© Springer-Verlag Berlin Heidelberg 2015
Nima Rezaei (ed.)Cancer Immunology10.1007/978-3-662-44946-2_1818. Recent Advances in the Use of NK Cells Against Cancer
(1)
Department of Pathology and Molecular Medicine, McMaster Immunology Research Center (MIRC), McMaster University, Hamilton, ON, L8S 4K1, Canada
Keywords
NK cellsInnate immunityCancerImmunotherapyIL-15NK cell therapyEx vivo NK cell expansion18.1 Introduction
In the recent past, cancer immunotherapy was focused on adaptive immune cells such as CD8+ T cells and their antitumor cytotoxic capabilities. More recently, due to increased understanding of the biology and function of innate immune cells in tumors as well as technical advances, natural killer (NK) cells have emerged as an exciting new option for targeting tumor cells. In this chapter, we will introduce the important facts about NK cells that are required to understand their function in the tumor microenvironment and will proceed to recent clinical studies utilizing NK cells to fight cancer. Cancer immunotherapy using NK cells is progressing rapidly, and initial results, both preclinical and clinical, are very promising.
18.2 NK Cell Basics
NK cells are lymphocytes of the innate immune system, well known for their role in immunosurveillance and defense against virally infected or malignant cells. NK cells complement T cell immunity in their ability to recognize transformed cells without prior sensitization [1]. Human NK cells can be defined by their expression of the cell surface marker CD56. CD56bright NK cells are referred to as the immunoregulatory subset and precede the CD56dim subset in maturity [2, 3]. The CD56dim population represents the majority of NK cells in peripheral blood (90 %), and this subset is highly cytotoxic. Overall, NK cells make up 10–15 % of peripheral blood mononuclear cells (PBMCs) in the circulation [4]. From the circulation, they are able to extravasate into inflammatory peripheral sites containing malignant cells.
18.2.1 How Do NK Cells Become Activated to Kill?
Once in contact with malignant cells, NK cells can be activated to kill tumor cells through several different mechanisms. Cytokine activation of NK cells requires priming from factors such as interleukin-15 (IL-15), an important cytokine in the survival, development, and activation of NK cells [5–7]. Several other cytokines are also well known to activate NK cells including IL-2 and IL-12 [8]. In addition to cytokines, NK cell activation is regulated by the expression of activating or inhibitory receptors present on the NK cell’s surface. Whether or not an NK cell kills its target is determined by the balance of these receptors and the density of their corresponding ligands. NK cells kill target cells which lack inhibitory ligands, such as MHC class I molecules, on their cell surface. In this way, it is ensured that NK cells do not harm healthy cells which express MHC I but only those in which MHC I has been downregulated [9]. In humans, the two main groups of inhibitory receptors include the killer immunoglobulin receptors (KIRs) which bind to HLA class I and CD94-NKG2A/B, which recognizes HLA-E [10]. The loss of a single MHC class I allele can lead to the induction of NK cell lysis of tumor cell targets, a process which is known as “missing self” NK cell activation [11]. Unlike what was initially thought, NK cells are capable of overcoming the inhibitory signals delivered by MHC class I molecules by recognizing activating ligands upregulated on target cells. In general, activating ligands are not expressed on untransformed cells to prevent autoimmunity. However, when cells become transformed, stress caused by DNA damage can upregulate activating ligands, causing the cell to become a target for NK cell destruction [12]. This type of NK cell activation is known as “stress-induced self” activation [11]. A well-known example of an NK cell activating receptor is NKG2D. The ligands for NKG2D, which include MHC class I polypeptide-related sequence A and B (MICA and MICB) are stress-inducible proteins [11]. The DNA damage response, which occurs during tumorigenesis, causes the upregulation of these ligands, relaying signals to the NK cell to cause tumor cell destruction. Another important group of NK cell activating receptors is the natural cytotoxicity receptors (NCRs). This family includes the receptors NKp44 and NKp46, of which the corresponding ligands on tumor cells have yet to be discovered [11].
Upon activation, NK cells are able to kill tumor cells directly through the release of cytotoxic granules containing perforin and granzyme, through antibody-dependent cellular cytotoxicity (ADCC) and death receptor ligands on their surface such as TNF-related apoptosis-inducing ligand (TRAIL) and Fas ligand [1]. ADCC is a mechanism which results in the destruction of antibody-coated cells by NK cells [13]. NK cells express the FCγRIII (also known as CD16) which binds to the Fc portion of IgG on target cells and causes cell lysis. TRAIL and Fas ligand also bind to their corresponding receptors on tumor cells and cause cell death. Activation of NK cells can also cause the release of IFN-γ, a critical cytokine for tumor control. IFN-γ acts indirectly to induce type I immune responses in the surrounding environment as well as directly on cancer cells themselves [10]. The direct mechanism of IFN-γ on cancer cells still remains to be determined.
