Radiation therapy plays a central and continuously evolving role in the treatment of head and neck cancer. In this review, some basic principles of radiation oncology are explained and common clinical scenarios are addressed in which radiation therapy forms part of the treatment course, with a focus on issues most pertinent to the interaction of radiation oncology with surgical treatment. These issues include refinement of the indications guiding the choice of adjuvant radiation therapy or chemoradiotherapy, sequencing and timing of therapies before or after definitive surgical management, and scientific and technical advances with the potential to reduce radiation-related toxicity.
Key points
- •
Intensity-modulated radiation therapy is now the dominant mode of head and neck radiotherapeutic treatment but is challenging because of its technical complexity.
- •
For definitive treatment of most advanced-stage head and neck cancers which are not treated with primary surgery, concurrent chemoradiation produces the greatest efficacy, at a cost of significantly increased toxicity over radiation therapy alone.
- •
For human papillomavirus–associated oropharyngeal cancers, active investigation into deintensification strategies is likely to reshape the landscape of treatment options over the next several years.
- •
The worst prognostic factors in the postoperative setting are positive margins of resection or nodal extracapsular extension, and even with adjuvant chemoradiation, rates of locoregional recurrence are high in these patients.
- •
Future developments in radiation therapy technology will require substantial capital investments, with the understanding that most of these technical improvements are likely to decrease toxicities rather than improve oncologic outcomes.
Introduction
Radiation therapy plays a central and continuously evolving role in the treatment of head and neck cancer. In part, the uses of radiation are varied because of the diversity of histology and natural history within subsites of the head and neck. Although the basic principles of radiation therapy have not changed over the past decades, a greater understanding of clinically applicable aspects of radiation biology and the wide availability of technologically based advances have contributed to the increasing sophistication of radiation-based treatment, with quantifiable benefits in improved tumor control and quality of life. In addition, ongoing discovery in surgical and systemic therapy are informing multimodality approaches involving radiation therapy.
In this review, some basic principles of radiation oncology are explained and common clinical scenarios are addressed in which radiation therapy forms part of the treatment course, with a focus on issues most pertinent to the interaction of radiation oncology with surgical treatment. These issues include refinement of the indications guiding the choice of adjuvant radiation therapy or chemoradiotherapy, sequencing and timing of therapies before or after definitive surgical management, and scientific and technical advances with the potential to reduce radiation-related toxicity.
Technical principles of modern head and neck radiation therapy
Ionizing radiation as known to most practitioners is a far remove from that delivered by the machines used in the 1950s and 1960s. Modern linear accelerators are capable of producing deeply penetrating photon beams that are shaped by high-attenuating tungsten collimation in space and over time, creating intricate three-dimensional (3D) distributions of the radiation dose. They may be imagined as dose clouds in concentric arrangements falling off to lesser and lesser dose levels.
Intensity-modulated radiation therapy (IMRT) is a photon-based ionizing radiation treatment. IMRT was developed to improve the conformality of the dose cloud to the shape of the tumor, sparing (avoiding) the normal tissues in proximity. The underlying concept is to modify the intensity of the radiation beam as it is delivered across and around the site of the cancer. This complex planning process requires computer assistance, and hence, IMRT is almost always inverse planned, meaning that instead of designing the apertures and then calculating the resulting doses (forward planning), the desired shapes of the dose clouds (called contours) are drawn by the radiation oncologist using a 3D computed tomography (CT) scan, and the computer defines the radiation beam angles to optimize the intensity modulation so that the resultant dose cloud mimics the contours to the highest degree possible.
IMRT found some of its earliest uses in prostate and head and neck cancer although its subsequent adoption in general radiation oncology practice was rapid. It is estimated that IMRT is used for greater than 85% of head and neck cancer treatments across the United States, and the prevalence is similar in other developed nations. IMRT is used more frequently in head and neck cancer cases than for any other major class of cancer.
