Clinical trial data show that radiation enhances local tumor control of extremity sarcomas with acceptable morbidity when sophisticated radiation techniques are combined with limb-sparing resections performed by oncologic surgeons with sarcoma expertise. Similar controlled data is not available for retroperitoneal sarcomas but some studies suggest a benefit for radiotherapy. Radiation can be delivered by external beam or brachytherapy; it can be given pre-operatively, post-operatively, or intra-operatively. Indications for and advances in radiation therapy are discussed in this article.
Overview
The major therapeutic goals in the treatment of soft tissue sarcomas (STSs) are survival and local tumor control (LC) with the lowest achievable morbidity. For extremity lesions, another important goal is optimal limb function. Surgical resection of the primary tumor is an essential component of treatment for virtually all patients (although not discussed in detail in this article, radiation therapy [RT] alone can be an effective treatment for selected patients who are medically inoperable or who decline surgery). A high-quality surgical margin is necessary for LC when surgery is used without radiation (ie, the surgical plane of dissection should traverse normal tissue outside the reactive tumor zone). This is because STSs tend to infiltrate normal tissue adjacent to the evident lesion. Thus, removal of the gross lesion by a simple excision alone (only a narrow margin) is followed by high rates of local recurrence (LR). Radical resections are associated with a reduction in the LR rate but, in cases of extremity STS, may compromise limb function. For retroperitoneal tumors, they may still fail to achieve LC because of the many adjacent critical structures that cannot be excised. The combination of conservative surgery and RT has been shown in randomized controlled studies to achieve higher rates of LC than surgery alone. Selected patients, generally those with superficial T1 (≤5 cm) lesions that can be widely excised with acceptable margins, can be well managed by surgery alone without RT. Good limb function is achieved in the majority of patients with extremity sarcomas; acute and late RT-associated morbidity are seen in some patients undergoing RT. One focus of current STS clinical trials is the evaluation of techniques and technologies to potentially reduce the risk of radiation-associated morbidity; details of Radiation Therapy Oncology Group clinical studies looking at these questions can be accessed at the following website ( http://www.rtog.org/ClinicalTrials/ProtocolTable/StudyDetails.aspx?study=0630 ), data from these studies are emerging and are discussed later. There are no published randomized studies of which the author is aware that compare surgery alone with surgery and adjuvant RT for retroperitoneal STS. Nevertheless there are data to suggest that radiation can enhance LC for the retroperitoneal tumors with acceptable morbidity when sophisticated radiation techniques are combined with radical resections performed by experienced oncologic surgeons with expertise in managing sarcomas. Advances in RT in this area are discussed.
Because both surgical and radiation techniques are critical for optimizing LC of tumor and functional outcome with acceptable morbidity, it is important to manage these patients in dedicated multispecialty centers composed of physicians with expertise in STS, including orthopedic oncologists, surgical oncologists, plastic surgeons, radiation oncologists, medical oncologists, sarcoma pathologists, and bone and soft tissue diagnostic radiologists. RT may involve external beam RT, which can be 3-D conformal or intensity-modulated RT (IMRT), and use photons or protons, brachytherapy (BRT), intraoperative RT, or combinations thereof. External beam RT can be given either preoperatively or postoperatively. The clinical considerations and the outcome data that must be considered in choosing the most appropriate treatment technique for individual patients are discussed. Although there are similar general considerations for extremity, truncal, head and neck, and retroperitoneal sarcomas, there are sufficient differences in behavior, as well as in surgical and radiation techniques and their underlying rationale, that this discussion is divided into separate sections on extremity/truncal and retroperitoneal sarcomas. In the interest of time and space, the role of adjuvant RT in the management of head/neck and gynecologic or visceral sarcomas and the use of RT in the management of pediatric rhabdomyosarcoma are not discussed. Although the clinical behavior of the pediatric nonrhabdomyosarcoma STSs seems similar to that of the adult STSs, management considerations related to growth retardation and other potential toxicities of RT that are particularly relevant to pediatric patients are sufficiently different that the use of adjuvant RT for these tumors also are not addressed.
Extremity and truncal STS
Introduction
Because STSs tend to infiltrate normal tissue adjacent to the tumor, simple excision alone is followed by LR in 60% to 90% of patients. Radical resection of a wider margin of apparently normal tissue around the tumor reduces the LR rate to approximately 25% to 30%. With the use of compartmental resections, the LR rate drops to 10% to 20% with surgery alone. One study that reported a zero LR rate derives from the amputation arm of the National Cancer Institute trial comparing amputation with limb salvage treatment. Careful selection of patients and attention to technique in some recent series, however, has achieved high rates of LC in selected patients (generally with smaller, lower-grade lesions) with conservative surgery alone.
For the majority of patients with extremity STS undergoing conservative surgery, RT is also used to achieve a high rate of LC with good functional result. The rationale for combining RT with surgery is to avoid the functional and cosmetic deformity associated with radical resection and to avoid the late consequences of high RT doses to large volumes of normal tissue in patients treated with primary RT alone. RT at moderate-dose levels (50–65 Gy) is as effective as radical resection in eradicating the microscopic extensions beyond the gross lesion, resulting in similar high rates of LC. This has allowed maximization of functional and cancer-related outcome without the significant morbidity of radical surgery. Most centers report LC rates of approximately 90% with the combination of margin-negative limb-sparing surgery and RT for high-grade extremity STSs and 90% to 100% for low-grade STSs depending on the size.
