co-workers (1970), namely that those eluates that fixed complement had broad specificities, as evidenced, for example, by the ability to react both with normal red cells and with Rhnull cells.
In patients who develop a positive DAT as a result of taking α-methyldopa, with or without haemolytic anaemia, the autoantibodies have the same Rh-like specificities as in idiopathic AIHA (Carstairs et al. 1966; Worlledge et al. 1966; Garratty and Petz 1975).
Often, mixtures of specific autoantibodies, for example auto-anti-e and autoantibodies with no recognizable specificity, occur together. In such cases the presence of the specific autoantibody may be suspected if the serum is titrated against red cells of different Rh phenotypes. Differential absorptions of the serum with R1R1, R2R2 and rr red cells confirm the presence of specific autoantibody or reveal a relative specificity (i.e. stronger reactions with red cells carrying certain antigens, e.g. E), when it has not previously been suspected. If the three red cells are properly selected, so as to cover between them the vast majority of important antigens, clinically significant alloantibodies can also be excluded (Wallhermfechtel et al. 1984). The additional use of polyethylene glycol (PEG) or LISS in the absorption procedure is reported to reduce markedly the number of absorptions required to identify alloantibodies in sera with autoantibodies and so decrease the time required for laboratory investigation (Cheng et al. 2001; Chiaroni et al. 2003).
When the autoantibody has a specificity resembling that of Rh alloantibodies, red cells that are compatible in vitro survive normally, or almost normally, in the recipient’s circulation (Holländer 1954; Ley et al. 1958; Mollison 1959; Högman et al. 1960). In the example shown in Figure 7.2, the patient was ccddee, with an autoantibody of apparent specificity anti-e. The mean lifespan of transfused e-positive (DCCee) red cells was about 8 days, which was similar to that of the patient’s own red cells (see Dacie 1962, p. 450), whereas the survival of e-negative (DccEE) red cells was only slightly subnormal. For references to further similar cases in which red cell survival has been studied, see Petz and Swisher (1989, pp. 565–567).
Figure 7.2 Survival, in a ddccee patient with autoimmune haemolytic anaemia, of e+ (DCCee) red cells (•), estimated by differential agglutination, and of e– (DccEE) cells (×), estimated by 51Cr labelling and corrected for Cr elution. The patient’s serum contained an autoantibody reacting preferentially with e+ cells. (The legend of this figure as published originally (Mollison 1959) stated incorrectly that the e+ cells were autologous and were labelled with 51Cr.)
(Source: Mollison 1959. Reproduced with permission of Oxford University Press.)
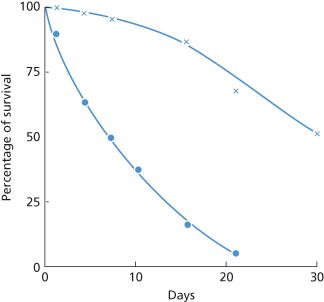
Specificity Mimicking that of Alloantibodies with Rh Specificity
A minority of warm autoantibodies at first sight appear to have the specificity of an Rh alloantibody, such as anti-E. For example, an eluate prepared from the red cells of a patient of phenotype DCCee may react more strongly with E-positive than with E-negative cells and thus appear to contain anti-E. However, in about 70% of such cases all antibody activity can be absorbed completely by red cells lacking the corresponding antigen, e.g. DCCee in the present example. The specificity of these autoantibodies seems in fact to be anti-Hr or anti-Hr0 (Issitt and Pavone 1978).
The case reported by van’t Veer and co-workers (1981) in which a negative DAT was found on the red cells of a patient with severe haemolytic anaemia, whereas strong autoantibodies of apparent anti-C and anti-e specificity were present in the serum demonstrates that such Rh specificities may be entirely illusory: not only (1) could the autoantibodies be absorbed with C-negative and e-negative cells, respectively, but also (2) during the episode in which the DAT was negative and the patient’s red cells (DCcee) did not react in vitro with the patient’s own autoantibodies, they reacted normally with auto-anti-C and allo-anti-e. The nature of the epitope with which such antibodies react is not known. Neither is it clear why the epitope should be so strongly associated with Rh alloantigens. The case reported by Rand and co-workers (1978) in which autoantibodies with anti-E specificity were eluted from an E-negative patient’s cells clearly demonstrates the mimicking nature of the specificity of the autoantibodies. Not only could the anti-E be absorbed to exhaustion by E-negative cells, but also the eluate from the E-negative cells used for absorption contained antibodies that again showed positive reactions only with E-positive cells. A possible explanation for this phenomenon is provided by observations on the specificity of anti-Is reported by Potter and co-workers (2002) who conclude that anti-I specificity is mediated through binding to a hydrophobic patch adjacent to the conventional antigen binding site (see p. 259). It is well established that many monoclonal anti-Ds are encoded by the same Ig gene (V4–34) as cold agglutinins and can exhibit cold agglutinin activity (Thorpe et al. 1998). The cold agglutinin activity itself could account for absorption of anti-D by D-negative red cells. Alternatively, the unusually high positive charge of anti-Ds and/or the considerable structural homology between D and CE polypeptides (discussed in Chapters 3 and 5; see also Thorpe et al. 1998) might predispose to absorption of these antibodies on all red cells irrespective of Rh phenotype. Some monoclonal anti-D recognized a ce polypeptide in which Arg145 was substituted by Thr, Thr 154 is not found in the D polypeptide. In this case, cold reactivity was ruled out as a possible explanation (Wagner et al. 2003).