This situation presents ongoing challenges, because of the complexity of IMRT. To specify the areas where the dose cloud should be delivered, the contours delineating the anatomic boundaries of the tumor mass and normal anatomic structures must be precisely drawn on the CT scan using for planning. The Hounsfield units of that CT scan are used to determine the density of the tissues (and thus to calculate the delivered dose to each small volume of the body). The radiation dose is specified in units of Gray (Gy), corresponding to 1 J of energy deposited per kilogram of material. Because of the anatomic expertise needed to delineate normal and abnormal structures on the CT scan, typically incorporating margins of error of a few to several millimeters, IMRT is an order of magnitude more demanding to prescribe, at least at the tumor delineation step, than less conformal techniques. IMRT-related quality assurance of the delivered treatments and the required machine maintenance is also more demanding.
However, IMRT is not the only form of radiotherapy suitable for head and neck cancers. For skin cancers or other superficially located tumors, low-penetrating photons (in the kilovoltage rather than megavoltage energy range) or electron therapy can be useful, and for well-circumscribed, physically accessible tumors, some large centers maintain an active practice of brachytherapy, or “short therapy,” in which radioactive wires or seeds are introduced directly into the intended treatment volume. Fig. 1 depicts these forms of radiation delivery.
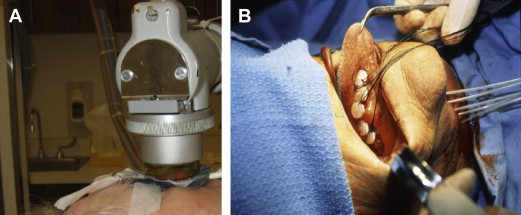
As another alternative to IMRT, traditional conformal techniques using less conformal two-dimensional (2D) radiograph-based forward planning (eg, anterior and posterior beams or opposed lateral beams) or 3D CT-based forward planning (using more beam angles to encircle the central target and achieve greater conformality) are highly effective if the normal tissues close to the treatment volume are able to tolerate high levels of radiation. Concomitant with their relative technical simplicity, these less conformal 2D or 3D techniques carry the burden of a heavier side effect profile, because more normal tissue is typically irradiated.
Fig. 2 shows the initial delineations that are considered basic requirements in designing an IMRT treatment. According to International Commission on Radiation Units and Measurements Report 50, the gross tumor volume (GTV) encompasses all disease that can be appreciated on physical examination or radiologic imaging. The clinical target volume (CTV) is the area considered to be at high risk for microscopic disease spread. The planning target volume (PTV) is an additional envelope around the CTV (to account for a few millimeters of daily patient setup and machine variance). The PTVs are fed into the computational algorithm that generates the beam angles and intensity-modulated segments that are delivered from the head of the linear accelerator machine ( Fig. 3 ).
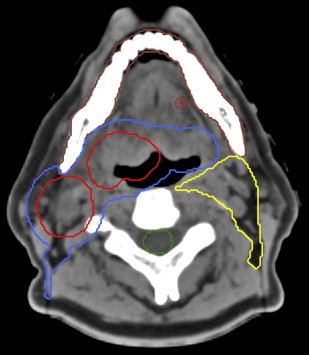
The prescribed standard dose to volumes encompassing the GTV is typically 7000 cGy over 6 to 7 weeks. For example, the recently concluded RTOG 1016 (Radiation Therapy Oncology Group 1016) protocol (a phase 3 randomized study of human papillomavirus [HPV]-associated oropharyngeal cancer) assigned, randomized patients to receive radiation therapy with either concurrent cisplatin or with concurrent cetuximab. The protocol prescription was 7000 cGy over 6 weeks, with a dose of 5600 cGy delivered to the areas of likely microscopic disease involvement and 5000 to 5250 cGy delivered to areas at very low risk for presence of tumor.
Introduction
Radiation therapy plays a central and continuously evolving role in the treatment of head and neck cancer. In part, the uses of radiation are varied because of the diversity of histology and natural history within subsites of the head and neck. Although the basic principles of radiation therapy have not changed over the past decades, a greater understanding of clinically applicable aspects of radiation biology and the wide availability of technologically based advances have contributed to the increasing sophistication of radiation-based treatment, with quantifiable benefits in improved tumor control and quality of life. In addition, ongoing discovery in surgical and systemic therapy are informing multimodality approaches involving radiation therapy.