In addition to its benefit in improving LC rates, adjunctive RT has had a significant impact on limb salvage for extremity STSs. As an example, in the 1970s, 50% of patients with extremity STS underwent amputation; those patients treated by wide excision alone with limb preservation experienced a 30% rate of LR. With the subsequent application of RT and advanced reconstructive techniques, the rate of primary amputation at major centers has been reduced to approximately 5%, and the incidence of LR with limb preservation has been reduced to 10% to 15% without any measurable decrease in overall survival (OS). A single, prospective randomized trial showed similar rates of disease-free survival and OS for patients treated with amputation or the combination of limb-sparing surgery and RT for extremity STS.
Selection of Patients for Surgical Resection Alone (No RT)
Because of potential acute and late morbidity from RT, it is important to select patients who may be effectively treated with surgery alone. Several published series have evaluated wide-excision limb-sparing surgery alone. In one report, 119 selected patients with extremity STS were grouped according to anatomic location as subcutaneous (n = 40), intramuscular (n = 30), or extramuscular (n = 49). The 70 patients with subcutaneous and intramuscular tumors were all treated by local surgery, and a wide margin (requiring a cuff of fat tissue around the tumor and inclusion of the deep fascia beneath the tumor) was obtained in 56. These patients were followed without postoperative RT. During a median follow-up of 5 years (range, 3.5–10 years), only 4 had an LR, despite 84% having had high-grade tumors. The investigators concluded that postoperative RT may not be necessary in this subgroup. A similar study at the MD Anderson Cancer Center evaluated LC for T1 tumors after excision alone with negative margins. Wide local excision was performed with the intent of including a 1-cm to 2-cm margin of normal tissue around the mass. Negative surgical margins were achieved in 84% of the 88 enrolled patients; the remaining 16% with microscopically positive margins received postoperative RT. In those with excision alone, the 5-year LR rate was 7.9%, and the 5-year sarcoma-specific death rate was 3.2%. In another study, 74 patients with localized STS of the extremity or trunk underwent function-sparing surgery without RT. The overall 10-year actuarial LC rate was 93% and was dependent on the adequacy of surgical margins (87% for patients with margins of less than 1 cm versus 100% for patients with margins of 1 cm or more). The 10-year survival rate was 73%. This approach may be appropriate for carefully selected patients with small (<5 cm) superficial tumors or small deep tumors that can be resected with all margins greater than or equal to 1 cm (or perhaps less if an intervening fascial barrier).
Surgical Resection Combined with RT
The recommended treatment for most patients who are medically and technically operable is the combination of function-preserving surgery and RT, with the exception of the minority of patients with small, generally superficial lesions that can be widely excised with negative margins and good functional results. In most instances, the probability of LC and the late functional and cosmetic results are superior using this combined modality approach. RT is an effective treatment of STS because the radiation sensitivity of cell lines derived from sarcomas is not less than that of epithelial cell lines. For small sarcomas, good LC rates can be achieved by RT alone. LC probabilities of more than 90% for tumors of estimated volumes of 15 mL to 65 mL (approximately a sphere of 3–5 cm in diameter), however, require high radiation doses (>75 Gy). For unresected sarcomas, there seems to be an advantage for doses above 63 Gy. Because most treatment volumes are large, the late normal tissue changes resulting from these dose levels are clinically important in nearly all patients. In animal models, a significantly lower radiation dose is required to achieve LC when RT is combined with simple excision than with RT alone.
The impact of combined modality treatment that includes external beam RT on both LC and survival has been evaluated in only one prospective randomized trial. In this study, 91 patients with high-grade lesions were randomly assigned to surgery plus postoperative chemotherapy with or without postoperative adjuvant external beam RT, and 50 with low-grade lesions were randomized to surgery plus adjuvant external beam RT or surgery alone. In the patients with high-grade lesions, there were no LRs in the patients randomized to external beam RT, whereas the patients receiving only adjuvant chemotherapy had a 22% actuarial LR rate at 10 years. In patients with low-grade sarcoma, the LR rates were 4% versus 33% in the postoperative RT and surgery alone groups, respectively. There was no influence of postoperative RT on OS for either high-grade or low-grade tumors.
Preoperative (Neoadjuvant) Versus Postoperative (Adjuvant) RT
There are potential advantages to both preoperative and postoperative administration of RT. Preoperative RT might reduce tumor burden before resection, theoretically allowing more conservative surgical therapy. RT fields can be limited to the tumor and adjacent tissues at risk for microscopic infiltration, a volume that is considerably smaller than that which must be treated after surgery, where the entire surgical bed is included in the initial target volume irradiated to 50 Gy. RT doses are lower (50 Gy preoperative vs 60–66 Gy postoperative). Postoperative RT allows histologic examination of a tumor specimen, especially the margins, aiding in further treatment planning; it is also associated with fewer acute wound complications.