Specificities Outside the Rh System
The possible involvement of Wrb in the specificity of autoantibodies was investigated by Issitt and co-workers (1976). Of 64 sera from patients with AIHA, two failed to react with Wr(a+ b–) cells and contained only anti-Wrb; the remaining sera reacted with Wr(a+ b–) red cells but, after absorption with these cells to remove anti-dl, 32 could be shown to contain anti-Wrb. The Wrb antigen is formed by the association of band 3 with glycophorin A (see Chapter 6). Some, but not all, warm autoantibodies capable of co-precipitating band 3 and glycophorin A were shown to have anti-Wrb specificity by Leddy et al. (1994). In patients with warm AIHA, autoantibodies with many other specificities are occasionally encountered, e.g. A (Szymanski et al. 1976); K, k and Kpb in association with weakening of Kell antigens (see below); Kx (Sullivan et al. 1987); Jka (van Loghem and van der Hart 1954); Jk3 (O’Day 1987); N (Bowman et al. 1974); S (Johnson et al. 1978); U (Marsh et al. 1972); Vel (Szalóky and van der Hart 1971); IT (Garratty et al. 1974); Ge (Reynolds et al. 1981); Sdx (Denegri et al. 1983) and Sc1 (Owen et al. 1992). For others, see Garratty (1994).
Kell Antibodies Associated with Autoimmune Haemolytic Anaemia
Several cases have been described in which a patient has developed a positive DAT, usually with overt haemolytic anaemia, and has been found to have autoantibodies of Kell specificity in the serum associated with weakening of Kell antigens. Seyfried and co-workers (1972) described a patient with potent anti-Kpb in his serum; during the period of his acute illness his own red cells reacted with anti-Kpb only after they had been treated with ficin. Sixteen weeks later, when the patient was better, Kell antigens were of normal strength. Beck and co-workers (1979) described a patient with similar serological findings but without AIHA. A patient has been described in whom, during consecutive relapses of autoimmune thrombocytopenia the Kell and Lutheran antigens became virtually undetectable. It was shown that this was due to transient absence of the Kell and Lutheran proteins during a relapse (Williamson et al. 1994). Other examples of weakening of red cell antigens in association with the appearance of alloantibodies or autoantibodies of the corresponding specificity are given in Chapter 3.
The frequency of autoantibodies with Kell specificity in patients with warm AIHA was estimated to be about 1 in 250 by Marsh and co-workers (1979).
Autoantibodies Mimicking Alloantibodies with Specificity Other Than Rh
Autoantibodies may mimic the specificity of anti-K (Garratty et al. 1979; Viggiano et al. 1982); anti-Jkb plus anti-Jk3 (Ellisor et al. 1983); anti-Kpb (Manny et al. 1983; Puig et al. 1986), anti-Fyb (Issitt et al. 1982; van’t Veer et al. 1984), anti-Fya plus anti-Fyb (Harris 1990) and anti-hrB-like (Vengelen-Tyler and Mogck 1991). In all of these cases, the patient was negative for the corresponding antigen, the antibodies could be absorbed by red cells negative for the corresponding antigen, and eluates from such cells again showed the mimicking specificity.
Autoantibodies Directed Against Non-Polymorphic Determinants
Some warm autoantibodies are directed against determinants that are clearly non-polymorphic. For example, anti-phospholipid antibodies, which occur in some patients with SLE and which may cause haemolytic anaemia (Arvieux et al. 1991) and antibodies against triosephosphate, found in some patients with falciparum malaria (see section on positive DAT in malaria, above).
Negative Direct Antiglobulin Test Despite Warm Autoantibodies in the Serum
In a case reported by Seyfried and co-workers (1972), during an episode of severe haemolysis, the DAT on the patient’s red cells was negative despite the presence of potent autoantibodies in the serum. The antibodies had anti-Kpb specificity, and weak anti-Kpb could be eluted from the patient’s red cells. The antigens of the Kell system were severely depressed at the time when the DAT was negative, but were of normal strength after recovery. Cases of transient depression of LW, associated with appearance of anti-LW in the serum, and without haemolytic anaemia, are described in Chapter 5. Several further cases, similar to the case of Seyfried and co-workers, have been observed in which the autoantibodies have had the following specificities: anti-E (Rand et al. 1978); anti-Rh of undefined specificity (Issitt et al. 1982; Vengelen-Tyler et al. 1983); ‘mimicking’ anti-C + anti-e (see above) (van’t Veer et al. 1981); anti-Ena (Garratty et al. 1983); anti-Kpb (Brendel et al. 1985; Puig et al. 1986); specificity for a high-frequency antigen in the Kell system (Vengelen-Tyler et al. 1987); anti-Jka (Ganly et al. 1988); anti-Jk3 (Issitt et al. 1990) and anti-Fya + Fyb (Harris 1990). In all the foregoing cases, there was total or severe depression of the antigens, against which the autoantibodies were directed (compare with Chapter 3). In some cases, although the DAT was negative, an eluate from the patient’s red cells contained weak autoantibodies of the same specificity as those in the serum. In some cases the DAT had been positive before the episode of severe haemolysis. In other cases the patient presented with a negative DAT and the antibodies were first thought to be alloantibodies.
