Fig. 18.1
Basic IONM equipment setup ET endotracheal tube, REC recording electrodes, GND ground electrodes (Adapted from Randolph et al. [4] permissions awaited)
Various nerve monitoring formats are available for IONM. These include glottic observation [39–41], laryngeal palpation [21, 25, 42, 43], endotracheal tube–based surface electrodes [26, 44–50], postcricoid surface electrodes [51, 52], and many more. These can be audio only or ones that provide both audio and visual waveform information. The major drawback with audio only systems is that the information such as wave-form amplitude, latency, and morphology which may provide insights into the pathologic state of the nerve is unavailable. As our understanding of RLN electrophysiology improves this information may prove to be useful in surgical decision making. Also, at times, it might be difficult to differentiate between the signal and the artifact with audio-only systems. Currently, the most preferred neural monitoring equipment is an endotracheal tube–based system that includes a visual graphic documentation of the EMG waveform elicited from thyroarytenoid muscle [53]. Prefabricated endotracheal tubes with paired stainless steel electrodes exposed at the level of the glottis or standard tubes with thin electrodes placed over the tube with adhesive pads can be used. Another option is to use needle electrodes instead of surface electrodes but these carry additional risk of injury to the cords, laceration or hematoma, and are less preferred.
Introduction of any technique comes with its own learning curve. Studies have suggested that optimum functioning of the IONM can be achieved after performance of 50–100 monitoring cases [4, 54]. Dionigi et al. [54] have reported that setup related problems can occur in up to 10 % of patients. The most common problem being that related to tube positioning and occurred in 53 % of the patients. Chiang et al. have shown that routine application of IONM can reduce setup related problems from 4.4 to 0 % [55]. Thus, adherence to a standard setup and tube placement algorithm reduces the occurrence of intraoperative problems. Ground electrodes are adhered to the shoulder or the sternum area. Poor grounding can cause a noisy baseline, making it difficult to interpret the EMG data. It is also important to keep the electrocautery unit more than 10 ft away from the neural monitoring unit to avoid electrical interference. Choice of the stimulating electrode depends upon its intended use. Monopolar electrodes are generally preferred for initial neural mapping, whereas bipolar electrodes may be more sensitive in stimulating a focal point on the nerve once it is identified. The major drawback with bipolar electrodes being that incorrect orientation of the anode (positive) and cathode (negative) relative to the nerve may give a false negative signal [56]. After the equipment is setup, care is taken to ensure that the recording side and the stimulation side circuitry is functional (Fig. 18.1).
18.2.4 Anesthesia
An important member of the team performing IONM is the anesthesiologist. A detailed discussion on special needs of anesthesia during nerve monitoring is imperative prior to surgery. It is important that long acting muscle relaxants are avoided during surgery to enhance the accuracy of the nerve monitoring system. Short acting muscle relaxants such as succinyl choline can be used for induction with the aim of recovering normal muscle twitch activity within minutes of intubation. A good way of ensuring optimum contact between the electrodes and the vocal cord is to select an endotracheal tube with the largest admissible size. Use of a drying agent and oral suctioning of any secretions are also helpful in this regard. Tube displacement of up to 6 cm has been documented during positioning of the patient [57]. It is thus prudent to check tube placement after final positioning. The gold standard for tube placement is visual confirmation [4]. Another reliable way of confirming tube positioning is identification of “respiratory variations.” These are waveforms with amplitude between 30 and 70 μV which are observed in the small window of time when the effect of initial muscle relaxant wears off and the patient is in a lighter plane of anesthesia. They appear as coarsening of the baseline EMG activity and can be seen reliably in more than 90 % of the patients. In a recently concluded study, our unit found that presence of respiratory variations independently predicted a good evoked response intraoperatively and obviated the need of a repeat laryngoscopy in most patients [58]. Impedance checks for a higher overall impedance (more than 5 Ω) or an imbalance between the two sides (more than 1 Ω) is another good tactic for checking the robustness of the IONM circuitry. During the initial setup, the monitor is set at an event threshold of 100 μV and the stimulator probe at 2 mA for initial neural mapping. This can be reduced to 1 mA after the nerve is identified for a more focal stimulation and end of surgery prognostication. These levels have been reported to be extremely safe and no unfavorable effects have been reported [59]. In the surgical field, the stimulator probe is tested on the strap muscle first to look for a local muscle twitch and to confirm that the current is being reported back on the monitor. Prior to labeling any tissue as being truly negative (not the RLN), a predissection supra threshold vagal stimulation (V1) should be performed to obtain a true positive signal verifying the integrity of the IONM circuitry. The suprathreshold vagal stimulation utilizes 2 mA current and is usually performed effectively without direct vagal dissection by placing the stimulator probe between the jugular vein and carotid artery. The surgeon should also feel for a “laryngeal twitch” response from the laryngeal muscles by rolling the fingers on the side of the larynx. This response always accompanies a true positive signal.
