In this article, the application of radiotherapy, alone and in combination with surgery and chemotherapy, in the treatment of metastases to the brain (the most common malignant brain lesion), primary malignant gliomas (the most common malignant primary brain tumor), and metastases to the osseous spine is reviewed. Brain metastases may be treated with surgical resection, whole-brain radiotherapy, stereotactic radiosurgery, or some combination of these treatments. The optimum treatment of brain metastases is a matter of controversy, and patient and disease factors favoring one approach over another are presented.
Key points
- •
Options for the treatment of brain metastases include stereotactic radiosurgery, whole-brain radiotherapy, surgical resection, or some combination of these techniques, with the optimum therapy dependent on the size, number, and location of the brain lesions, the type and extent of primary tumor, and the patient’s performance status and preferences.
- •
The standard of care for malignant gliomas remains maximal safe resection followed by concurrent conformal radiotherapy and temozolomide.
- •
Metastatic lesions to the osseous spine compressing the spinal cord are optimally treated with decompressive surgery followed by radiotherapy—nonsurgical candidates should be considered for stereotactic radiosurgery or conventional external beam radiotherapy.
Introduction
Radiation therapy is an integral component of the management of primary and metastatic tumors of the central nervous system (CNS). In this article, the application of radiotherapy, alone and in combination with surgery and chemotherapy, in the treatment of metastases to the brain (the most common malignant brain lesion), malignant gliomas (the most common malignant primary brain tumor), and metastases to the osseous spine is reviewed. Minimizing radiation-induced damage to normal tissues is a fundamental objective in radiotherapy and it is a particular concern in the treatment of CNS lesions.
Introduction
Radiation therapy is an integral component of the management of primary and metastatic tumors of the central nervous system (CNS). In this article, the application of radiotherapy, alone and in combination with surgery and chemotherapy, in the treatment of metastases to the brain (the most common malignant brain lesion), malignant gliomas (the most common malignant primary brain tumor), and metastases to the osseous spine is reviewed. Minimizing radiation-induced damage to normal tissues is a fundamental objective in radiotherapy and it is a particular concern in the treatment of CNS lesions.
Brain tumors
Radiotherapy Techniques
Whole-brain radiotherapy
Radiation therapy to the entire brain (whole-brain radiotherapy [WBRT]) uses a relatively simple technique, typically using 2 parallel opposed lateral fields with the patient in the supine position. In preparation for planning and treatment, the patient is positioned on a head rest and immobilized using either a custom-molded thermoplastic mask or simply tape ( Fig. 1 A). Before treatment, radiographic or computed tomographic (CT) images are acquired and a treatment plan is developed using opposed megavoltage radiographic beams (see Fig. 1 B). The central axis of the beam is typically placed near the canthus to minimize the divergence of radiation beams into the eyes. In addition, the face and anterior eyes/lenses are blocked and the beam is shaped, using lead blocks or a multileaf collimator. At the time of treatment, the correct position of the treatment field is verified by either kilovoltage or megavoltage imaging of the treatment fields with the patient appropriately positioned on the radiation treatment table.

Intracranial stereotactic radiosurgery
In stereotactic radiosurgery (SRS) of brain lesions, a high dose of radiation is delivered in a single fraction or a few fractions with rapid dose falloff from the periphery of the target lesion into the surrounding normal brain tissue. Although a variety of disparate radiotherapy systems (eg, GammaKnife [Elekta, Stockholm, Sweden], CyberKnife [AccuRay, Sunnyvale, California], Novalis Tx [Varian Medical Systems, Palo Alto, California and BrainLAB, Munich, Germany]) are used in intracranial SRS, all share common features, as described in the attached consensus definition for radiosurgery ( Box 1 ). Radiosurgery systems demand and demonstrate exquisite accuracy (<1-mm deviation) for patient immobilization, target localization, and dose delivery. Typically, a patient is immobilized with either a semirigid, custom-molded, removable head mask ( Fig. 2 A) or a stereotactic head ring fixed to the patient’s skull. Target delineation is performed using fine-cut CT scans (∼1-mm slice thickness) fused with magnetic resonance imaging (MRI) images and occasionally functional imaging modalities, such as positron emission tomography. Intravenous contrast agents are often used to better define the target lesion.