18.2.2 Why Should NK Cells Be Targeted as Anticancer Agents?
The supporting evidence which demonstrates that NK cells play an important role as anticancer agents comes from both mouse and human research. Using transgenic mouse models that lack NK cells or their activation receptors, it was revealed that these cell types are vital in cancer immunosurveillance [14]. For instance, in a model of spontaneous epithelial and lymphoid malignancy, the absence of the NK cell activating receptor NKG2D resulted in defective tumor surveillance and an increase in tumor growth [15]. The importance of NK cells in early tumorigenesis was also shown in a Her2/neu transgenic mouse model generated on a perforin-deficient background [16]. In this model, NK cells and perforin reduced the onset and number of mammary tumors growing in the Her2/neu model.
In humans, the importance of NK cells in tumor surveillance is mostly derived from correlative studies [8]. For instance, in an 11-year follow-up study, it was found that low NK cell cytotoxicity in peripheral blood lymphocytes correlated with an increase in cancer risk [17]. In addition, the presence of NK cells within several difference cancers, including squamous cell lung cancer, gastric cancer, and colorectal cancer, has been shown to be a positive prognostic factor for these patients [18–20]. It has also been found that not only can NK cells kill many human cancer cell lines they are also capable of killing human melanoma cells that have the characteristics of cancer stem cells [21]. From these studies, it is clear that there is a correlation between the presence of NK cells in a tumor and a positive clinical benefit for cancer patients and that NK cells have the potential to kill parts of tumors resistant to other therapies. However, it has also become evident that not only is the presence of NK cells important but their phenotype and functional status is equally significant to net clinical outcome.
18.3 Challenges Involved in Targeting NK Cells
The importance of NK cells in controlling cancer growth has been clearly defined. However, scientists face many challenges when targeting NK cells in the fight against cancer because tumors develop a slew of different strategies to avoid NK cell attack. Some of these challenges include low NK cell numbers and altered homing into malignant tissues as well as low NK cell activity in cancer patients. Despite the many challenges involved in targeting NK cells to efficiently kill tumor cells, novel immunotherapeutic strategies which may overcome these obstacles are under investigation.
18.3.1 How Many NK Cells Are in Cancer Patients and Tumors?
A major challenge in the study of intratumoral NK cells has been that very limited numbers of NK cells can be detected and extracted within established tumors [22]. This is consistent with research that has demonstrated that NK cells are decreased in a variety of different cancer patients including head and neck cancer, breast cancer, and chronic myelogenous leukemia [23, 24]. The low numbers of NK cells observed have been linked to a mechanism of spontaneous NK cell apoptosis in the circulation of these patients, particularly in the CD56dim population. CD56dim NK cells are defined as having preferential homing abilities for inflammatory sites; therefore, an increase in apoptosis in this population would greatly decrease the ability of NK cells to accumulate within tumors and contribute to tumor cell elimination [3]. As the number of NK cells decrease with tumor growth, cytotoxicity and cytokine secretion are reduced as well. In addition, the ability of these NK cells to interact with and activate other innate and adaptive immune cells within the tumor is lost.
In animal studies, tumor growth has been linked to decreased lymphopoiesis, which results in a reduction in overall NK cell numbers [25]. In addition to overall low NK cell numbers, distant tumor growth has been found to have significant effects on NK cell maturation [26]. NK cells from mice challenged with several tumor lineages have been shown to undergo a maturation arrest in the bone marrow leading to a decrease in mature, functional NK cells that can produce IFN-γ in the periphery. In human studies, it has been shown that advanced breast cancer patients have an increased proportion of immature NK cell subsets in their peripheral blood [3]. Similar findings were found in patients with non-small cell lung carcinoma (NSCLC), where a majority of tumor-infiltrating NK cells had a CD11b-CD27- phenotype, indicative of inactive and immature cells [27]. Interestingly, the presence of these immature NK cells had an impact on clinical outcome for NSCLC patients, as the frequency of these cells correlated with increasing tumor stage and size. These studies stress that a deeper understanding of the ability of tumors to alter the NK cell educational process in cancer patients is required. This knowledge will be crucial to effectively utilizing these cells for future immunotherapies.