The human RLN and vagus nerve typically have biphasic or a triphasic waveform. Parameters such as amplitude and latency in the waveform can provide useful prognostic information regarding glottis function at the end of surgery. Amplitude is defined as the vertical height of the apex of the positive initial waveform deflection to the lowest point in the net subsequent opposite polarity phase of the waveform (Fig. 18.2). Interestingly, a study performed by Caragacianu et al. [59], reports that the strength of the stimulation current (1 mA vs. 2 mA) had no significant impact on the amplitude recorded. It also notes that a final amplitude of >250 μV recorded during postdissection vagal stimulation at 1 mA accurately predicts postoperative glottic function. However, currently there is a lack of consensus over the definition for latency. The INMSG defines latency as the time from the stimulation spike to the first evoked waveform peak [4]. Latencies recorded during intraoperative monitoring are distinctive and can differentiate artifacts from neural stimulated structures and also can be used to distinguish superior laryngeal nerve, vagus nerve, and RLN and also distinguish left from right vagus nerve easily.
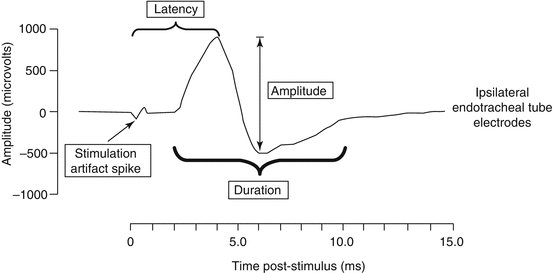
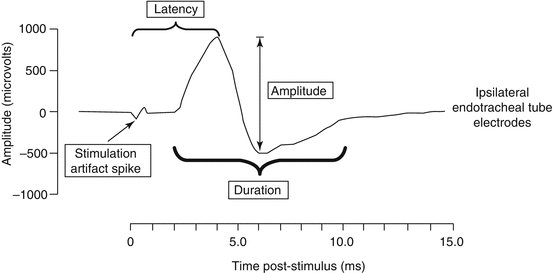
Fig. 18.2
A typical triphasic evoked waveform captured during IONM with various parameters indicated (Adapted from Intraoperative monitoring: normative range associated with normal postoperative glottic function. Caragacianu et al. [59] permission awaited)
18.2.5 Loss of Signal and Its Interpretation (Fig. 18.3)
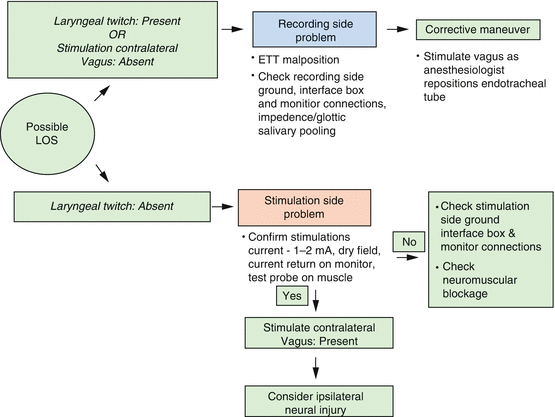
Fig. 18.3
Intraoperative evaluation of loss of signal (LOS) standard (Adapted from Randolph et al. [4]. Permissions awaited)
Absence of EMG activity or loss of signal (LOS) during surgery should prompt a quick review of the IONM setup. The first response of the surgeon should be to feel for the laryngeal twitch response. If the response is present then the problem is likely to be on the recording side of the system while if the twitch response is absent then it is considered a stimulation side problem. Adherence to the accompanying algorithm (Fig. 18.3) can guide the surgeon to adopt a systematic approach towards managing such a scenario. A true loss of signal is currently defined only when the following conditions are met:
1.
Presence of a satisfactory EMG (Amplitude >100 μV) prior to the event
2.