- •
Stereotactic radiosurgery (SRS) uses externally generated ionizing radiation to inactivate/eradicate defined target(s) in the head or spine without the need to make an incision.
- •
The target is defined by high-resolution imaging.
- •
To assure quality care, the procedure involves a multidisciplinary team consisting of a neurosurgeon, radiation oncologist, and medical physicist.
- •
Although SRS typically is carried out in a single session, it can be administered in up to 5 sessions.
- •
SRS is performed using a rigidly attached stereotactic guiding device, other immobilization technology, and/or an image-guidance system.
- •
Technologies used to perform SRS include linear accelerators, particle beam accelerators, and multisource Cobalt 60 units.
- •
To enhance precision, these devices may incorporate robotics and real-time imaging.

In a collimator-based linear-accelerator system, such as the Novalis Tx, a typical treatment plan for an ellipsoid lesion, such as a small brain metastasis, consists of 3 to 5 non-coplanar conformal arcs (as illustrated in Fig. 2 B), yielding the conformal dose distribution shown in Fig. 2 C. Multiple intensity-modulated beams are often used for treating irregularly shaped targets and/or those that are intimately associated with critical organs. The correlation between the patient geometry (and/or immobilization device geometry) and the treatment machine geometry is achieved through 2 different approaches: (1) matching the geometry of the immobilization device with the machine isocenter through dedicated measurement devices with the assistance of room lasers; (2) matching planning/simulation images with treatment images (either 2D orthogonal images or 3D cone-beam CT images) acquired using an imaging device mounted in the treatment room (or machine) while patient is in the treatment position. Both approaches are able to achieve localization accuracy of about 1 mm. Radiation delivery consists of multiple beams intersecting at a single point (isocenter), shaped by fixed geometry cones and delivered in multiple arcs, dynamically conformal arcs continuously shaped by a multileaf collimator, or multiple intensity-modulated static beams or dynamic arcs. In the CyberKnife system, multiple collimated small-diameter beams are delivered to an intracranial lesion using a linear accelerator attached to a highly mobile robotic arm.
In contrast, the GammaKnife system uses hundreds of γ-ray sources (Co-60) precisely collimated to intersect at a single isocenter, yielding a small spherical or ellipsoid high-dose “cloud,” the diameter of which is determined by the size of the collimator opening. Dose falloff from the cloud into the surrounding tissue is extremely rapid. Treatment of irregular and/or large targets is achieved by “packing” together multiple dose clouds, positioned in the target by precisely repositioning the stereotactic headframe with respect to the machine isocenter.
Brain Metastases
Primary cancer metastatic to the brain (brain metastases) is the most common malignant lesion in the brain, developing in greater than 200,000 patients with cancer in the United States each year. Although brain metastases from lung, breast, kidney, and skin (melanoma) are most frequently encountered, virtually any histology from any anatomic site can metastasize to the brain. The optimal management of brain metastases is controversial, given the improved control of extracranial disease and increased longevity after the diagnosis of cancer; multiple tumor and patient factors influence prognosis, evolving patient expectations for treatment and a large number of treatment options. Treatment typically consists of WBRT, radiosurgery, surgical resection, or some combination of these modalities, as described in later discussion. Chemotherapy does not yet play a significant role in the management of brain metastases.
WBRT with and without surgery
WBRT is widely used for the treatment of patients with brain metastases. WBRT can temporarily halt the growth of brain metastases, gradually reducing mass effect and neurologic deficits and extending life. However, there is a substantial risk of recurrence and neurologic death, and WBRT is typically delivered with palliative intent. Survival following WBRT is quite variable and an analysis of Radiation Therapy Oncology Group (RTOG) trials involving 1200 patients with brain metastases suggested the important factors influencing survival. A technique termed Recursive Partitioning Analysis (RPA) was used to identify 3 RPA classes that predicted median overall survival (OS). The most favorable outcome (median OS 7.1 months) was observed in patients less than 65 years of age with well-controlled extracranial disease and a Karnofsky performance status ≥70 (RPA class 1). Patients with a Karnofsky performance status less than 70 were classified as RPA class 3 and demonstrated a median OS of 2.3 months. Patients who did not meet criteria in one of the above classes (RPA class 2) had a median OS of 4.2 months.