Low numbers of NK cells in tumor samples from cancer patients can also be attributed to inefficient homing of the NK cells to malignant tissues [28]. This is particularly evident in patients with large solid tumors, where NK cell therapy represents an extraordinary challenge. In these patients, it is very difficult to adoptively transfer or activate enough NK cells to home to one or multiple tumors and impart meaningful effects on tumor growth [13]. There is a greater chance of directing NK cells to malignant tissues in patients with minimal disease or those that have already undergone surgery or chemotherapy to eliminate any residual tumor cells [13]. The goal of any NK cell cancer immunotherapy should involve two points: to increase the number of NK cells in malignant tissues and to activate them to a sufficient level so that they can suppress tumor growth.
18.3.2 What Is the Functionality of NK Cells in Tumors?
It has also become apparent from clinical evidence that the activity of NK cells from cancer patients is greatly reduced. There are multiple mechanisms in place which fully activate NK cells toward tumor cell destruction. In addition to recognizing cells which lack MHC class I, NK cells require multiple stimulatory signals to achieve maximal responses. These include the co-activation of various activating receptors present on NK cells with their corresponding ligands on the surface of tumor cells [13]. However, NK cells from human tumors have a reduction in the expression of activating receptors. Instead, these altered NK cells have an increase in the expression of inhibitory receptors – known to reduce NK cell activity. For instance, the progression of human breast cancer has been associated with a reduction in the function of tumor-infiltrating NK cells in comparison to peripheral blood NK cells [22]. Tumor-infiltrating NK cells were found to display a decrease in the expression of activating NK cell receptors (such as NKp30, NKG2D, DNAM-1, and CD16) and an increase in inhibitory receptors (such as NKG2A). Importantly, the NK cells displaying this altered phenotype had reduced cytotoxic capabilities. This altered NK cell phenotype has also been described in patients with NSCLC, where the local tumor microenvironment drastically impairs the ability of NK cells to degranulate and produce IFN-γ, rendering them less tumoricidal and indirectly supportive to cancer growth [29]. Similarly, in another study on NSCLC, the majority of NK cells infiltrating the tumor displayed a CD56bright phenotype and were less capable of tumor cell killing compared to peripheral blood or normal lung tissue NK cells [30]. Defective expression of activating receptors has also been a hallmark of metastatic melanoma [31] and acute myeloid leukemia (AML) [32] suggesting that this altered phenotype is a common feature of the antitumor immune response. If novel NK cell immunotherapies are to achieve clinical responses in patients, they have to find a way to increase the expression and maintenance of activating receptors on NK cells at the tumor site.
Why is it that when NK cells arrive at the tumor site, they lose their activity? Like all other immune cells, NK cells can change their characteristics based on the factors present within their environment. Within human tumors, NK cell inhibition can be mediated by interactions with neoplastic cells, T-regulatory cells, myeloid cells, or stromal cells [33]. Each of these cell types can express or release inhibitory factors, which can have profound effects on NK cell activity. For instance, the immunosuppressive cytokine TGF-β has been found to inhibit the expression of activating receptors NKp30 and NKG2D on human NK cells, thereby decreasing their killing ability [34]. TGF-β levels are often found to be elevated in cancer patients, including lung and colorectal cancer patients, and this is associated with a weakened NK cell immune response [35]. It was previously found that an inverse correlation exists between NK cell activation and T-regulatory cell expansion in tumor-bearing patients [36]. These findings were explained by a mechanism linked to the expression of membrane-bound TGF-β on T-regulatory cells causing direct inhibition of NK cell effector functions and NKG2D expression. This data suggests that minimizing T-regulatory cell numbers or the levels of TGF-β in the tumor could constitute a novel way to activate NK cells. PGE2, a small lipid molecule, has also been found to modulate NK cell antitumor responses. It has been demonstrated that PGE2 directly suppresses cytotoxicity and IFN-γ production by human NK cells [37]. Furthermore, the tryptophan catabolite, L-kynurenine, generated by the enzyme indoleamine 2,3-dioxygenase (IDO) has immunomodulatory properties which can have drastic effects on NK cells. L-kynurenine can interfere with the cytokine-induced upregulation of NKp46 and NKG2D, thereby modulating NK cell cytotoxic capacity [38].
In addition to being suppressed by factors within their environment, NK cells themselves can also upregulate immunoregulatory molecules such as programmed cell death-1 (PD-1). In a human study, it was found that NK cells from multiple myeloma (MM) patients expressed increased levels of PD-1 compared to healthy donor NK cells [39]. The direct interaction between PD-1 on NK cells and its corresponding ligand PD-L1 on tumor cells resulted in reduced NK cell function against MM tumor targets [39]. These examples allude to the fact that the most promising therapeutic approaches will involve combination therapies which include the activation of endogenous or adoptively transferred NK cells with removal of the suppressive signals that inhibit them.