There is one randomized controlled study comparing preoperative with postoperative RT. This study was designed to evaluate the incidence of acute wound healing complications in patients with potentially curable extremity STS. In this Canadian trial, 190 patients were randomly assigned to either preoperatively RT (50 Gy preoperatively for all 94 patients randomized to this arm with 16–20 Gy postoperative boost reserved for the 14 patients in this arm with a positive margin) or postoperative RT (50 Gy initial field + 16–20 Gy boost field for all patients). Complications were defined as secondary wound surgery, hospital admission for wound care, or the need for deep packing or prolonged wound dressings within 120 days of tumor resection.
The study was terminated when a highly significant result was obtained at the time of a planned interim analysis. With a median follow-up of 3.3 years, a significantly higher percentage of preoperatively treated patients had acute wound complications (35% vs 17%). Other factors associated with wound complications were the volume of resected tissue and lower limb location of the tumor. Late morbidity was initially not reported. Because the RT fields for the postoperative RT were larger and the dose delivered for most patients was higher, the investigators indicated that more follow-up would be needed to assess whether these larger RT volumes and higher RT doses would lead to more late treatment effects in these patients.
In a later publication, the LR rate, regional or distant failure rate, progression-free survival, and functional outcome did not differ between the groups. These data have been updated with a median follow-up of 6.9 years. There remain no differences in LC between the patients in the 2 arms of the study with more than 90% LC. The regional and distant failure rates as well as the progression-free and OS rates are also no different between the 2 arms of the study. The postoperative RT patients, however, have greater 5-year actuarial rates of grade 2 to grade 4 late toxicity (86%) when compared with the preoperative patients (68%) ( P = .0002). Subcutaneous toxicity rated as grade 3 (severe induration and loss of subcutaneous tissue or field contracture greater than 10% linear measurement) or grade 4 (necrosis) was significantly more common in the postoperative group, 36% versus 23% ( P = .02).
There is, thus, a difference in the morbidity profile between preoperative RT and postoperative RT. A higher rate of generally reversible acute wound healing complications occurred in patients receiving preoperative treatment, which was offset by a higher rate of generally irreversible late complications, including grades 3 and 4 fibrosis, in patients receiving postoperative RT. Because few acute wound healing complications occurred in either group when the tumor was in the upper extremity, it seems prudent to treat these patients with preoperative RT. The author has also favored preoperative RT for the majority of patients with lower extremity tumors, because acute wound complications can usually be managed and go on to heal whereas the late treatment effects are usually permanent. For patients with lower extremity lesions, this study makes it clear that new strategies are needed to (1) reduce the risk of acute wound healing problems when patients receive preoperative RT and (2) reduce the risk of late treatment–induced effects when higher-dose, larger-field postoperative RT is given.
Brachytherapy
Compared with external beam RT, BRT minimizes the radiation dose to surrounding normal tissues, maximizes the RT dose delivered to the tumor, and shortens treatment times. In the usual dosage schedule, treatment is completed within 5 days and requires only one hospitalization. At the time of surgery, afterloading catheters are placed in a target area of the tumor operative bed, defined by the surgeon, and spaced at 1-cm intervals to cover the entire area of risk ( Fig. 1 ). BRT can also be used for delivery of a boost to the tumor bed in conjunction with external beam RT.

A phase III trial of postoperative BRT versus no BRT was conducted in 126 patients who had complete resection of either extremity STS or superficial trunk STS. The BRT dose was 45 Gy using low dose rate iridium Ir 192. The 5-year LC rates were 82% and 67% for the BRT and surgery alone groups, respectively. The advantage of BRT was seen only in the high-grade sarcomas and was limited to LC, because there was no difference between the groups in distant metastasis or disease-specific survival.
Although it is unclear whether BRT is associated with a higher risk of wound complications (discussed later), the rate of wound reoperation may be higher. BRT has been combined with free flap construction as a means of enhancing primary healing in difficult anatomic situations without an increase in the incidence of wound breakdown. There have been no randomized comparisons of the relative efficacy or morbidity of external beam RT with BRT.
The Memorial Sloan-Kettering Cancer Center group recently did a retrospective comparison of patients who had received adjuvant RT with IMRT (discussed later) to a group of patients who had received adjuvant brachytherapy. A higher rate of LC was noted in the IMRT group in spite of higher risk features in that group. On the basis of this analysis, they have adopted IMRT as their standard adjuvant RT technique. They did not compare IMRT with 3-D conformal RT. Because the RT treatment target volumes are similar between IMRT and 3-D conformal RT, a difference in LC with these 2 external beam techniques was not expected, although morbidity may differ.
BRT for sarcomas has traditionally been given by low dose rate. There are emerging data on the use of fractionated high dose rate schedules. High dose rate BRT has been used in conjunction with external beam RT for the tumor bed boost in doses of 15 Gy to 24 Gy, often hyperfractionated at 2.3 Gy to 4 Gy twice a day. Early retrospective evidence suggests that comparable rates of LC can be achieved when combining either low dose rate or high dose rate BT with external beam RT. One report using high dose rate BRT alone in doses of 40 Gy at 2.3 Gy to 3 Gy twice a day, however, reported poor LC of only 20% in contrast to 100% when BRT was combined with external beam RT.