No or low response (i.e., 100 μV or lower) with stimulation at 1–2 mA in a dry field
3.
Absence of laryngeal twitch and/or glottis twitch on ipsilateral vagal stimulation
A true LOS strongly suggests a neural injury. Identification of the point of injury can allow the surgeon to guide further management of the injured nerve. Once the signal is lost, the surgeon can locate the point of injury by stimulating the RLN at the laryngeal entry point [60] and then proceeding proximally to identify the injured segment. If identified, segmental injuries are labeled as a Type 1 Injury. Sometimes, the whole course of the RLN and the vagus nerve is nonconductive, this is labeled as a global nerve injury and is defined as a Type II injury. Detection of LOS in a visually intact nerve is the most significant advantage of IONM, which can guide the surgeon in reconsidering surgery on the contralateral side and can prevent a potential bilateral cord paralysis [56].
18.2.6 Prognostic Testing Errors
Postdissection suprathreshold RLN and vagus stimulation is a reliable indicator of postoperative glottis function. As mentioned earlier, numerous studies have shown a high negative predictive value (>95 %) for the same. However, the following errors might occur while using IONM.
1.
False positive errors: (Errors where there is an LOS intraoperatively but the nerve is functional postoperatively). These can occur in the following conditions
(i)
Stimulation of the nerve in a wet bloody field may yield a false positive LOS
(ii)
Equipment errors on the recording side (tube displacement, improper grounding of electrodes)
(iii)
Prolonged neuromuscular blockade
(iv)
Early neuronal recovery especially if the postoperative laryngoscopy is delayed by a few days
2.
False negative errors: (Errors where there is a positive signal present at the end of the surgery stimulation of RLN but absence of nerve function postoperatively). Although uncommon, especially if IONM is performed in a systematic manner, these errors can occur due to:
(i)
Stimulation of the nerve distal to the site of injury. This error can be avoided by postdissection vagal stimulation.
(ii)
Endotracheal tube related injury such as laryngeal edema and dislocation of the arytenoid cartilage.
(iii)
Injury to the nerve after the final stimulation – during wound closure.
(iv)
In situations where there is an extralaryngeal branching of the RLN, a posterior branch injury may not be detected by IONM if postcricoid electrodes are not used. This may present as an abduction defect on laryngoscopy.
18.3 Promising Advances in IONM
18.3.1 Continuous Vagal Monitoring and Neural Injury Prevention
While IONM in its present format, is tremendously useful in assisting a surgeon, its greatest shortcoming is that it can only intermittently evaluate the functional integrity of the RLN, thus allowing the nerve to be at risk for injuries in-between stimulations [3, 31]. This could underlie the notion that, IONM, in its current format may have limited ability to prevent neural injury [31, 61, 62]. Continuous IONM (CIONM) with vagal nerve electrode provides a real-time EMG data by monitoring the entire vagus and RLN functional integrity uninterruptedly throughout the surgery. This real-time electrophysiological information from RLN obtained by CIONM is extremely vital as it alerts the surgeon prior to the occurrence of irreversible neural damage and thereby evading a possible permanent injury to the RLN. CIONM is not associated with significant neural, cardiac, pulmonary, or gastrointestinal vagal side effects [63, 64].
CIONM, through adverse EMG changes, can alert a surgeon regarding an impending neural injury and allow the surgeons to prompt a corrective action to preserve nerve function by aborting maneuvers that lead to adverse EMG changes and thereby possibly preventing permanent vocal cord paralysis [65]. However, it is essential that, a surgeon is able to differentiate between true adverse events and artifacts as well as can isolate meaningful adverse events. Our group has identified mild (mCE) and severe combined events (sCE) by combining reduction in amplitude with increase in latency (Table 18.1). In our study, notably, mCE and isolated amplitude or latency changes were not associated with vocal cord paralysis, while a sCE denoted a typically reversible electrophysiologic event if the related surgical maneuver was aborted, otherwise sCE could lead to LOS (which typically is much less reversible) and to possible postoperative vocal cord paralysis. Thus, modification of surgical maneuver associated with sCE can prevent subsequent neural injury leading to postoperative vocal cord paralysis. However, surgeons should keep in mind that any IONM format is more useful for preventing impeding neural damage secondary to a stretch or compression injury than the neural damage due to an inadvertent neural transection injury [65].