As there is abundant evidence of an altered intratumoral NK cell state, it was hypothesized that these altered NK cells induce a unique gene expression signature distinct from NK cells found in healthy tissues. To examine this idea, researchers flow sorted NK cells isolated from non-tumoral and tumoral lung tissues from NSCLC patients and used microarray analysis to determine gene expression changes [40]. It was found that intratumoral NK cells have a unique transcriptional signature induced by the tumor microenvironment. This transcriptional signature suggests that NK cells which initially arrive at the tumor site become activated and then eventually exhausted after tumor cell recognition. In addition to an altered gene expression state, new evidence is arising which promotes the idea that NK cells are not only nonfunctional within tumors but that they might be able to support tumor growth through the release of pro-angiogenic factors. Tumors from patients with NSCLC were isolated and analyzed for their expression of pro-angiogenic factors [41]. Flow cytometric analysis of NK cells from these tumors revealed that these cells produced vascular endothelial growth factors (VEGF), placental growth factor (PGF), and interleukin-8 (IL-8). Induction of pro-angiogenic factors was mediated by TGF-β, as exposure to the immunosuppressive cytokine caused upregulation of VEGF and PIGF in NK cells from healthy subjects. Further research into the pro-angiogenic phenotype of NK cells and the impact they have on tumorigenesis are needed in other cancer types.
18.4 Cancer Immunotherapies Involving NK Cells
As outlined, there is extensive evidence that NK cells are capable of killing tumor cells both in animal models and in human studies. This has led to a high degree of interest in using NK cells as an immunotherapy over the last 20 years. While there have been many disappointing results and challenges, there are also many studies that indicate we are finally gaining enough knowledge about NK cells to design trials with much higher levels of success. Herein, the historical journey of NK cell-related immunotherapy will be outlined followed by the newest and most exciting studies in the field. Since cancer patients lack high numbers of NK cells and possess poorly activated NK cells, a natural idea to remedy this would be to transfer activated NK cells to them. One of the largest barriers to successful therapy with NK cells has been the production of large numbers of activated cells. Thus, the technological advances that are and will be extremely important for the area of adoptive cell transfer (autologous and allogeneic) will be discussed. In addition, the role of NK cells in monoclonal antibody (mAb) therapies and the status of systemic cytokine treatments to increase NK cell responses will be addressed.
18.5 Adoptive NK Cell Transfer
18.5.1 How Can We Produce Large Numbers of Activated NK Cells?
The main barrier to performing large clinical trials involving NK cell adoptive transfer has been the ability to produce large numbers of activated NK cells under good manufacturing practice (GMP) conditions. NK cells do not grow easily in culture and it has been difficult to produce large numbers of them. Different sources have been used to grow NK cells including the most common, human PBMCs (patient or donor derived), as well as NK cells derived from umbilical cord blood (UCB) or human stem cells. New knowledge regarding NK cell survival, proliferation, and activation has been employed to expand NK cells to the highest numbers possible while still ensuring that they possess a phenotype capable of killing tumor cells. In addition, advances in technology have allowed the upscaling of production. Multiple studies have been published over the last 10 years. These can be subgrouped into those involving cytokines, feeder cell lines, or artificial antigen-presenting cells (aAPCs).
Cytokines such as IL-2 and IL-15 have long been known to support NK cell proliferation, survival, and/or activation [5–7, 42, 43]. Thus, they were a natural starting point for this technology. Klingemann and Martinson [44] published an early study in which lymphocytes were isolated from PBMCs and underwent CD56 positive selection via magnetic bead technology [44]. Cells were then cultured in the presence of IL-2 or IL-2+IL-15. While there was expansion during the second week, it was variable and high levels of CD3+CD56+ NKT cells were produced. While the cells in the IL-2/IL-15 combination treatment were highly cytotoxic, the NK cells produced were mostly CD16 negative [44]. Another group performed a similar protocol, in which CD3+ cells were removed and the remaining cells were cultured overnight with IL-2 [45]. While these initial studies were a good starting point, they were limited by the poor expansion capability of NK cells under these conditions.