External Beam RT Treatment Planning
The RT treatment technique should be carefully planned so that the tissues irradiated are only those judged at risk. To use the smallest target volumes, the part to be irradiated must be securely and reproducibly immobilized, which generally involves customized immobilization, typically with moldable thermoplastic ( Fig. 2 ). Treatment is planned by performing a CT scan of the immobilized, affected extremity. This is facilitated by the availability of a large bore scanner that allows maximum flexibility in arranging the limb such that the contralateral extremity and the trunk will be out the treatment beams. Treatment planning is enhanced by the ability to fuse the RT planning CT scan with the MRI scan. A radiation oncologist defines the target volumes (on each section of the CT/MRI of the affected region); target definition may be improved by review of the planning studies jointly by the radiation oncologist, surgeon, and diagnostic radiologist. The radiation oncologist also defines nontarget critical structures in the treatment volume, specifies dose constraints for these, and then works with the dosimetrists to design treatment techniques that achieve the closest feasible conformation of treatment to target volumes ( Fig. 3 ). This may require complex field arrangements, treatment angles, gapped fields, wedge filters, tissue compensators, bolus, and/or intensity modulation. In general, the radiation oncologist attempts to:
- •
Avoid inclusion of an entire joint space.
- •
Avoid full-dose RT of adjacent bone to reduce the risk of pathologic fracture.
- •
Use wedges and tissue compensators as needed to account for tissue heterogeneities and minimize dose inhomogeneity.
- •
Incorporate an appropriate planning target volume expansion to account for treatment setup variability and any target motion.
- •
Review the treatment plan at multiple levels along the extremity to assess dose homogeneity to the target and normal tissues.

RT Treatment Volumes and Dose
The extent of normal tissue to be irradiated adjacent to the tumor bed in cases of preoperative RT and adjacent to the surgical bed in cases of postoperative RT is not definitively known. Few patterns of failure studies that relate the extent of the RT field to the site of LR have been reported. Because sarcomas are judged to infiltrate along rather than through tissue planes, longitudinal margins proximally and distally have traditionally been considerably more generous than radial margins. Historically, fields that extended from the muscle origin to insertion or provided generous proximal/distal margins on the tumor were used. In some centers from the 1970s through the mid-1990s, 5-cm to 10-cm proximal and distal block margins were used for large grade 1 and small grade 2 lesions and more generous fields with 10-cm to 15-cm margins encompassed large grade 2 to grade 3 lesions. The advent of improved MRI delineation of tumor extent and subsequent surgical experience showing high rates of LC with surgical margins greater than or equal to 1 cm or including fascial barriers prompted radiation oncologists to use proximal/distal margins of 5 cm or less for small grade 1 lesions and 5-cm to 7-cm proximal/distal margins for larger, higher-grade lesions. Newer (3-D) treatment planning systems seem to allow smaller and more accurate treatment volumes in patients with extremity STS.
There are few studies in the literature looking at the appropriate target volume used when planning RT for extremity STS. One group found a remarkable difference in 5-year LC where the margin was less than 5 cm (30%) or at least 5 cm (93%). These data conflict with the BRT data, where acceptable results are achieved using margins 2-cm longitudinally and 1.5 cm to 2 cm laterally. A study from the Royal Marsden hospital has suggested that, as in other tumor sites, the great majority of LRs occur within the high-dose volume. The use of 5-cm proximal and distal block margins and 2-cm radial block margins (on the tumor for preoperative RT or on the surgical bed for postoperative RT) for the first 50 Gy provided high rates of LC in the randomized National Cancer Institute of Canada trial (discussed previously).
A recent retrospective study lends valuable insight into optimal field size in preoperative RT. Patterns of LR were evaluated in 56 patients who underwent preoperative RT to a median dose of 50 Gy. The field size used for all patients was a clinical target volume (CTV) that included the T1 postgadolinium tumor with a 1-cm to 1.5-cm radial margin and 3.5-cm longitudinal margin, with an additional expansion of 5 mm to 7 mm to define the planning target volume. Boost doses of 10 Gy to 20 Gy were administered to the 12 patients with close or positive margins. The 5-year actuarial LC rate of 88.5% was comparable to other series. Tumor size and surgical margin status were the primary predictors of LR. All 5 LRs occurred in patients with either positive resection margins or resection margins of less than 1 mm. No LRs occurred in those with margins of 1 mm or greater. Although good LC rates were achieved, all LRs occurred within the preoperative CTV. The target volume issue is complicated by the variety of histologic subtypes of STS, including some with a very infiltrative biology, such as myxofibrosarcoma, which may require wider fields than some other more circumscribed tumors.
This study raises questions as to whether the more traditional large-volume primary RT field is necessary and whether the boost is necessary when an adequate surgical margin has been achieved. These questions are particularly relevant because of the advent of techniques, such as IMRT and protons, that can allow selective sparing of normal tissues. It is important to determine the volumes that can safely be spared before these techniques can be optimally implemented. Such studies are currently being conducted by cooperative groups in the United States and Europe. A phase II study by the Radiation Therapy Oncology Group (RTOG), which completed accrual in September 2010, is evaluating the role of image-guided RT (cone beam CT, tomotherapy, and so forth) in delivering preoperative RT to patients with extremity STS using more limited CTV margins (RTOG study 0630). CTVs in this study encompass the T1 postgadolinium enhancing region plus a 3-cm longitudinal margin and 1.5-cm radial margin for intermediate-grade or high-grade tumors that are 8 cm or greater. For all other tumors, a 2-cm longitudinal margin and a 1-cm radial margin are used. The primary outcome of interest is toxicity 2 years after treatment, and secondary outcomes will evaluate LC and other survival measures.