In a case reported by Herron and co-workers (1987) the autoantibodies were found to react much more strongly with old, i.e. relatively dense, red cells than with young cells and it was suggested that the DAT during an episode of severe haemolysis became negative because only young red cells remained in the circulation.
Role of CD47 in Modulating the Severity of Autoimmune Haemolytic Anaemia in Mice
CD47 is a glycoprotein present on all cells. In human red cells it is associated with the proteins of the band 3-Rh complex (see also Chapters 3 and 5). CD47 appears to inhibit phagocytosis of normal circulating red cells by ligating the macrophage inhibitory receptor signal regulator protein alpha (SIRPalpha; Oldenborg et al. 2000). Non-obese diabetic (NOD) mice spontaneously develop mild AIHA aged between 300 and 550 days, whereas CD47-deficient NOD mice develop a severe AIHA at age 180–280 days. In addition, CD47-deficient C57BL/6 mice are much more susceptible to experimental passive AIHA induced by anti-red cell monoclonal antibodies than their wild-type counterparts (Oldenborg et al. 2002). These results are consistent with a role for CD47 in antibody-mediated phagocytosis.
Transfusion As a Stimulus for Allo- and Auto-Antibody Production
Young and co-workers (2004) carried out a retrospective analysis of blood bank records in order to determine the frequency of red cell autoimmunization associated with alloimmunization. They found 121 out of 2618 patients with a positive direct or indirect antiglobulin test (IAT) to have red cell autoantibodies. Forty-one of these patients also had alloantibodies and 12 of these developed their autoantibodies in temporal association with alloimmunization after recent blood transfusion. These authors conclude that autoimmunization and the development of AIHA should be recognized as a complication of allogeneic blood transfusion and recommend that once red cell autoimmunization is recognized, a strategy that minimizes exposure to allogeneic blood should be employed. In total, 6 out of 16 D-negative patients who developed anti-D after transfusion with D-positive red cells also made IgG autoantibody and three of these patients suffered prolonged haemolysis (Frohn et al. 2003). Shirey and co-workers (2002) advocate prophylactic antigen-matched donor blood for patients with warm autoantibodies in order to minimize the risk of alloantibody production.
Red Cell Transfusion and Other Therapy for Patients with Autoimmune Haemolytic Anaemia Associated with Warm Autoantibodies
In severe AIHA, transfusion produces only a very transient increase in Hb concentration and carries an increased risk of: (1) inducing the formation of alloantibodies; (2) increasing the potency of the autoantibodies; and (3) inducing haemoglobinuria due to autoantibody-mediated red cell destruction (Chaplin 1979). Accordingly, even in severely anaemic patients, it is usually best to begin treatment with corticosteroids, following which the Hb concentration usually starts to rise within 7 days (Petz and Garratty 1980, p. 392). If the effect of corticosteroids is not satisfactory, or if a quicker effect is needed, intravenous immunoglobulin (IVIG) can be given which, in very high doses (e.g. 0.4 g/kg per day) may have a very rapid effect (MacIntyre et al. 1985; Newland et al. 1986; Argiolu et al. 1990). However, in a study including 73 patients, IVIG had a rapid effect in only about 35% of cases, and particularly in patients with hepatomegaly and patients with a low pre-treatment haemoglobin. It is recommended that this treatment should be restricted to selected cases, for example to those in which the pre-treatment haemoglobin level is < 60–70 g/l or those with hepatomegaly (Flores et al. 1993). In the patients with warm haemolysins IVIG may be valuable, as Ig has been found to inhibit complement-dependent lysis (Frank et al. 1992).
Splenectomy is indicated in patients who have failed to respond to steroids and IVIG. Rituximab (monoclonal anti-CD20) has been used successfully in the treatment of AIHA in several studies. Shanafelt and co-workers (2003) consider that rituximab should be considered as salvage therapy for immune cytopenias that are refractory to both corticosteroid treatment and splenectomy. These authors report complete remission in 5 out of 12 patients with idiopathic thrombocytopenia, and two out of five patients with AIHA. Similar conclusions were reported by Bussone et al. (2009). However, serious adverse effects have been reported (reviewed in Petz 2001). Jourdan and co-workers (2003) report a case of severe AIHA that developed following rituximab therapy in a patient with a lymphoproliferative disorder. Patients refractory to steroids, spelenectomy and rituximab may respond to immunosuppressive therapy with cyclophosphamide, azathioprine or mycophenylate mofetil (Packman 2008). Treatment with cyclosporin (4 mg/kg per day) can be tried and may result in a fairly rapid increase in Hb concentration (Hershko et al. 1990).