Acute side effects of WBRT include complete hair loss and mild scalp erythema and pruritus in nearly all patients, occasional sensation of fullness in the ears and parotid swelling, and mild anorexia and moderate fatigue, which can be severe in the debilitated and/or elderly patient. Steroids (primarily dexamethasone) should be given judiciously, using the lowest dose to control symptoms while carefully managing the many potential side effects. Prophylactic antiepileptic drugs should not be routinely administered. The long-term impact of WBRT on neurocognition and quality of life is a common concern of patients and their families. A frequently cited study of patients from Memorial Sloan-Kettering with single brain metastases treated with WBRT reported that “radiation-induced dementia” was observed in 5 of 47 patients at 1 year (11% crude rate). Four of the 5 patients who developed dementia were treated with a high dose per fraction (three 5- to 6-Gy fractions) and that the other received a concurrent radiosensitizer. In contrast, none of the 15 patients treated in ten 300-cGy fractions developed dementia. Typically, patients with brain metastases receive ten 300-cGy, fourteen to fifteen 250-cGy, or twenty 200-cGy fractions, although shorter and more protracted courses can be used. Although there are data from low-grade primary tumors suggesting that a more protracted course affords better preservation of neurocognition, the optimal dose/fractionation regimen for brain metastases has not been determined.
Studies of single brain metastases treated with WBRT with and without surgery have yielded conflicting results. For example, Patchell and colleagues’ randomized trial of WBRT alone versus surgery plus WBRT in 48 patients suggests that surgery be considered in all surgical candidates with a single resectable lesion. The rate of recurrence at the original site of metastasis was significantly lower in patients who were resected and irradiated (20 vs 52%, P <.02); overall survival was higher (40 vs 15 weeks, P <.01) and functional independence was longer (38 vs 8 weeks, P <.005) compared with those who received WBRT alone. In contrast, Mintz and colleagues’ randomized study of WBRT with and without surgery in 84 patients with single brain metastases showed no significant difference with the addition of surgery (5.6 vs 6.3 months).
Surgery with and without WBRT
Although surgical resection can alleviate the mass effect associated with a brain metastasis and substantially reduce the tumor burden, recurrence of disease at the resection cavity and in other areas of the brain occurs frequently. In a randomized trial by Patchell and colleagues of 95 patients with a solitary brain metastasis randomized to surgery with or without WBRT, recurrence of tumor anywhere in the brain was less frequent in the group receiving WBRT (18% vs 70%; P <.001). The addition of WBRT prevented brain recurrence at both the site of the original metastasis (10 vs 46%, P <.001) and other sites in the brain (14% vs 37%, P <.01). Patients in the group receiving WBRT were also less likely to die of neurologic causes (14% vs 44%, P = .003). However, the study showed no significant difference in overall survival with or without WBRT.
A subsequent study from the European Organization for Research and Treatment of Cancer (EORTC) randomized 359 patients with 1 to 3 brain metastases status either after surgery or after SRS observation versus WBRT. Of the 160 surgically resected patients, 79 were randomized to observation alone versus 81 to WBRT. The primary endpoint was deterioration in performance status. Similar to the Patchell study, recurrence at the resection site was significantly higher when WBRT was omitted (27 vs 59% at 2 years, P <.001, for the surgical resection group), as was distant recurrence in the brain (23 vs 42%, P <.008). Patients in the group receiving WBRT were less likely to die of intracranial progression (28 vs 44%, P <.002, for the overall group). However, the study found no difference in time to deterioration of performance status (10.0 months for observation alone vs 9.5 months with WBRT, P = .71) or in overall survival (10.7 months for observation alone vs 10.9 months with WBRT, P = .87).