Another study, performed by investigators in Toronto, that needs to be carefully considered in the discussion of RT volumes, correlates MRI and surgical findings in patients undergoing surgery without any neoadjuvant therapy. It provided a histologic assessment of peritumoral edema as demonstrated by increased T2-weighted signal intensity on MRI scans performed preoperatively on 15 patients with high-grade extremity or truncal sarcomas ranging from 3.1 cm to 30.1 cm (mean 13.8 cm). The extent of peritumoral T2-weighted signal intensity changes beyond the tumor ranged from 0 cm to 7.1 cm (mean 2.5 cm); contrast enhancement ranged from 0 cm to 5.3 cm (mean 1.1 cm). Tumor cells were identified histologically in the tissues beyond the gross tumor in 10 of 15 cases. In 6 cases, the tumor cells were located within 1 cm of the tumor margin, and in 4 cases, malignant cells were found at a distance greater than 1 cm and up to 4 cm. The location of the tumor cells did not correlate with tumor size or extent of peritumoral changes on the MRI scans. In 9 of 10 cases, however, the tumor cells were identified histologically in areas with corresponding high T2-weighted signal changes on MRI. With ever-increasing ability of the available RT technology to conform the RT dose to the target, this study has significant implications for RT target design and must be considered in future studies of RT volumes in this disease.
The radial CTV margins should be viewed with respect to the direction of most likely spread and are rationally derived from surgical series with high rates of LC without adjuvant RT with surgical margins greater than or equal to 1 cm or an intervening fascial barrier. Because the gross tumor delineation with RT planning imaging is less precise than that achievable in the operating room, radial CTV margins of 1.5 cm to 2.0 cm with approximately 0.5 cm for planning target volume expansion are added to account for daily setup variation. Where there is intervening bone, interosseous membrane, or major fascial planes and these planes are intact in the imaging studies, the radial CTV margins can be constricted to the surface of these structures. When a fascial plane has been violated, wider margins are appropriate to cover areas of potential contamination by tumor.
For patients receiving preoperative RT, 50 Gy is administered over 5 weeks, followed 3 to 5 weeks later by a conservative resection. For patients with negative surgical margins and no other unfavorable prognostic features, such as tumor cut-through or satellite lesions after prior surgical interventions, 50 Gy of preoperative RT seems sufficient to provide LC in a high proportion of patients. Sadoski and colleagues analyzed 132 consecutive patients with STS of the extremities treated with preoperative RT and resectional surgery and found that (1) the 5-year actuarial LC rates were 97% and 81% for patients with negative margins and positive margins, respectively (this difference was highly significant); (2) there was no difference in LC between the various subcategories of negative margins (negative at <1 mm, 96%; negative at greater than 1 mm, 97%; not measured, 94%; and no tumor in the specimen, 100%); (3) there was no difference in LC for treatment of primary and LR lesions (after previous surgery alone) when the tumors were stratified for margin status; and (4) for the patients with negative margin, LC was not a function of STS size.
For patients with positive margins after preoperative RT, it has generally been recommended to use a shrinking treatment volume technique with delivery of either BRT or a postoperative external beam RT boost dose of 16 Gy to 18 Gy to the tumor bed once the surgical wound has healed. A boost dose to 66 Gy is given postoperatively or intraoperatively for microscopically positive margins and to 75 Gy if there is gross residual disease. In patients with frozen section evidence of close or positive margins, a boost dose can be administered intraoperatively using BRT or electron beam. For BRT, it is appropriate to deliver approximately 16 Gy by low dose rate techniques or 14 Gy to 16 Gy by high dose rate given as 3.5 Gy to 4 Gy twice a day for microscopically positive margins. Gross residual disease needs a dose of approximately 20 Gy to 25 Gy. Although delivery of a postoperative RT boost after resection with positive margins remains the standard of care, its efficacy in achieving LC has been called into question by the results of at least one retrospective study using external beam RT.
For patients undergoing postoperative RT, irradiation usually begins 14 to 20 days after surgery, once the wound is healed. After resection of large tumors, it may be necessary to wait 3 to 4 weeks to allow resorption of the seroma. The initial volume must include all tissues handled during the surgical procedure, including the drain site, often encompassing the surgical bed with an initial CTV of approximately 4 cm proximally and distally and 1.5 to 2 cm radially. The dose to the initial CTV is 50 Gy. Progressively shrinking treatment volumes are then used to encompass the tumor bed and, if needed, areas of positive margins or gross residual disease; the final dose is 60 Gy for volumes with negative margins, 66 to 68 Gy for areas of positive margins or LR disease, and 75 Gy for gross residual sarcoma.
The available information on a dose-response relationship for LC of extremity STS treated with surgery and postoperative RT is somewhat conflicting. Mundt and colleagues reported that LC was dose dependent. Although postoperative patients receiving less than 60 Gy had worse LC than those receiving 60 Gy or more, no difference was seen in LC between patients receiving 60 Gy to 63.9 Gy (74.4%) and those receiving 64 Gy to 66 Gy (87.0%) ( P = .5). Severe late sequelae were more frequent in patients treated with doses of 63 Gy or more than in patients treated with lower doses (23.1% vs 0%).