In this review, some basic principles of radiation oncology are explained and common clinical scenarios are addressed in which radiation therapy forms part of the treatment course, with a focus on issues most pertinent to the interaction of radiation oncology with surgical treatment. These issues include refinement of the indications guiding the choice of adjuvant radiation therapy or chemoradiotherapy, sequencing and timing of therapies before or after definitive surgical management, and scientific and technical advances with the potential to reduce radiation-related toxicity.
Technical principles of modern head and neck radiation therapy
Ionizing radiation as known to most practitioners is a far remove from that delivered by the machines used in the 1950s and 1960s. Modern linear accelerators are capable of producing deeply penetrating photon beams that are shaped by high-attenuating tungsten collimation in space and over time, creating intricate three-dimensional (3D) distributions of the radiation dose. They may be imagined as dose clouds in concentric arrangements falling off to lesser and lesser dose levels.
Intensity-modulated radiation therapy (IMRT) is a photon-based ionizing radiation treatment. IMRT was developed to improve the conformality of the dose cloud to the shape of the tumor, sparing (avoiding) the normal tissues in proximity. The underlying concept is to modify the intensity of the radiation beam as it is delivered across and around the site of the cancer. This complex planning process requires computer assistance, and hence, IMRT is almost always inverse planned, meaning that instead of designing the apertures and then calculating the resulting doses (forward planning), the desired shapes of the dose clouds (called contours) are drawn by the radiation oncologist using a 3D computed tomography (CT) scan, and the computer defines the radiation beam angles to optimize the intensity modulation so that the resultant dose cloud mimics the contours to the highest degree possible.
IMRT found some of its earliest uses in prostate and head and neck cancer although its subsequent adoption in general radiation oncology practice was rapid. It is estimated that IMRT is used for greater than 85% of head and neck cancer treatments across the United States, and the prevalence is similar in other developed nations. IMRT is used more frequently in head and neck cancer cases than for any other major class of cancer.
This situation presents ongoing challenges, because of the complexity of IMRT. To specify the areas where the dose cloud should be delivered, the contours delineating the anatomic boundaries of the tumor mass and normal anatomic structures must be precisely drawn on the CT scan using for planning. The Hounsfield units of that CT scan are used to determine the density of the tissues (and thus to calculate the delivered dose to each small volume of the body). The radiation dose is specified in units of Gray (Gy), corresponding to 1 J of energy deposited per kilogram of material. Because of the anatomic expertise needed to delineate normal and abnormal structures on the CT scan, typically incorporating margins of error of a few to several millimeters, IMRT is an order of magnitude more demanding to prescribe, at least at the tumor delineation step, than less conformal techniques. IMRT-related quality assurance of the delivered treatments and the required machine maintenance is also more demanding.
However, IMRT is not the only form of radiotherapy suitable for head and neck cancers. For skin cancers or other superficially located tumors, low-penetrating photons (in the kilovoltage rather than megavoltage energy range) or electron therapy can be useful, and for well-circumscribed, physically accessible tumors, some large centers maintain an active practice of brachytherapy, or “short therapy,” in which radioactive wires or seeds are introduced directly into the intended treatment volume. Fig. 1 depicts these forms of radiation delivery.
As another alternative to IMRT, traditional conformal techniques using less conformal two-dimensional (2D) radiograph-based forward planning (eg, anterior and posterior beams or opposed lateral beams) or 3D CT-based forward planning (using more beam angles to encircle the central target and achieve greater conformality) are highly effective if the normal tissues close to the treatment volume are able to tolerate high levels of radiation. Concomitant with their relative technical simplicity, these less conformal 2D or 3D techniques carry the burden of a heavier side effect profile, because more normal tissue is typically irradiated.
Fig. 2 shows the initial delineations that are considered basic requirements in designing an IMRT treatment. According to International Commission on Radiation Units and Measurements Report 50, the gross tumor volume (GTV) encompasses all disease that can be appreciated on physical examination or radiologic imaging. The clinical target volume (CTV) is the area considered to be at high risk for microscopic disease spread. The planning target volume (PTV) is an additional envelope around the CTV (to account for a few millimeters of daily patient setup and machine variance). The PTVs are fed into the computational algorithm that generates the beam angles and intensity-modulated segments that are delivered from the head of the linear accelerator machine ( Fig. 3 ).