WBRT with and without SRS
The RTOG randomized 333 adult patients with 1 to 3 brain metastases treated with WBRT to SRS within 1 week of completing WBRT versus observation (RTOG 9508 ). Of the 164 patients assigned to the SRS arm, 31 did not complete SRS with the principal reasons being refusal (9 patients) or disease progression/death (12 patients). Although local control was significantly improved in the group undergoing SRS (82 vs 71% at 1 year, P = .01), recurrent disease anywhere in the brain was not significantly better. Nonetheless, median overall survival was significantly higher with the addition of SRS in patients with a single brain metastasis (median overall survival time 6.5 vs 4.9 months, P = .039), patients less than 65 years old with controlled extracranial disease and Karnofsky performance status ≥70 (median overall survival time 11.6 vs 9.6 months, P = .045), and patients with brain metastasis ≥2 cm in greatest diameter ( P = .045 vs WBRT alone). In addition, patients treated with SRS exhibited significantly reduced steroid use and less deterioration in Karnofsky performance status than those who did not. Rates of acute and late toxicities were quite similar between the 2 groups, although SRS carried an approximate 0.5% monthly rate of radionecrosis.
SRS with or without WBRT
SRS alone with close follow-up to detect and treat recurrent disease has been suggested as an alternative to WBRT, as it potentially avoids neurocognitive and systemic sequelae encountered with treatment of the entire brain. On the other hand, omitting WBRT carries a significantly higher risk of recurrence, which in turn may result in increased neurocognitive deficits. Sneed and colleagues performed a retrospective analysis of 569 patients from 10 institutions treated with SRS alone versus SRS with up-front WBRT. There was no significant difference in the median overall survival time for patients receiving SRS alone versus SRS and WBRT for any RPA class, with the hazard ratio for overall survival of 1.09 ( P = .033) when adjusted for RPA class.
Aoyama and colleagues randomized 132 adult patients with 1 to 3 brain metastases to SRS alone versus SRS and WBRT. The addition of WBRT improved control both at the site of the original metastases (89 vs 73% at 1 year, P = .002) and at distant sites in the brain (58 vs 36% at 1 year, P = .003). However, overall survival was not significantly different in either arm, 7.5 months with SRS alone vs 8.0 months with SRS and WBRT, P = .42). As measured by mini-mental status examination, neurocognition did not differ between these arms, although mini-mental status examination is not a sensitive instrument for detecting changes in cognition.
Chang and colleagues conducted a trial of 58 patients with brain metastases randomized to SRS alone versus SRS and WBRT. The primary endpoint of the trial was neurocognitive decline measured by a comprehensive battery of tests. Four months after SRS, neurocognitive decline was substantially higher in the group receiving WBRT, 52% versus 24%. However, in contrast to most other studies, survival was substantially poorer in patients treated with WBRT alone, with median and 1-year overall survivals of 5.7 versus 15.2 months and 63% versus 21%, respectively ( P = .003). Similar to other studies, the 1-year freedom from recurrence anywhere in the brain was 27% for SRS alone versus 73% for SRS plus WBRT ( P = .0003). As the neurocognitive decline was measured at a time when many of the patients in the WBRT group were close to death, the results of this study are somewhat difficult to interpret.
SRS with surgery
Following resection of a single brain metastasis, the surgical cavity alone can be treated with radiosurgery, omitting whole-brain radiotherapy, with the objective of decreasing the high rates of local recurrence observed with surgery alone and avoiding the side effects of WBRT. For example, Choi and colleagues irradiated 120 resection cavities in 112 patients with brain metastases. At 1 year, the rate of recurrence at the cavity was 9.5%, whereas the rate of distant failure in the brain was 54%. They also examined the effect of irradiating only the resection cavity versus the resection cavity expanded by 2 mm and found that the rate of local failure at 1 year was significantly lower in the 2-mm group (3 vs 16%, P = .042). No significant difference in toxicity was observed as a function of resection margin.