Fein and colleagues reported that patients receiving less than 62.5 Gy had a 5-year LC of 78% versus 95% in those with a dose greater than 62.5 Gy. In a multivariate analysis of patients undergoing postoperative RT, Zagars and Ballo identified dose as an independent variable for LC. Doses of 64 Gy or more correlated with improved LC. Recognizing that the effectiveness of a particular dose was also related to other factors influencing LC, such as margin status, anatomic site, and LR presentation, they recommended postoperative doses of 60 Gy for patients with negative margins and otherwise favorable prognostic features while suggesting increasing doses for less favorable presentations, up to doses of 68 Gy for positive margins. In contrast, other investigators have not been able to demonstrate a clear dose-response relationship in reviews of their patients undergoing postoperative RT. In practice, most centers give 60 Gy of postoperative RT for patients with negative margins and 66 Gy to 68 Gy for positive margins, using shrinking fields (described previously).
With regard to preoperative RT, Robinson and colleagues failed to demonstrate a variation in LC according to dose, although the response rate to preoperative RT was clearly dose dependent. The accepted dose for preoperative RT is 50 Gy.
For treatment of an extremity STS, a good functional result demands that a portion of the cross-section of the extremity be spared from high-dose RT. Thus, some tissue should not be irradiated to high dose to avoid compromising lymphatic drainage. For large tumors that are treated with wide resection, there may be persistent leg edema, requiring the use of a pressure-type stocking, even though the RT treatment volume is less than circumferential. This may be a problem for patients with large (>10 cm) sarcomas of the medial thigh.
When postoperative RT is combined with adjuvant chemotherapy, RT daily dose has been reduced in some series by 10% from 200 cGy to 180 cGy; RT is not given concurrently with doxorubicin. Instead, 2 to 3 days are allowed between the doxorubicin and RT. Some preoperative protocols have interdigitated chemotherapy and RT (discussed later); total preoperative RT has been reduced (ie, 44 Gy) in this setting.
Intensity-Modulated Photon RT
The purpose of RT is to maximize the dose delivered to the tumor while minimizing the exposure of dose-sensitive critical structures to high dose. This has been achieved traditionally by shaping the spatial distribution of the high RT dose to conform to the target volume (hence, 3-D conformal RT), thereby reducing the dose to the nontarget structures. Although this approach is satisfactory in the treatment of targets that are approximately convex in shape, it is less than optimal for targets that contain complex concavities or that wrap around critical structures. Growing experience suggests that IMRT plans produce dose distributions for patients that are superior to 3-D conformal plans, both in terms of dose conformity around the tumor and dose reduction to the specified critical normal structures, albeit at the cost of irradiating a larger volume of normal tissue with a low to moderate dose ( Fig. 4 ). Dosimetric studies comparing IMRT with conformal RT for STS have been reported. When evaluating sarcomas arising in the extremities, pelvis, trunk, and paranasal sinuses, IMRT plans were more conformal. In the extremities, bone and subcutaneous doses were reduced by up to 20%. A conformal-IMRT comparative planning study has been reported for a large extraskeletal chondrosarcoma of the extremity. Not surprisingly, IMRT produced excellent conformal treatment plans for this complex target volume, with less dose to the bone than the 3-D photon plan. Hong and colleagues performed treatment-planning comparisons of IMRT and 3-D conformal RT for 10 patients with STS of the thigh. They were able to document a reduction in femur dose without compromise in tumor coverage. In addition, IMRT reduced dose inhomogeneity (ie, hot spots) in the surrounding soft tissues and skin.
In a single-institution retrospective study, Alektiar and colleagues recently reported on a series of 41 adult patients with STS of the extremity treated with limb-sparing surgery and IMRT. In their cohort, 51% had positive or close (<1-mm) margins, and 68% had tumors greater than 10 cm. At a median follow-up of 35 months, an encouragingly low rate of complications was observed, with 4.8% developing fractures and 32% developing edema (the majority of whom had grade 1 edema). The 5-year actuarial LC rate was 94%. Both the toxicities and LC rates reported in this study are comparable to those achieved with 3-D conformal RT techniques.
IMRT treatment plans, however, often have localized areas within the high-dose volumes, where dose inhomogeneities can be in the range of 10% to 15% above the prescription dose. Because there can also be dose inhomogeneities in the range of 5% below the target dose, treatment plans may be normalized to the 95% isodose line, meaning that selected areas of the treatment volume are receiving daily fractions and total doses of 15% to 20% above the target dose. Depending on the location of these hot spots, there can be unanticipated acute normal tissue toxicity. Because of the multiple field angles used with IMRT, a greater volume of the extremity receives some RT dose, albeit in the low-dose to moderate-dose range. Although early experience suggests acceptable overall levels of late toxicity, further study and longer follow-up are required to determine whether there are late effects specifically attributable to these focal areas of high dose or the larger volume of normal tissue exposed to these low to moderate doses. The group at Memorial Sloan-Kettering Cancer Center recently adopted IMRT (over brachytherapy) as the preferred approach for adjuvant RT.