Further advancement in the field came with the addition of irradiated feeder cells to the protocols. In the majority of these studies, NK cells were isolated from PBMCs via immunomagnetic bead treatment to deplete CD3+ cells and enrich CD56+ cells. The cells were then subsequently cultured with irradiated feeder cells at a ratio of 1:10 (NK:feeder). In two similar studies, NK cells were purified from PBMCs via this method, and the immune cells that remained after selection were irradiated and cultured with NK cells [46, 47]. In addition, the cytokines IL-2 ± IL-15 and an anti-CD3 mAb (OKT3) were added. After 2–3 weeks, the cells were harvested and had expanded between 117- and 300-fold [46, 47]. The clinical potential of this method was demonstrated in a recent study that utilized patient NK cells to mimic an autologous transplant setting and then used either patient feeder cells or donor feeder cells to stimulate NK cells [47]. Patient NK cells incubated with healthy donor feeder cells were able to expand more and had increased purity (93.8 % CD56+CD3−) [47]. Another variant of this method is the use of allogeneic irradiated feeder cell lines. For example, Berg et al. utilized an irradiated Epstein-Barr virus (EBV)-transformed lymphoblastoid cell line as feeder cells to expand NK cells (with the addition of IL-2) [48]. After 28 days of culture, the NK cells expanded 300–1,000-fold and had high cytotoxicity [48].
An alternate feeder cell line that has been used frequently in GMP manufacturing of NK cells is a variant of the K562 cell line, which has been modified to express the membrane-bound form of IL-15 attached to the CD8α receptor and human 41BBL (K562-mbIL15-41BBL) [49–51]. When NK cells from either patients or healthy donors were cultured with irradiated K562-mbIL15-41BBL cells and IL-2, there was rapid expansion of the NK cells (in 7 days, expanded median 21.6-fold). After a final CD3+ depletion, NK cells had high levels of activation and were able to kill tumor cells in vitro and in a xenograft model [50]. While the success of these protocols was impressive, further modifications have been made to improve upon them. Gong et al. modified the K562-mbIL15-41BBL cells to also co-express MICA, an NKG2D-activating ligand [52]. After 24 days of culture with this feeder cell line, the NK cells expanded by 550-fold and had increased activation and cytotoxicity compared to those cultured with the original K562-mbIL15-41BBL cells [52]. Another breakthrough came recently in an attempt to optimize the signals that NK cells require ex vivo to propagate. In this case, a new K562-based cell line was created, termed an aAPC [53]. Researchers engineered the K562 cell line to express FcγRI, B7-2, and 41BBL and added either mbIL-15, mbIL-21, or both [53]. IL-21 is another gamma chain cytokine involved in NK cell proliferation [54]. When the irradiated K562 cell line that included mbIL-21 was cultured with PBMCs and IL-2 (no selection, 1:2 ratio PBMC:aAPCs) for 21 days, they expanded by 47,967-fold (825-fold expansion with the IL-15 construct) [53]. This level of expansion was higher than ever reported before for NK cells and was attributed to the fact that IL-21 signaling promotes an increase in telomere length and prevents the senescence that NK cells usually reach [53]. Not only were these cells highly cytotoxic they also had an increased ability to perform ADCC [53]. Others have also used these aAPCs to produce NK cells from human embryonic stem cells and induced pluripotent stem cells [55].
As can be imagined, the ability to grow large-scale cultures of NK cells in a GMP facility is also dependent on practical technologies. The methods currently used to grow NK cells include tissue culture flasks, cell culture bags, and bioreactors. A recent study attempted to expand NK cells in all three of these conditions and compare the resultant products [56]. Interestingly, the cells grown in the closed system or fully automated bioreactor were more cytotoxic than those grown in flasks and had higher NKp44 levels [56]. This method would be ideal if NK cell therapy becomes increasingly employed, as it is less labor intensive and can produce even higher levels of NK cells in a similar time frame. However, it might not be able to be used in all protocols, as certain NK expansion methods cannot be performed in a closed system.
Another major barrier to the large-scale use of NK cell adoptive therapy has been an inability to utilize frozen NK cells. Several recent reports using the previously mentioned expansion protocols have assessed the viability of these cells. Berg et al. found that expanded NK cells could be frozen and when thawed had decreased activating receptors and cytotoxicity. However, their activity could be restored with IL-2 treatment [48]. Others found that NK cells could be successfully expanded from frozen CD34+ umbilical cord blood samples [57]. Recently, it was reported that NK cells produced via the feeder cell line K562-mbIL15-41BBL or the aAPC K562-mbIL21 method could be frozen and still function well when thawed [51, 58]. These reports give hope that certain centers could produce expanded NK cells (either autologous or allogeneic) and ship them to smaller centers, allowing more patients the opportunity to receive these novel treatment options.