Decision-making
All patients with brain metastases should be treated with some form of radiation therapy. In determining which patients should be treated with surgery before or after radiation, there are generally 6 features to consider, as follows: comorbidities, patient prognosis, size, location of metastasis, number of metastasis, and patient preference. In general, patients with comorbidities such that perioperative risk is extraordinary should be treated with radiation alone. In addition, patients with a poor prognosis because of fulminant metastatic disease or other comorbidities should also be treated with radiation only, as the time to recover from surgery is generally a minimum of 3 to 6 weeks and surgery imparts risks that may increase the recovery time. Given increasing tumor burden and decreasing maximum tolerated dose for radiosurgery as tumor diameter increases, many patients with tumors greater than 3 cm, and most patients with lesions less than 4 cm, should be considered for surgery. Similarly, the size and location of the mass also may suggest surgery. For example, a 3-cm mass in the frontal lobe can be safely treated with radiation alone, but a similarly sized lesion in the cerebellum may produce obstructive hydrocephalus; radiation without surgical decompression may worsen the hydrocephalus. It is also generally accepted that patients with a single metastasis or more than one metastasis that can all be accessed through a reasonably sized craniotomy should be considered for surgical resection. Finally, patient preference should always be taken into account given the lack of level I data to support specific recommendations, particularly with regard to the choice between surgery plus radiation versus radiation alone; this discussion must involve the neurosurgeon, radiation oncologist, and the patient.
Primary Brain Tumors
Malignant gliomas
Anaplastic astrocytomas and glioblastomas (World Health Organization grade III and IV malignant gliomas, respectively ) account for about two-thirds of primary malignant brain tumors in adults with an annual incidence in the United States of approximately 6 cases per 100,000 person-years. Although meningiomas are more frequently encountered, these are overwhelmingly benign tumors and the discussion in this section focuses on the management of the far more aggressive malignant gliomas. Malignant gliomas arise from neuroepithelial tissue with a peak incidence in the sixth decade of life. The cause of most cases of malignant gliomas is unknown in more than 90% of cases, although exposure to ionizing radiation and certain genetic syndromes are associated with an increased risk of this disease.
Surgery
Maximum safe resection of malignant glioma is a key element in the management of malignant gliomas, as outcomes seem to be more favorable in patients undergoing a gross or near total resection compared with minimal debulking or biopsy alone. However, there are no randomized control studies proving the superiority of a gross total resection and it is unlikely that such a trial would be performed. With surgery alone, median progression-free survival and overall survival are on the order of only a few months, as tumor cells are present well beyond the gross lesion. Thus, surgery and radiotherapy are typically both used, as described below.
Radiotherapy
Radiation therapy following surgical resection of malignant gliomas has been a recommended component of the management strategy since the 1970s, because it improves overall survival compared with surgery alone. At that time, the present standard total dose of 60-Gy radiation delivered in 1.8- to 2-Gy daily fractions was established. Trials to improve outcome by dose escalation using conventionally fractionated radiotherapy (RT), hyperfractionation, brachytherapy, or a stereotactic radiosurgery boost have not revealed a benefit to increasing dose beyond 60 Gy. For example, in RTOG 9305, 203 patients with glioblastoma were randomized to receive SRS versus no SRS before a course of conventional RT (a total of 60 Gy in 2-Gy daily fractions) and concurrent carmustine. No significant differences in survival (14.1 vs 13.7 months with or without SRS), neurocognition, quality of life, or patterns of failure were found, with 90% of failures occurring at the treatment field in both arms. Thus, standard RT typically consists of thirty or thirty-three 2.0- or 1.8-Gy daily fractions to a total dose of 59.4 to 60 Gy delivered over a 6-week to 7-week period. However, there is evidence in elderly patients that treatment at a slightly higher dose per day for a significantly shorter period (eg, 40 Gy delivered over 3 weeks) yields reasonable outcome.