Proton Beam RT
The rationale for the use of protons (or other charged particles) rather than photons (ie, radiographs, which have traditionally been used for RT) is the approximately 60% reduction in radiation dose to normal tissue with protons. Protons and other charged particles deposit little energy in tissue until near the end of the proton range, where the residual energy is lost over a short distance, resulting in a steep rise in the absorbed dose, known as the Bragg peak ( Fig. 5 ). The Bragg peak is too narrow for practical clinical applications, so for the irradiation of most tumors, the beam energy is modulated by superimposing several Bragg peaks of descending energies (ranges) and weights to create a region of uniform dose over the depth of the target; these extended regions of uniform dose are called spread-out Bragg peaks (see Fig. 5 ). Although the beam modulation to spread out the Bragg peaks increases the entrance dose, the proton dose distribution is still characterized by a lower-dose region in normal tissue proximal to the tumor, a uniform high-dose region in the tumor, and zero dose beyond the range of the deepest Bragg peak, which is usually just beyond the tumor target.
Although protons have been extensively used for sarcomas of the skull base and spine/paraspinal tissues, there may be opportunities to use protons with significant sparing of normal tissues in some patients with extremity STS ( Fig. 6 ). Large, medial proximal thigh lesions can be effectively treated with sparing of the femur, hip joint, genitalia, and anorectal tissue. Lesions around the shoulder can be treated without irradiating the lung apex or shoulder joint. With the recent completion of proton beam facilities at major sarcoma centers in the United States (Massachusetts General Hospital, MD Anderson Cancer Center, University of Florida, and University of Pennsylvania) and Europe (Paul Scherrer Institute in Switzerland and Institut Curie in France as well as other centers in France, Italy, Sweden, and Germany), it is anticipated that a larger proportion of STS patients will be treated with protons.
Neoadjuvant Doxorubicin-Based Chemotherapy Plus RT
Eilber and colleagues, at the University of California, Los Angeles, popularized preoperative regional chemotherapy and RT followed by limb salvage surgery in patients with high-grade STSs. They were able to achieve a high rate of primary limb salvage, low rate of LR (approximately 9%), and long-term survival in 65% of patients. The current regimen consists of doxorubicin (30 mg per day for 3 days) followed by RT given at 28 Gy in 8 fractions. Several other groups using this regimen have also obtained low rates of LR with varying degrees of toxicity. It is not clear if intra-arterial administration provides added benefit over intravenous doxorubicin. One study comparing these 2 methods of administration found that the intra-arterial route was thought associated with a higher incidence of complications but no improvement in survival or function. Another report evaluated 2 separate protocols using preoperative treatment with intravenous doxorubicin and ifosfamide with or without intra-arterial cisplatin; the histologic response and LR rates after surgery were better with the all-intravenous regimen.
Combination intravenous chemotherapy regimens, such as mesna, doxorubicin, ifosfamide, and dacarbazine (MAID), may provide better antitumor activity than single-agent doxorubicin. Interesting results have been noted with neoadjuvant MAID plus RT. The experience with preoperative MAID chemotherapy interdigitated with 44 Gy RT and followed by surgery, postoperative MAID, and further RT (16 Gy) for those with positive margins was reported in a series of 48 patients with high-grade extremity STS greater than or equal to 8 cm. Despite the low objective response rate to preoperative therapy (partial response in 5 and stable disease in 36), all patients were able to undergo limb-sparing surgery initially, with 7 having positive margins. Median tumor necrosis was 95%, suggesting that conventional imaging in this setting may underestimate the degree of response to therapy. Hospitalization for febrile neutropenia occurred in 25% of patients at some time during treatment. Wound healing complications developed in 14 of 48 MAID patients (29%). One MAID patient developed late fatal myelodysplasia. The 5-year rates of LC (92% vs 86%), freedom from distant metastases (75% vs 44%), disease-free survival (70% vs 42%), and OS (87% vs 58%) all compared favorably with the outcomes of a cohort of historical control patients who were matched for tumor size, grade, patient age, and era of treatment.
There were similar results when this regimen was used in a multicenter United States cooperative group trial, in which 66 patients (64 of whom were analyzed) with primary high-grade STS 8 cm or larger in diameter received a modified MAID regimen plus granulocyte colony-stimulating factor and RT, followed by resection and postoperative chemotherapy. Although preoperative chemotherapy and RT was successfully completed by 79% and 89% of patients, respectively, grade 4 hematological and nonhematological toxicity was experienced by 78% and 19% of patients, respectively. Grade 3 or grade 4 skin toxicity was noted in 34%. The limb preservation rate was 92%. With a median follow-up of 2.75 years, the estimated 3-year OS, disease-free survival, and locoregional control rates were 75%, 56%, and 82%, respectively. Three patients (5%) experienced fatal grade 5 toxicities, among whom 2 developed late myelodysplasia and 1 developed infection. It remains to be confirmed in randomized studies whether these aggressive interdigitated approaches offer benefit to the subgroup of patients with large, high-grade sarcomas, who are at the highest risk of treatment failure.