Table 18.1
Mild and severe combined events
Mild combined event (mCE): | Amplitude decrease of >50–70 % with a concordant latency increase of 5–10 % |
Severe combined event (sCE): | Amplitude decrease of >70 % with a concordant latency increase of >10 % |
18.3.2 Superior Laryngeal Nerve (SLN) Monitoring
RLN paralysis and hypoparathyroidism are the most common complications discussed when a thyroidectomy is planned but injury to external branch of SLN (EBSLN) can occur in up to 58 % of patients undergoing thyroid surgery [66]. EBSLN is at risk of injury during superior pole dissection and ligation of superior thyroid vessels. Injury to external branch of the SLN (EBSLN) results in cricothyroid muscle dysfunction and affects vocal projection and the ability to produce higher registers of the voice. While subtle, these voice changes can significantly impact professional voice users. The injury to EBSLN is difficult to identify intraoperatively as well as by postoperative laryngeal exam. Intraoperative visual identification of EBSLN is limited as 20 % of EBSLNs run a subfasical course [67]. IONM is now widely accepted as an adjunct to visual identification of the RLN, and can be similarly extended to EBSLN identification. Application of IONM should allow stimulation and identification of all EBSLNs including those visually unidentifiable subfascial EBSLNs mentioned earlier. Recently published guidelines on EBSLN monitoring emphasize that SLN monitoring is associated with higher rate of nerve identification as compared to visual identification alone [5]. The SLN monitoring utilizes the laryngeal head of the sternothyroid muscle as a useful landmark for identification of the EBSLN. The tissue parallel and underneath the laryngeal head of the sternothyroid muscle is stimulated to delineate distal course of EBSLN before it enters the cricothyroid muscle. A twitch in the cricothyroid muscle seen upon this stimulation is presently the most accurate way of EBSLN localization. For detailed review of technique and utility of EBSLN monitoring, the reader is requested to refer to the guidelines published by the INMSG [5]. It has been recently demonstrated that a specially designed electrode array incorporated on an endotracheal tube can facilitate quantifiable EMG response from the EBSLN in all patients [68].
18.3.3 Neural Monitoring and Staged Thyroidectomy in Thyroid Cancer Surgery: An Emerging Concept
The Concept of staging oncology surgeries has a longstanding history. Staged surgery was recommended by Dunhill, for severe toxic goiter in 1912 and by Lahey for severe hyperthyroidism in 1936 [69, 70]. Frazzell, in 1961, proposed a planned staging of surgeries where sacrifice of bilateral internal jugular veins was anticipated [71]. Extensive neck surgeries typically carry a higher risk of complications, and bilateral RLN paralysis being one of the most dreadful of all. Extensive neck surgeries are often necessary to address frequently encountered bilateral nodal metastases in thyroid cancers. By staging these surgeries, a surgeon may offset some of the associated complications, especially the temporary ones. RLN neuropraxia as well as parathyroid glands may recover functionally in the intervening period between the two stages of the surgeries.
While the aforementioned staged surgeries are typically planned preoperatively, often a surgeon intraoperatively faces a dilemma of proceeding to bilateral surgery or of staging the opposite side surgery. Usefulness of LOS as an intraoperative decision-making tool in such circumstances is slowly becoming evident. Dralle et al. found that 94 % of surgeons in Germany would stage a total thyroidectomy upon encountering a LOS during the surgery [72]. Goretski et al. have reported that when LOS was integrated in surgical decision making in bilateral surgeries, incidence of bilateral vocal cord paralysis reduced to zero from 17 % [73]. A surgeon should be aware that a detailed preoperative consent process is essential if staging of surgery is being envisioned as a possibility [74]. Our unit routinely performs staged surgery when patients present with extensive thyroid cancer with bilateral nodal disease. Our unpublished data from a series of patients undergoing planned staged surgery for advanced thyroid malignancy, reported zero percent permanent RLN paralysis and 3.3 % permanent hypoparathyroidism rate. Postoperatively thyroglobulin was undetectable in 90 % and averaged 0.8 ng/ml during a three-year follow-up.