18.6 Autologous Transfer of NK Cells
The initial clinical trials involving NK cell transfer were autologous in nature and involved the use of IL-2 both in vivo and in vitro. These trials were based on the observation that IL-2-activated patient NK cells cultured with matched autologous melanoma cell lines demonstrated high cytotoxic activity [59]. In several phase I/II trials, patients were treated with IL-2 and their lymphocytes were subsequently harvested by leukapheresis. Patient lymphocytes were then cultured for several days in vitro with IL-2 before these lymphokine-activated killer (LAK) cells were reinfused back into the patient [60–64]. After LAK cells were infused into the patient, IL-2 was administered again systemically. Examination of the LAK cells revealed that the cells with cytotoxic activity against tumor cells were NK cells, not T cells [60]. These trials took place in patients with advanced colon, breast, lung, ovarian, pancreatic, renal cell, and melanoma cancers and overall had very disappointing results [61–64]. In addition, some reported treatment-related deaths due to high-dose IL-2 [62]. A few trials attempted to transfer autologous NK cells generated by IL-2 ex vivotreatment as a post autologous stem cell transplant treatment and found that although it was well tolerated and there was increased NK cytolytic function, there were no real clinical improvements for the patient [65, 66]. In a more recent trial, patients with metastatic melanoma and renal cell carcinoma (RCC) received autologous transfer of IL-2-activated NK cells after lymphodepletion [67]. In this trial, PBMCs were depleted of CD3 cells and the resultant cells were cultured with irradiated autologous PBMCs as feeder cells, IL-2, and OKT3 (and anti-CD3) for 21 days [67]. The IL-2-activated NK cells achieved high lytic activity in vitro; however, once the cells were transferred to the patients, no clinical responses were observed. In these patients, the expression of NKG2D on the transferred NK cells was lowered and the re-isolated NK cells could not lyse tumor cells in vitro unless they were restimulated with IL-2.
After these disappointing results, the field shifted gears and began to concentrate on allogeneic NK cell adoptive transfer, which will be discussed in the following. Nevertheless, researchers are still working on novel ways to increase clinical responses after autologous NK cell transfer. As further research was conducted on IL-2, it came to light that perhaps the use of this cytokine decreased the effectiveness of autologous NK cell therapy. While IL-2 activates NK cells, it has also been shown to increase T-regulatory cells in vivo, which, as mentioned, can negatively regulate antitumor NK cell responses [68, 69]. In fact, in an animal model of lung cancer, depletion of T-regulatory cells improved the outcome of NK cell adoptive transfer [70]. We will discuss the possibility of other cytokines to support NK cell activation in another section. Thus, researchers have started to employ new methods to expand NK cells, including aAPCs. A preclinical paper was recently published which utilized the K562-mbIL21 aAPC previously described [53, 58]. Researchers were able to expand NK cells from children with neuroblastoma by 2,363+/−443-fold. These cells expressed high levels of the activating receptors NKG2D and CD16 resulting in greater cytotoxicity against neuroblastoma cells lines as well as in a xenograft model of neuroblastoma [58]. If results could be translated into the clinic, they will provide new hope for the area of autologous NK cell transfer. There will likely be many more clinical studies published in the near future based on this platform.
18.7 Allogeneic Transfer of NK Cells
As mentioned, NK cells are negatively regulated by MHC I expression on target cells (KIR on NK cell and HLA class I allele on target cell). In 2002, Ruggeri et al. published a seminal study that revealed that this fact can be exploited [71]. If NK cells possessing a KIR that recognizes a particular HLA molecule are transferred into a host lacking that HLA allele, they will have increased cytotoxicity against cells lacking that particular HLA allele. This is known as donor vs. recipient NK cell alloreactivity [71]. For instance, 112 leukemia patients received a hematopoietic transplant with either KIR ligand incompatibility or not (from an HLA haplotype-mismatched family donor) [71]. It was found that receiving NK cells from an alloreactive donor increased 5-year event-free survival by 55 % over those who received nonalloreactive NK cells in AML [71]. It also simultaneously prevented graft-versus-host disease (GVHD) and decreased rejection [71]. This was a huge development in the field of adoptive NK cell therapy as it could explain some of the failures of autologous NK cell transfer. The next development was described in a non-transplant setting where allogeneic PBMCs were taken from haploidentical related donors, enriched for NK cells, and cultured overnight in IL-2 [45]. These were then infused into 19 poor prognosis AML patients after they underwent a high-dose immunosuppressive regime [45]. Remission was achieved in 5 of 19 patients and the NK cells expanded in vivo [45]. Success in these early studies led to a plethora of similar clinical trials both in hematological cancers [72–75] and solid tumors [73, 75–77]. While some early studies found success with enriched but not expanded alloreactive NK cells [72, 74], others at the phase II level proved non-beneficial [75]. There have been several preclinical studies using the newest methods of NK cell expansion (feeder cells lines – irradiated allogeneic PBMCs, K562-mbIL15-41BBL, the additive OKT3) and the testing of their efficacy in various solid tumor xenograft models [78–82]. For example, NK cells were transferred after their expansion with K562-mbIL15-41BBL into a xenograft model of myeloma. These NK cells were found to have high levels of activating receptors (NKG2D) and inhibited tumor growth and were found to still proliferate after a month in the tumor (with IL-2 systemic treatments) [79]. This study indicates that NK cells can persist in the host and remain active. Collectively, the results indicate that generating large numbers of activated NK cells with the latest techniques may be very useful and efficacious in future allogeneic NK cell adoptive transfers.