The prescribed standard dose to volumes encompassing the GTV is typically 7000 cGy over 6 to 7 weeks. For example, the recently concluded RTOG 1016 (Radiation Therapy Oncology Group 1016) protocol (a phase 3 randomized study of human papillomavirus [HPV]-associated oropharyngeal cancer) assigned, randomized patients to receive radiation therapy with either concurrent cisplatin or with concurrent cetuximab. The protocol prescription was 7000 cGy over 6 weeks, with a dose of 5600 cGy delivered to the areas of likely microscopic disease involvement and 5000 to 5250 cGy delivered to areas at very low risk for presence of tumor.
Clinical evidence in support of highly conformal therapy
For the most part, radiation oncologists have considerable faith in radiation physics; a rationally derived preferred dose distribution is considered acceptable evidence of clinical superiority. Despite this tendency to embrace technological superiority in the absence of clinically proven superiority, distinct lines of evidence support the improvements believed to result from the use of IMRT. A randomized study of patients with nasopharyngeal carcinoma showed a reduction from 82% to 39% in the rates of observer-rated severe xerostomia at 1 year in the patients who received IMRT with concurrent chemotherapy, compared with those treated similarly but with 2D radiation therapy (based on grading by clinicians according to the RTOG/European Organization for Research and Treatment of Cancer [EORTC] scale). Another trial in patients with early-stage nasopharyngeal carcinoma showed improvements in stimulated saliva flow rates as well as EORTC-scored quality of life at 1 year in patients treated with IMRT rather than 3D conformal radiation therapy.
It might be considered a problem that in the United States, expensive and technically demanding IMRT was adopted quickly and widely, without testing for which applications it would be best suited or when its advantages would be most profound over 3D radiation therapy. A multicenter randomized study of parotid-sparing IMRT (PARSPORT) was conducted in the United Kingdom, at a time when the implementation of IMRT was not yet universal. Thus, the PARSPORT trial was able to test these issues in a relatively technique-neutral environment. The study reported that at 12 and 24 months, IMRT produced statistically significant benefits in recovery of salivary secretion and in dry-mouth-specific and global quality of life scores.
The very long-term results of IMRT have yet to be fully appreciated in realms outside xerostomia, but generally, the side effect profile seems to be positive. A few institutional reports have reported lower risks of major radiation-related complications using IMRT, focusing most notably on a reduction in the rates of osteoradionecrosis. Other investigators have proposed deployment of IMRT to reduce dose to the swallowing structures, especially the pharyngeal constrictors, in an attempt to mitigate the long-term swallowing dysfunction associated with long-term radiation-induced fibrosis of these areas. When tumor anatomy is in close apposition to the mandible or pharyngeal constrictors, it is not possible to avoid those structures even with IMRT. Furthermore, the physics of IMRT require that when dose is shifted away from a designated high-priority critical structure, there may be a price to pay elsewhere. On the other hand, a few reports now associate IMRT with novel toxicities resulting from the beam path. These toxicities include nausea related to brainstem irradiation, alopecia of the posterior scalp, and oral cavity mucositis from beams passing through the mouth. Investigational efforts are under way to identify and ameliorate these IMRT-associated toxicities, potentially adding to the complexity of planning these treatments.