There have been several reports of a high incidence of alloantibodies in patients with the warm antibody type of autoimmune haemolytic anaemia (WAIHA) who have been transfused. In three series the frequency was 32–38% and was as high as 75% in patients who had received more than five transfusions (Branch and Petz 1982; Laine and Beattie 1985; James et al. 1988; reviewed by Garratty and Petz 1994). In these three series, the patient’s serum was absorbed with autologous red cells before being tested for alloantibodies. In another series it was found that 44% of alloantibodies could not be detected before autoabsorption (Wallhermfechtel et al. 1984). There has been one report indicating that red cell alloimmunization is rare in WAIHA (Salama et al. 1992) but the patients’ sera were not absorbed with autologous red cells before being tested and alloantibodies may have been overlooked.
The risk of haemolysis after red cell transfusions in patients with AIHA with warm autoantibodies has been questioned. No instance of increased haemolysis was seen in 53 patients even in cases in which the transfused red cells were incompatible with autoantibodies detectable in the recipient’s serum (Salama et al. 1992).
Transfusion is indicated only in special circumstances, for example if the patient is severely anaemic and is going into cardiac failure, or has neurological signs, or has rapidly progressive anaemia, or is to undergo splenectomy. In most other circumstances it is better to use palliative measures, such as absolute bed rest, to counteract the decreased tolerance to exercise, while monitoring the Hb level.
If transfusions are given, it is important to group the patient’s red cells for all clinically significant alloantigens, to facilitate the identification of any alloantibodies that may be produced. In patients who have previously been transfused or have been pregnant, it is also important to try to exclude the presence of alloantibodies, which may be masked by the presence of autoantibodies. Either autoabsorption can be used or, if sufficient autologous red cells cannot be obtained, differential absorptions (see Chapter 8). It is helpful to obtain red cells from the patient before the first transfusion is given, and to store these at 4°C or frozen, so as to have cells for autoabsorptions if needed (Petz and Swisher 1989, p. 564).
When the presence of an alloantibody has been established, antigen-negative red cells must be selected for transfusion: the practice of transfusing ‘least incompatible red cells’ is not acceptable under these circumstances (see Laine and Beattie 1985).
In selecting red cells for transfusion, any blood group specificity of warm autoantibodies should when possible also be taken into account. In Rh D-negative females with auto-anti-e who have not yet reached the menopause, the red cells should, if possible, be e-negative as well as D-negative (i.e. ddccEE). In patients with auto-anti-e, e-negative (EE) red cells may survive better than e-positive cells (see Figure 7.1) but may stimulate the production of anti-E (Habibi et al. 1974).
When transfusing patients with AIHA, packed red cells should be given in just sufficient quantities to raise the Hb concentration to a level that will make it possible for other therapy to be applied. In acute anaemia, oxygen may have to be given. A few patients need regular transfusions despite all other forms of therapy.
As mentioned above, the presence of warm autoantibodies in the serum may make it difficult to detect alloantibodies (see also Chapter 8).
T-Cell Reactivity in AIHA
Peptides corresponding to sequences in the D and CE polypeptides stimulated proliferation of T cells from the peripheral blood and spleen of seven out of nine patients with AIHA. In total, four of the seven reactive patients had autoantibody to the Rh proteins. Multiple peptides were also stimulatory in two positive control donors who had been alloimmunized with D-positive red cells (Barker et al. 1997). Stimulation of peripheral blood mononuclear cells from patients with AIHA with D polypeptide resulted in either proliferation and secretion of γ-interferon or secretion of interleukin 10 (IL-10). Peptides derived from the D polypeptide that preferentially induced IL-10 secretion suppressed T-cell proliferation against D polypeptide, suggesting that it may be possible to ameliorate red cell autoantibody responses in man with inhibitory peptides (Hall et al. 2002). An important role for IL-10 in the function of peptide-induced regulatory T cells in vivo is apparent from successful peptide therapy, based on nasal administration of peptides corresponding to dominant T-cell epitopes, in mouse models of autoimmunity such as experimental allergic encephalomyelitis, which are associated with a deviation from a Th1 to a regulatory IL-10 CD4+ T-cell response (Sundstedt et al. 2003). Autoantigenic Rh peptide-specific IL-10-secreting T regulatory cell clones have been derived from a patient with AIHA, raising the possibility that antigen-specific T reg cell could be used for the treatment of AIHA (Ward et al. 2008).
Haemolytic Anaemia in Recipients of Allografts
Alloantibodies produced by donor lymphocytes in grafted tissue may simulate autoantibodies in the recipient and cause haemolytic anaemia (see Chapter 11).
Positive Direct Antiglobulin Tests Due to Anti-Red Cell Antibodies in Antilymphocyte Globulin
Antilymphocyte globulin (ALG) is commonly prepared in horses and the serum contains antibodies against human red cells. Following the injection of ALG, the recipient’s red cells acquire a positive DAT within 1–3 days (Lapinid et al. 1984; Swanson et al. 1984). The reaction between AHG reagent and the horse serum on the patient’s red cells can be inhibited by adding diluted horse serum to the AHG reagent without interfering with the reaction between the AHG reagent and any human alloantibodies which may be bound to the patient’s red cells (Swanson et al. 1984). In the serum of patients injected with ALG, autoantibodies can be detected, which usually show no obvious specificity but which occasionally have a Lu-related pattern (Anderson et al. 1985).