18.8 NK Cell Lines for Allogeneic Adoptive Transfer
The development of NK cell lines for adoptive transfer into cancer patients is a highly attractive option for its ease of use and its ability to expand NK cells to high numbers. The most established NK cell line used thus far has been the NK-92 line, which was established from a 50-year-old male with non-Hodgkin lymphoma [83]. This cell line is dependent on IL-2 for growth and is highly cytotoxic against tumor cell lines, primary tumor cells, and xenograft tumor models [83, 84]. The high cytotoxicity can be attributed to the lack of inhibitory KIRs on these NK cells [85]. This cell line has been approved for use in clinical trials, and a GMP method is available which can expand these cells by 200-fold in 2 weeks [86, 85]. In a phase I trial conducted on 12 patients with refractory RCC and melanoma, escalating doses of NK cells from 1 × 108 to 3 × 109/m2 were administered [87]. There was only mild toxicity at the highest dose and some responses (one mixed response, one partial response, one survived) [87]. New cell lines are also being established that have even higher levels of cytotoxicity than NK-92 to improve results in clinical trials [88]. Another benefit to an NK cell line is the ability to manipulate it genetically to improve its performance. Several recent studies have created NK-92 variants, such as a cell line that expresses a chimeric antigen receptor (CAR) which is the scFv fragment of a CD20-specific antibody connected to the CD3ζ chain to signal in the cell [89]. It is able to efficiently kill CD20+ targets normally resistant to NK killing [89]. Another NK-92 variant expresses a CAR that targets an antigen overexpressed in neuroblastoma called disialoganglioside [90]. This type of innovative NK cell line may be very useful in the future as the NK cells can be activated through regular mechanisms or via their new receptor. Genetic manipulation is not limited to NK cell lines as several reports have shown that NK cells isolated from PBMCs can also be manipulated to express CARs specific to HER-2 (overexpressed on many epithelial tumors) or to express chemokine receptors such as CCR7 to promote migration of the NK cells to the lymph node [91, 92]. Strategies targeting chemokine receptors on NK cells may be able to overcome inefficient homing of NK cells to tumors in certain cancer types. As these advances improve results in preclinical models, genetic manipulation may prove to be a powerful tool for NK cell therapies.
18.9 NK Cells, ADCC, and mAb Therapy
Multiple mAbs to tumor antigens have been approved for use in humans and have become a commonly used immunotherapy proven to be quite efficacious. Initially, the methods by which these mAbs worked were a hot area of debate. The mystery was partly solved when an important paper in the field showed that Fc receptors on either monocytes/macrophages, neutrophils, or NK cells were key molecules in the ability of mAbs to function against tumors [93]. Herceptin (trastuzumab-TZB) was unable to protect from Her2+ breast cancer cells in a xenograft model when Fc receptor γ was knocked out [93]. As mentioned, Fc Receptor γ is a key molecule involved in ADCC. Further studies revealed that NK cells express CD16 (FcγRIII), an activating receptor that binds to the Fc region of IgG1, and is able to trigger ADCC [94, 95]. Others have shown that in cancer cell lines resistant to NK cell killing, the addition of a mAb allows NK cells to perform ADCC on resistant tumor cells [96, 97]. After these studies were published, researchers began to view mAb treatment in a new light. They found that in patients that respond to TZB therapy, there are increased levels of NK cell activity and ADCC in comparison to those that do not respond [98]. In addition, they found that in both Rituxan (rituximab-RXB) and TZB mAB therapy, patients with certain polymorphisms in the FcγRII and FcγRIIIa had a better objective response rate and progression-free survival [99, 100]. This was also related to an increased ability of their PBMCs to kill tumor cell lines via ADCC [100]. Once the contribution of NK cells and ADCC to mAb therapy success became known, it opened up a whole new area of ways by which we may be able to improve upon its efficacy.