Although the entire brain was initially irradiated due to the concern about the widespread and insidious distribution of tumor cells throughout the brain, various trials showed no significant differences in outcome when the volume of brain irradiated was reduced. Pattern-of-failure analyses show that most failures occur with 2 to 3 cm of the enhancing lesion visualized on CT are MRI scan and that a distant failure is almost always associated with a local failure. Thus, to avoid toxicity associated with whole-brain irradiation to 60 Gy, the current standard practice is to irradiate only the involved portion of the brain. Typically, the initial target for irradiation is the volume of brain exhibiting T2 hyperintensity on MRI expanded by 2 cm, and this target volume is treated to 45 to 46 Gy, which is followed by a “boost” of an additional 14 to 14.4 Gy to the contrast-enhancing residual lesion and/or resection cavity on T1-weighted MRI imaging, bringing the total dose to 59.4 to 60 Gy. To minimize the volume of normal brain irradiated and keep the dose to critical structures within tolerance limits, multiple shaped, intersecting radiation beams are used and intensity-modulated RT is often necessary to satisfy dose constraints.
Surgery, RT, and chemotherapy
Meta-analyses of the outcome in patients with malignant glioma treated with or without nitrosureas suggested a small but significant benefit from the addition of these intravenous agents. However, in 2005, Stupp and colleagues reported the results an EORTC trial that randomized 573 patients with glioblastoma receiving RT (60 Gy in 2-Gy daily fractions) with or without the oral alkylating agent, temozolomide (TMZ). TMZ was administered once daily during RT and then for 5 days each month for the next 6 months. The addition of TMZ was associated with significantly improved overall survival ( P <.0001), with median, 2-year overall survival, and 5-year overall survival of 15 versus 12 months, 27% versus 11%, and 10% versus 2%, respectively. Patients expressing lower levels of the enzyme responsible for repair of DNA damage, MGMT, exhibited a much more favorable response, although an improvement in survival was noted in the RT/TMZ arm even in those patients with unfavorable MGMT status. Likewise, although younger age, performance status, and increased extent of resection were associated with better outcome, the addition of TMZ conveyed improved survival in all prognostic groups. Consequently, the standard of care for adult patients with newly diagnosed glioblastomas includes maximum safe resection followed by conventionally fractionated RT with concurrent and adjuvant chemotherapy. This practice has been extended to cover all malignant gliomas, although data from randomized trials in anaplastic astrocytomas, for example, are absent.
Even with the optimum combination of surgery, RT, and chemotherapy, the typical prognosis in malignant gliomas remains poor with virtually all patients recurring and less than 10% surviving 5 years after diagnosis. A variety of novel approaches are under trial to improve outcome in newly diagnosed glioblastoma, including vaccine therapies and the use of anti-angiogenic agents. Of particular importance, a large phase III randomized trial from the RTOG (RTOG 0825) is examining the impact of anti-angiogenic bevacizumab (BVZ) on survival in newly diagnosed glioblastoma, when added to conventional regimen of RT and concurrent TMZ. Accrual is complete and the results are expected in the next few years. Given the extremely high rates of recurrence in malignant gliomas, improved treatment of recurrent disease is also a matter of great interest. BVZ has been approved by the Food and Drug Administration for the treatment of recurrent disease based on the results of phase II trials and SRS, alone or, particularly, in combination with BVZ, may offer benefits in this setting. However, randomized trials demonstrating the efficacy of SRS/BVZ have not been completed.
Normal tissue toxicity
The tolerance of normal CNS tissue to therapeutic radiation depends on a variety of factors, including the total radiation dose, dose/fraction, the size and location of the target lesion, and concomitant chemotherapy. A joint task force sponsored by the American Society of Radiation Oncology and American Association of Physics in Medicine recently published a comprehensive review quantifying normal tissue toxicity in clinical radiation oncology to a variety of organ systems, including the brain, brainstem, optic nerves/chiasm, hearing apparatus, and spinal cord.