Occasionally, a positive DAT in a patient who has been injected with ALG is due to human red cell alloantibody; the alloantibody is derived from the plasma which has been added to the ALG to inhibit horse antibodies against human plasma proteins (Shirey et al. 1983).
Administration of ALG may occasionally produce immune red cell destruction; in the case described by Prchal and co-workers (1985) the DAT was negative with AHG reagent but positive with anti-horse immunoglobulin.
Antibodies Against Bound or Induced Antigens
Drug-Induced Immune Haemolytic Anaemia
Among cases of acquired immune haemolytic anaemia 18% were due to drugs in the series of Dacie and Worlledge (1969) and 12.4% in the series of Petz and Garratty (1980). The great majority of cases of drug-induced haemolytic anaemia were at one time due to α-methyldopa (Worlledge 1969) but this drug is now used much less frequently. Cases resulting from other drugs are very rare, penicillin-induced anaemia being the least uncommon (Petz and Garratty 1980). Recently, four cases of haemolytic anaemia (one fatal) have been described following piperacillin therapy (Arndt et al. 2002), one case attributed to tazobactum (Broadberry et al. 2004) and another to teicoplanin (Coluccio et al. 2004). Antibodies to piperacillin are common in blood donors and patients so diagnosis of piperacillin-induced haemolytic anaemia should only be made if testing is carried out in the presence of piperacillin (Leger et al. 2008).
Most drug-induced immune haemolytic anaemias since the late 1980s have been caused by second- and third-generation cephalosporins, cefotetan and ceftriaxone respectively (Arndt and Garratty 2002; Petz and Garratty 2004; Arndt and Garratty 2005). In total, 10 out of 35 cases of cefotetan-induced severe haemolytic anaemia studied by Garratty and co-workers (1999) were in patients who had received cefotetan prophylactically for obstetric and gynaecological procedures. Citak and co-workers (2002) report the development of haemolytic anaemia in a child with no underlying immune deficiency or haematological disease following treatment with ceftriaxone for a urinary tract infection. The patient had antibody against ceftriaxone and was successfully treated with high-dose corticosteroids. Arndt and co-workers (2012) report on 25 patients with antibodies to ceftriaxone. Nine of these patients had fatal drug-induced immune haemolytic anaemia. The authors comment that reactions in children were usually dramatic with severe intravascular haemolysis.
Non-steroidal anti-inflammatory drugs (NSAIDs) can also induce very severe AIHA. Jurgensen and co-workers (2001) describe a case of fatal AIHA with multisystem organ failure and shock caused by diclofenac-dependent red cell autoantibodies.
The fluoroquinolones, ciprofloxacin and levofloxacin, have been associated with causing AIHA in single case reports (Lim and Alam 2003; Oh et al. 2003).
Most drug molecules are not large enough to induce an immune response but may become immunogenic when bound to a macromolecule, for example a protein at the surface of a cell, to form a hapten–carrier complex. Antibodies formed against such a complex may be specific for the hapten, the hapten–carrier combining site or the carrier alone (see Shulman and Reid 1993).
There are several ways in which drugs may be responsible for a positive DAT, often associated with immune haemolytic anaemia (reviewed in Issitt and Anstee 1998; Petz and Garratty 2004).
Drug Adsorption Mechanism
The drug may bind firmly to red cells; when an antibody is formed against the drug, the drug-coated cells may be destroyed. The drug antibodies can be detected in vitro with washed drug-coated cells. In these cases, the antibodies are directed against the drug alone (i.e. the hapten) and can be absorbed by the drug. This mechanism has been called ‘the drug-adsorption mechanism’ (Garratty and Petz 1975). Penicillin acts in this way and so, occasionally, do other drugs, particularly some of the cephalosporins (see Garratty 1994).
In about 3% of patients with bacterial endocarditis receiving massive doses of i.v. penicillin, a positive DAT develops but AIHA occurs only occasionally; the first case, associated with the prolonged administration of penicillin in high dosage (20 million units or more daily for weeks), was described by Petz and Fudenberg (1966): the patient’s serum contained an IgG penicillin antibody of unusual potency. If it is necessary to continue giving penicillin to patients with AIHA due to penicillin antibodies, transfusions may be required. Normal red cells, uncoated with penicillin, will appear to be compatible on crossmatching but after transfusion will become coated in vivo and destroyed in the same way as the patient’s cells.
Although penicillin antibodies are usually IgG they may be partly IgM (Fudenberg and German 1960) or solely IgM (Bird et al. 1975), in which case complement is bound and the red cells are agglutinated by anti-C3. In patients with immune haemolytic anaemia due to penicillin antibody, the antibody can invariably be demonstrated in high titre in the serum, using red cells coated in vitro with penicillin (Petz and Garratty 1980; Petz and Branch 1985).
IgM or IgG antibodies reactive with penicillin-coated red cells have been found in the serum of about 4% of haematologically normal subjects (Fudenberg and German 1960).
The benzyl-penicilloyl groups are the most immunogenic of the haptenic groups of penicillin (Garratty and Petz 1975).
Several cases of severe or even fatal haemolytic anaemia due to second- or third-generation cephalosporins have been described in which the drug adsorption mechanism was involved (see Garratty et al. 1992). In some of the cases the immune complex mechanism described below also seems to have been involved (Marani et al. 1994; Ogburn et al. 1994).