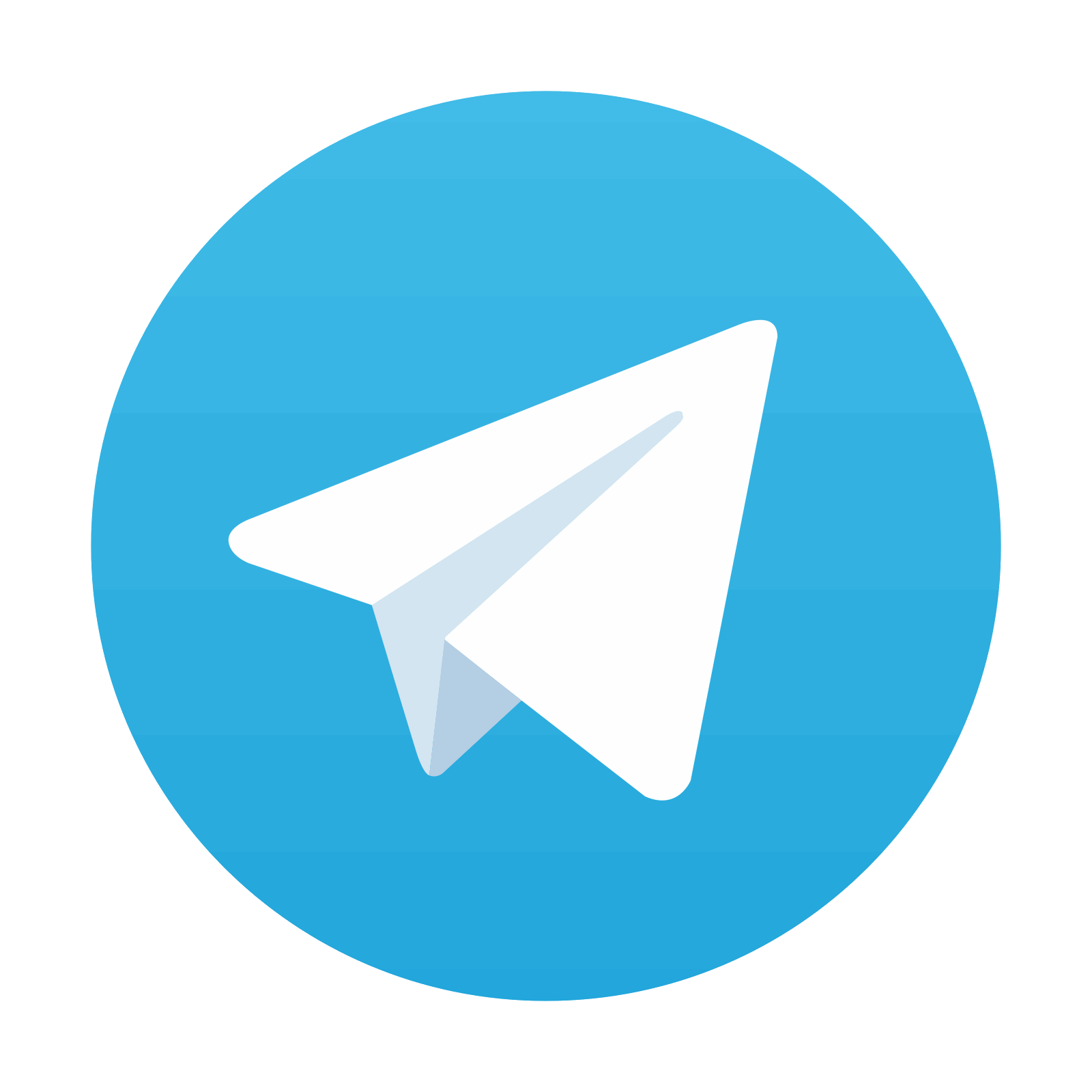
Stay updated, free articles. Join our Telegram channel

Full access? Get Clinical Tree
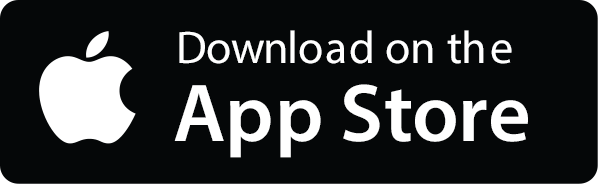
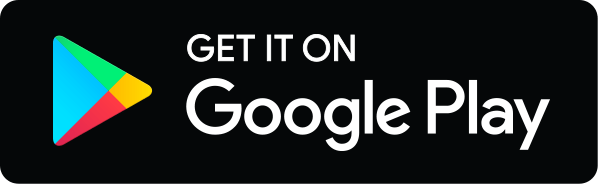