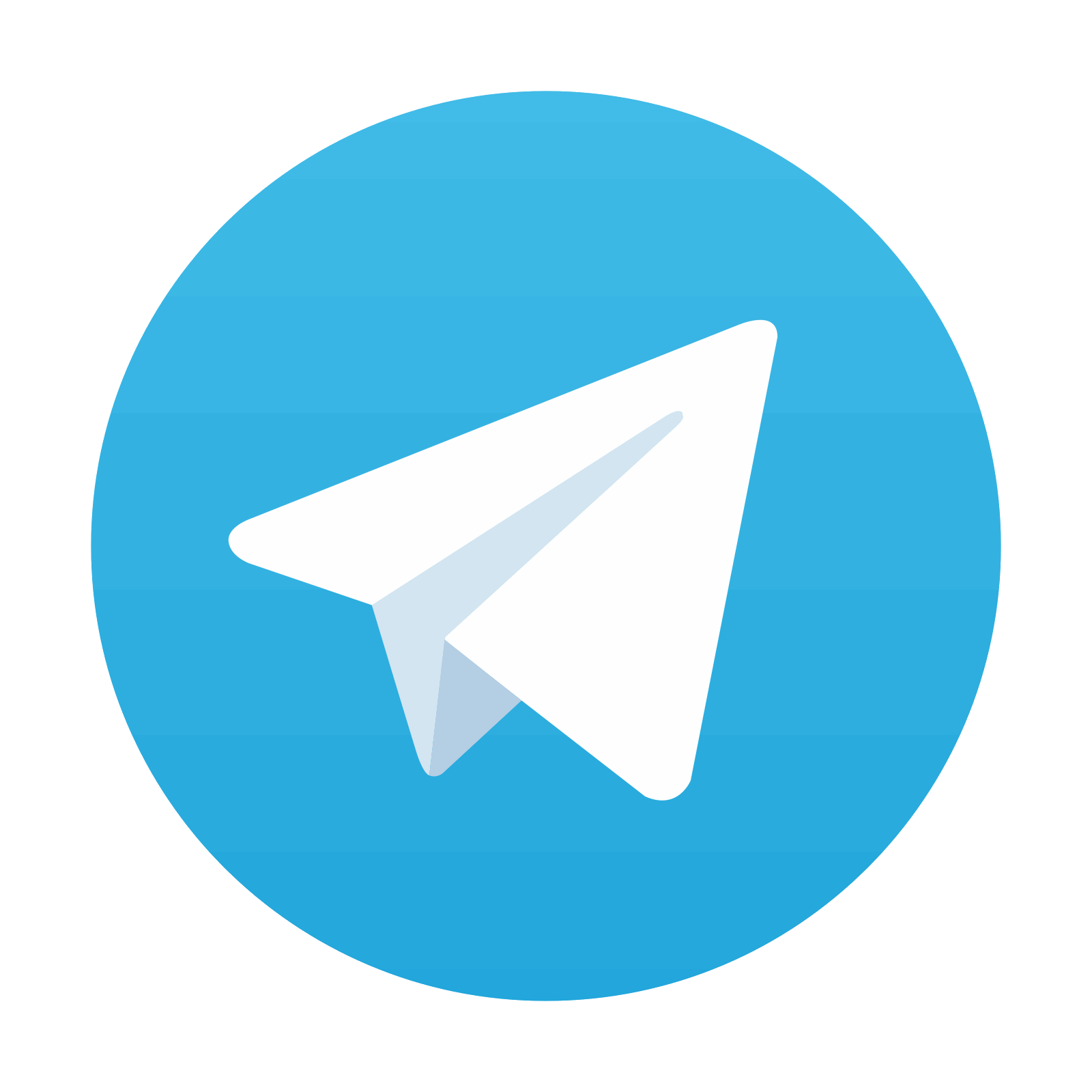
Stay updated, free articles. Join our Telegram channel

Full access? Get Clinical Tree
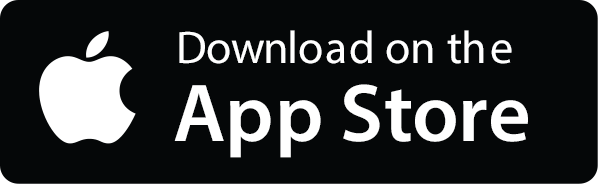
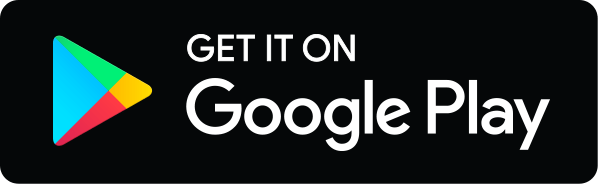