Trimolecular Complex Mechanism
The drug does not bind firmly to red cells so that drug-coated cells cannot be prepared. It has been suggested that in these cases, when antibodies are formed against the drug, immune complexes attach to the red cell. This immune complex theory has been criticized for the following reasons: (1) certain drugs cause haemolytic anaemia in some patients but immune thrombocytopenia in others implying that a specific membrane component is involved; (2) drug antibodies attach to the cell membrane by their Fab part suggesting specific binding rather than passive adsorption of immune complexes; (3) the drug antibodies cannot be absorbed by the drug alone and can only be detected by bringing red cells, free drug and antibodies together; and (4) the binding of the drug antibodies may depend on the presence of a particular red cell antigen, which implies that the drug binds to the cell surface, albeit loosely (Salama and Mueller-Eckhardt 1987a). It seems therefore more likely that a trimolecular complex of the drug, the drug antibody and a component on the red cell membrane is formed. (For a survey of the subject, see Shulman and Reid 1993 and Garratty 1994, who also gives a list of drugs acting in this way.) Drugs that produce red cell destruction by this mechanism can do so even when given in low doses. The haemolysis is arrested within 1–2 days of stopping the drug. The antibodies are often IgM and complement-activating and then only complement can be detected on the patient’s red cells (Garratty and Petz 1975).
In some cases, the antibodies are directed against a metabolite rather then the drug itself (Salama and Mueller-Eckhardt 1985, 1987a,b; Kim et al. 2002). The antibodies can then be detected by using urine from subjects who have taken the drug. Bougie and co-workers (1997) describe a case of haemolytic anaemia and subsequent renal failure resulting from diclofenac in which the patient had an antibody specific for a glucuronide conjugate of a known metabolite of diclofenac (4′-OH hydroxydiclofenac). The antibody could be demonstrated in the patient’s serum with red cells in the presence of urine taken from individuals who had ingested diclofenac. These authors point out that as glucuronidation is a common pathway of drug metabolism, studies on glucuronidation of other common medications associated with immune haemolytic anaemia should be considered.
Drug-Induced Autoantibody Formation
The drug does not bind firmly to red cells, antibody against the drug is not formed, but IgG autoantibodies are induced. α-Methyldopa and levodopa are prime examples of drugs acting in this way. In 15–20% of patients receiving α-methyldopa, the DAT becomes positive after 3–6 months’ treatment; the development of a positive DAT is dose dependent (Carstairs et al. 1966). Only about 1% of patients receiving the drug develop haemolytic anaemia.
It has been suggested that α-methyldopa induces red cell autoantibodies by inhibiting the activity of suppressor T lymphocytes (Kirtland et al. 1980). Although no effect on suppressor cells could be demonstrated in one investigation (Garratty et al. 1986), a drug, lobenzarit, which inhibits suppressor cell function, has been found to induce α-methyldopa-type autoimmune haemolytic anaemia (Andou et al. 1994).
α-Interferon seems to be responsible for the development of autoantibodies to various structural proteins or receptors and for the exacerbation of autoimmune disease (Conlon et al. 1990). The development of warm red cell autoantibodies has been observed in a patient receiving α-interferon and IL-2 (Perez et al. 1991). These various effects are believed to be due to the inhibition of normal cellular immune suppressor mechanisms.
As stated above, some drugs that do not bind firmly to red cells induce both anti-drug antibodies and red cell autoantibodies, for example nomifensine (Martlew 1986; Salama and Mueller-Eckhardt 1987a), tolmetin and suprofen (van Dijk et al. 1989).
The inference has been drawn that even when drugs bind loosely to red cells, they can induce the formation of antibodies against a red cell antigen alone (Salama and Mueller-Eckhardt 1987a). Alternatively the drug could directly influence the immune response against autoantigens (Kirtland et al. 1980).
Non-Specific Adsorption of Proteins
The drug may alter the red cell membrane in some way so that proteins are adsorbed non-specifically. Cephalosporin and cisplatin are believed to act in this way as a rule. This mechanism has not been shown to result in haemolytic anaemia unless antibodies against the drug are formed.
Effect of Red Cell Antigens on the Binding of Drug–Antibody Complexes
In a case in which streptomycin was involved, the drug was apparently bound to the red cell membrane through chemical groups related to M and possibly D (Martinez Letona et al. 1977). Several similar cases in which various drugs and different red cell antigens were involved have been reported (for a survey, see Garratty 1994).
Treatment of Drug-Induced Haemolytic Anaemia
In cases in which antibodies are involved against a drug that binds firmly to the red cell and in cases in which immune complexes are responsible for the destruction of the red cells, stopping the drug is sufficient to arrest the haemolytic process and treatment of the haemolytic anaemia is rarely necessary. In cases in which it is impossible to stop the drug and the patient is anaemic, red cell transfusions should be given. In AIHA induced by α-methyldopa, the drug must be stopped, but red cell destruction may continue for weeks or months. If treatment is required it is the same as for patients with drug-independent warm AIHA.
In occasional patients, autoantibodies disappear despite continued administration of the drug (Habibi 1983).