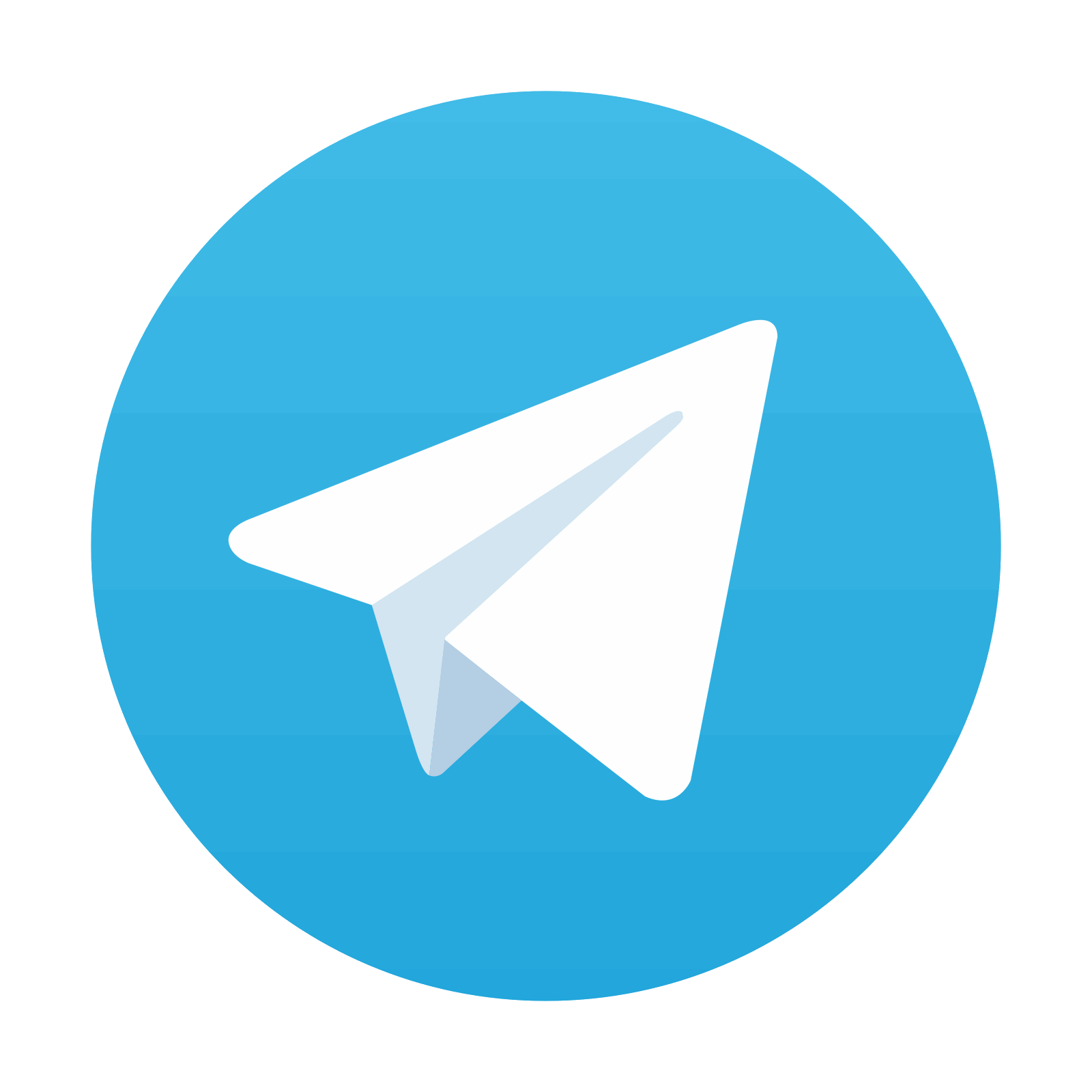
Stay updated, free articles. Join our Telegram channel

Full access? Get Clinical Tree
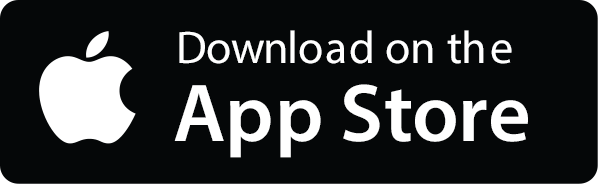
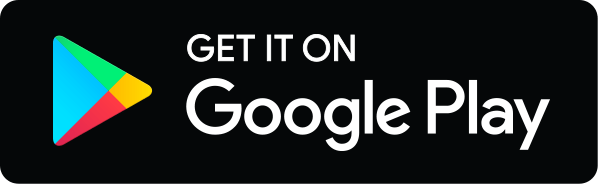