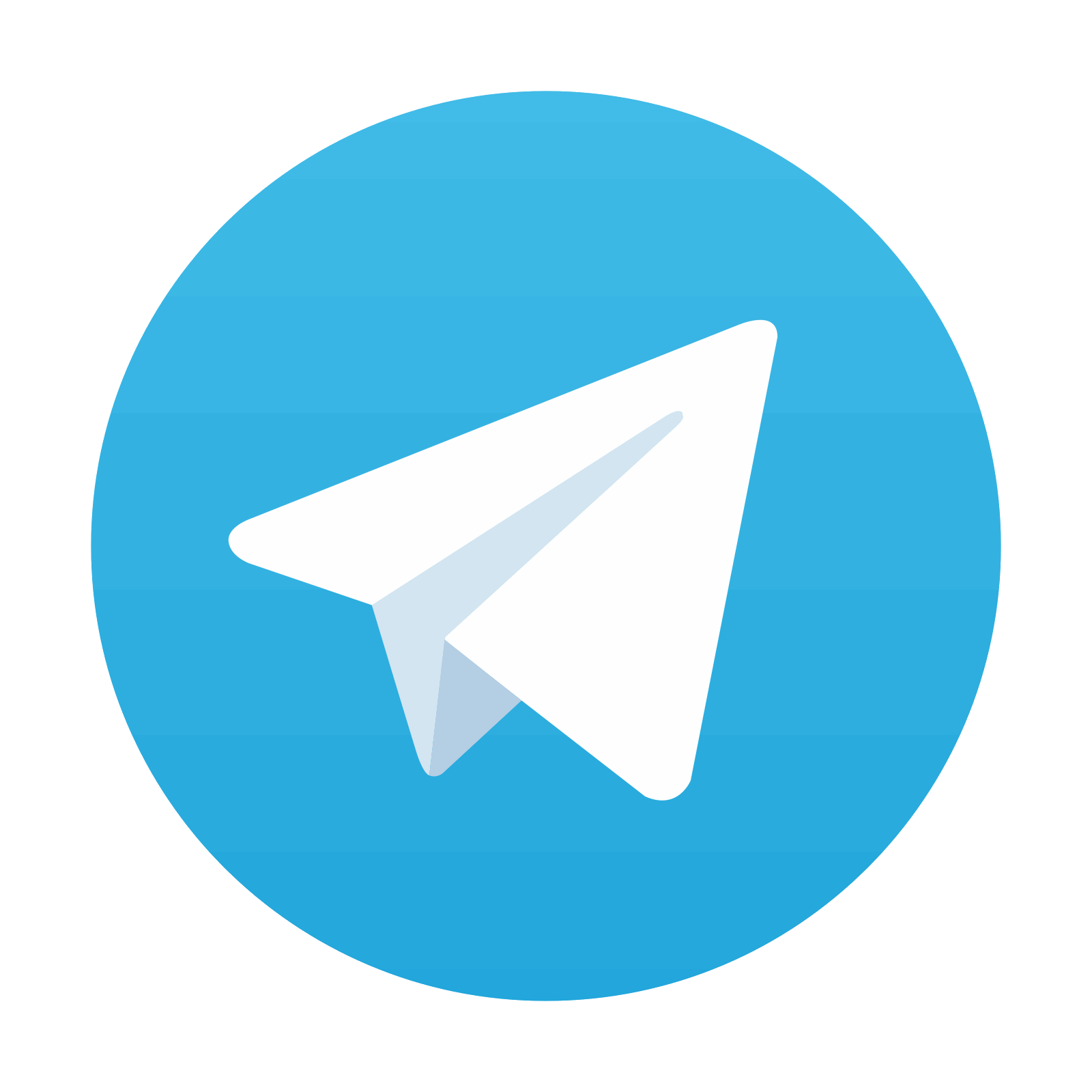
Stay updated, free articles. Join our Telegram channel

Full access? Get Clinical Tree
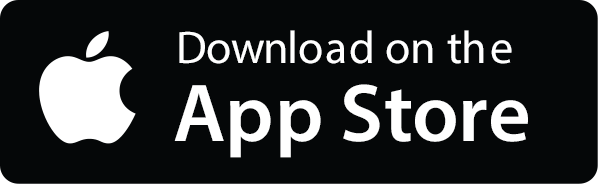
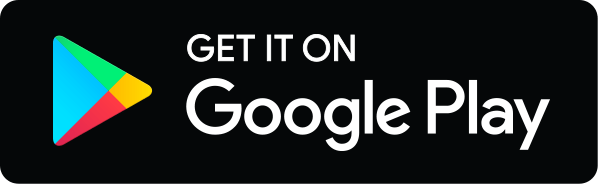