Antibodies Against Other Bound Antigens
Fatty Acid-Dependent Agglutinin (‘Albumin Agglutinin’)
The serum of a small proportion of people agglutinates red cells suspended in albumin but not those suspended in saline (Weiner et al. 1956). Agglutination is found only with caprylate-treated albumin (Golde et al. 1969, 1973) and the antibody is in fact directed against sodium caprylate or other fatty acid salts and not against albumin at all (Beck et al. 1976). The term ‘fatty acid-dependent agglutinin’ is therefore preferable to the previously used ‘albumin agglutinin’. Fatty acid-dependent agglutinins may cause false-positive reactions in slide tests in which blood grouping reagents containing albumin are used (Reid et al. 1975; Case 1976) and in the IAT test if albumin is used in the sensitizing phase of the reaction.
Antibiotics
Antibiotics are added to samples of red cells that are distributed commercially for the identification of alloantibodies. Such cells may give false-positive results if antibodies against the relevant antibiotic are present in a sample of serum. In a systematic search for such antibodies, Watson and Joubert (1960) found that 6 out of 1700 routine blood bank serum samples agglutinated chloramphenicol-treated cells. Three examples of an antibody of this kind were found to be IgM and two bound complement (Beattie et al. 1976). An IgA antibody agglutinating red cells suspended in 0.1 mg of neomycin/ml was described by Hysell and co-workers (1975). Antibodies vs. penicillin-treated red cells are described above.
Acriflavine
Some commercial anti-B-sera have acriflavine added to them as a colouring agent and this may be a cause of false-positive results if anti-acriflavine antibodies are present in a patient’s serum, possibly as a result of previous exposure to acriflavine. The antibodies may cause agglutination of normal red cells in the presence of a 1 in 150 000 dilution of acriflavine (Beattie and Zuelzer 1968; Beattie et al. 1971).
Immune Complexes Adsorbed to Red Cells in Vitro in Ulcerative Colitis
In occasional patients with ulcerative colitis, the DAT on clotted samples is positive but on anticoagulated samples is negative. Allogeneic red cells give a positive IAT with the patient’s serum but a negative test with plasma. It is postulated that the patient’s plasma contains an antibody against an activated coagulation factor and that, during clotting, immune complexes form and attach to the red cells (Garratty et al. 1980).
Lactose- or Glucose-Treated Red Cells
Antibodies have been described which agglutinated any red cells that had been incubated with lactose (Gray 1964) or glucose (Lewis et al. 1980). In the latter case, red cells from patients with diabetes reacted, although after incubation in saline, the cells were no longer agglutinated. Examples of anti-M and anti-N reacting only with lactose- or glucose-treated red cells are described in the preceding chapter.
Antibody Against Chemically Altered Red Cells (the LOX Antigen)
Red cells exposed to citrate–phosphate–dextrose solution in particular batches of plastic blood packs may acquire a new red cell antigenic determinant ‘LOX’, reacting with an antibody present in normal serum. The development of this antigen is probably associated with sterilization of the packs with propylene oxide gas (Bruce and Mitchell 1981).
Polyagglutinability
Red cells are said to be polyagglutinable when they are agglutinated by almost all samples of normal human serum although not by the patient’s own serum. The commonest forms of polyagglutinability are due to exposure, by the action of bacterial enzymes, of antigenic determinants (T, Tk, Th, Tx), which form part of the structure of the normal red cell membrane, but which are usually hidden. Another form of polyagglutinability due to somatic mutation leading to the emergence of a line of red cells lacking an enzyme essential for the formation of normal red cell antigens; as a result, a normally hidden antigen, Tn, is exposed. In all the foregoing cases, the red cells are polyagglutinable because antibodies (anti-T, etc.) corresponding to the determinants are present in serum from all normal adults (although not in serum from newborn infants). Why these antibodies are in all normal sera is not known but, like anti-A and anti-B, this may be related to the widespread occurrence of the antigens in the environment. Chicks kept in germ-free conditions developed anti-T and anti-Tn when fed Escherichia coli O86 in their drinking water (Springer and Tegtmeyer 1981). Tn has been found in several helminth parasites, including Echinococcus granulosus, Taenia hydatigena and Fasciola hepatica (Casaravilla et al. 2003) and in human skin mites (Kanitakis et al. 1997). Further forms of polyagglutinability may be due to the inheritance of an antigen (C3d, NOR or HEMPAS) for which a corresponding antibody is present in almost all normal human sera.
T Activation
Exposure of T Antigen in Vitro
As Figure 7.3 shows, the T determinant is normally covered by N-acetylneuraminic acid and can therefore be described as a cryptantigen. The antigen can be exposed by the action of bacterial or viral neuraminidases.
Figure 7.3 Proposed structure of the major O-glycosidically linked oligosaccharides of the sialoglycoproteins in normal, T- and Tn-exposed erythrocyte membranes. NeuAc, N-acetylneuraminic acid; Gal, D-galactose; GalNAcα1, N-acetyl-D-galactosamine. *It is possible that this NeuAc residue is present in some, but not all, Tn structures (PD Issitt, personal communication)
(Source: Adapted from Anstee 1981. Reproduced with permission of Elsevier.)
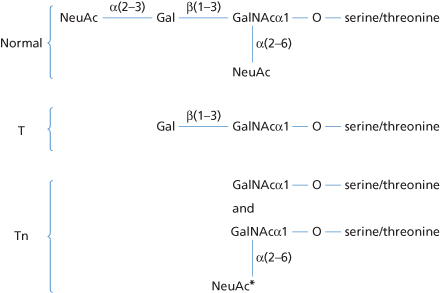
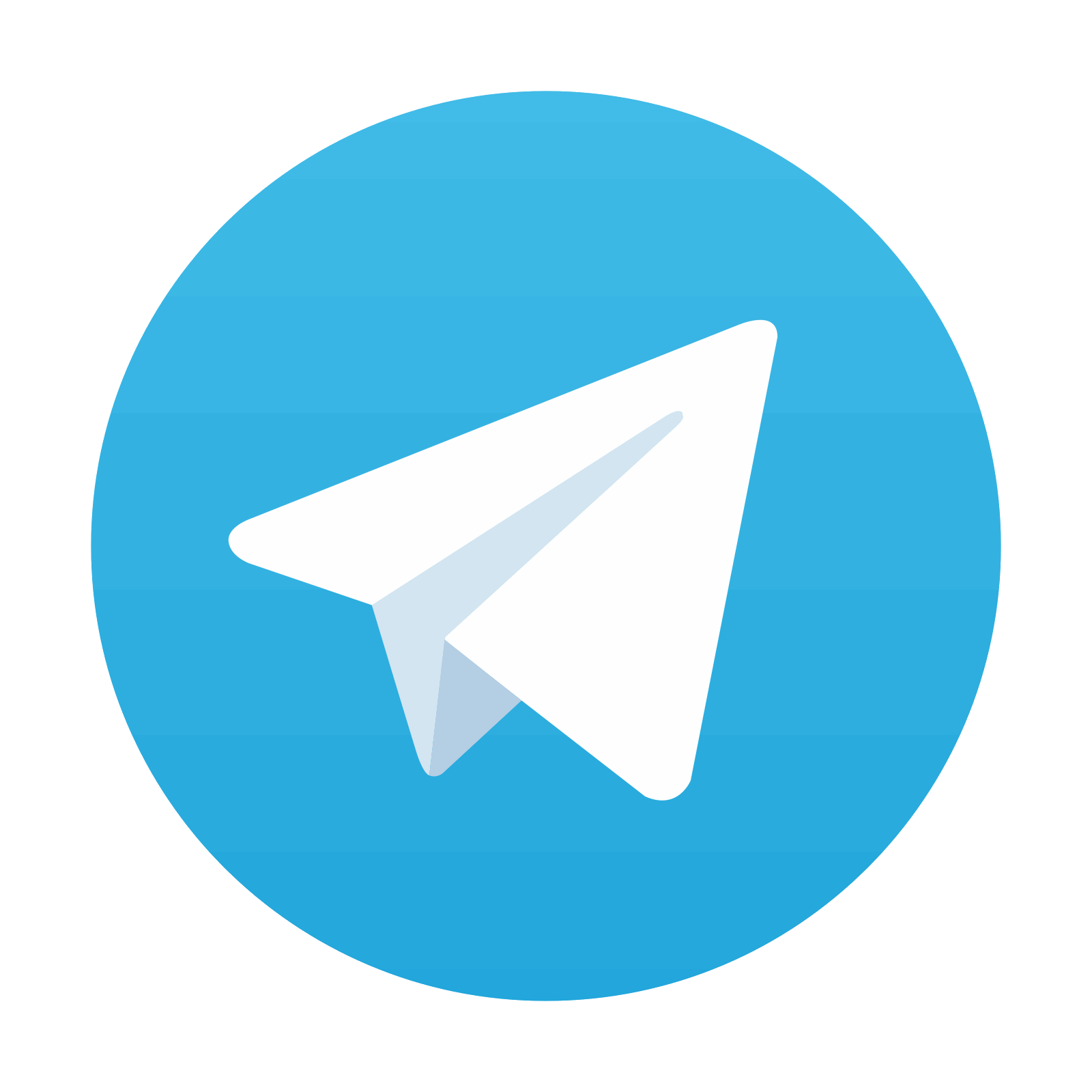
Stay updated, free articles. Join our Telegram channel

Full access? Get Clinical Tree
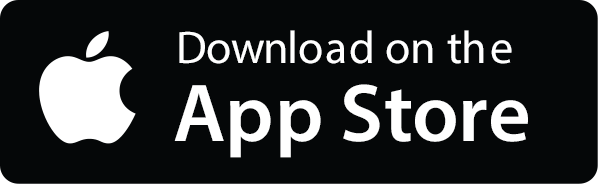
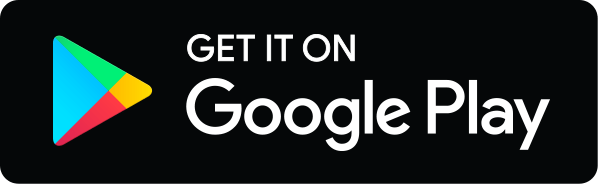