Combining Adjuvant RT with Adjuvant Chemotherapy
Adjuvant chemotherapy is standard treatment of rhabdomyosarcoma, osteosarcoma, and Ewing sarcoma but is not definitively established in other adult STSs. This topic is discussed in the article by Ravi and Patel elsewhere in this issue. The patients most commonly receiving adjuvant chemotherapy, those with deep, intermediate or high-grade STS greater than 5 cm, are generally patients who are likely to also require adjuvant RT. For these patients, chemotherapy has been sequenced with RT in a variety of schedules without any current standard approach. For nearly all of these, concurrent administration of standard doses and schedules of adriamycin, gemcitabine, actinomycin D, and other potent radiosensitizers with standard RT fraction schedules has generally not been done to avoid excessive acute and potentially late normal tissue toxicity. Some of these agents, however, have been combined with RT using altered schedules or doses (discussed previously), such as that popularized by Eilber and colleagues, the interdigitated MAID chemoradiation studies, and those in other experimental studies using altered chemotherapy and/or RT dose delivery schemes.
Soft Tissue Sarcomas of the Hand and Foot
The 5-year survival rate for STS of the hand and foot is approximately 80%, better than that for other extremity sites. This is likely related to the smaller size of these lesions at presentation. With surgical excision and the use of adjunctive RT when the minimum surgical margin is narrow (<2 mm), limb amputation can be avoided as primary therapy in most patients and up to two-thirds of patients can retain a normal or fairly normal extremity.
Wound Healing After Surgery and RT
In general, the use of adjunctive RT is associated with a higher frequency of wound complications. Precise quantification of the impact of RT on wound healing is difficult, however, because of the significant complications seen with surgery alone. In addition, there is much heterogeneity among patients with STS with respect to anatomic site, histologic type, lesion size, prior surgery, medical comorbidities, and age. The use of adjunctive RT can also be associated with joint stiffness, edema, and decreased range of motion. In one trial, extremity RT resulted in significantly worse limb strength, edema, and range of motion than with surgery alone for extremity STS, but the symptoms were transient and did not affect global quality of life.
Preoperative RT and Wound Complications
Preoperative RT is associated with a higher incidence of acute wound complications. In the randomized study of preoperative versus postoperative RT, a significantly higher percentage of preoperatively treated patients had acute wound complications (35% vs 17%). In another series of 202 patients undergoing preoperative RT, the overall wound complication rate was 37%. One patient died with necrotizing fasciitis and 33 (17%) required secondary surgery, including 6 (3%) who required amputation. In a recent retrospective study of 173 patients, major wound complications were more likely to occur in the lower extremity than in the upper extremity. In this cohort, 59% of whom also received preoperative chemotherapy, the rate of major wound complications was 32% after preoperative RT. In another report, wound morbidity was 25% (4 of 16) in patients treated with preoperative external beam RT plus BRT at the time of surgery but only 5% (2 of 40) in those treated postoperatively with BRT followed by external beam RT.
Brachytherapy and Wound Complications
The use of perioperative BRT may increase the incidence of wound complications. In one study of 105 patients with extremity and truncal sarcomas, major wound complications occurred in 9 of 41 (22%) patients treated with BRT compared with 2 of 64 (3%) non–BRT-treated patients. Patients treated with BRT also had a higher total number of complications as well as a higher combined frequency of major and moderate wound complications (44% vs 14%), and a longer time to wound healing (189 days vs 49 days).
The findings were different in a randomized trial of adjuvant BRT versus no BRT, however, in 164 patients with resected extremity or truncal STS. The incidence of serious wound complications was not significantly increased in the group receiving BRT (24% vs 14%, P = .133), but the incidence of wound reoperation was increased (6% vs 0%).
Strategies to Reduce Wound Morbidity
Based on the available literature, the following strategies are suggested to reduce acute wound morbidity in patients being treated with preoperative RT or perioperative BRT :
- •
Gentle handling of tissue during surgery
- •
Meticulous attention to achieving hemostasis before wound closure
- •
Avoidance of closure under tension
- •
Elimination of all wound dead space, using a rotated flap to fill the space, if necessary
- •
Wound drainage with tubes remaining in place until drainage has decreased to satisfactory levels
- •
Use of compression dressings
- •
Immobilization of the affected part for approximately 7 days
- •
Delineation of a subgroup of patients in whom postoperative boost dose can be omitted, which includes patients with negative margins and no tumor cut-through, complete tumor necrosis, or absence of tumor in the resection specimen and perhaps some patients with low-grade tumors and planned, focally positive margins.
Functional Outcome
There are increasing data available on the functional outcome of patients undergoing limb salvage procedures. The majority of patients have good or excellent functional outcome. In one series of 88 patients treated with surgery and either preoperative or postoperative RT, 68 had acceptable functional results, and 61 returned to work. Large tumors, neural sacrifice, proximal thigh tumors, and postoperative complications were associated with poor outcome. Subcutaneous tumors have a more favorable functional outcome. In a single institution series of 145 patients who underwent limb-sparing surgery plus RT, long-term treatment complications included bone fracture in 6%, contracture in 20%, significant edema in 19%, moderate to severe decrease in muscle strength in 20%, requirement for a cane or crutch in 7%, and tissue induration in 57%. Of these patients, 3 (2%) required amputations for treatment-related complications. The percentage of patients ambulating without assistive devices and with mild or no pain was 84%. Higher doses of RT, a long RT portal, and irradiation of more than 75% of the extremity diameter were associated with increased morbidity. Another study examined issues related to quality of life in patients with STS of the lower limb. Although RT was associated with reduced muscle power and range of motion compared with surgery alone, most patients retained good to excellent limb function and quality of life.
The functional outcome is often not as good in patients requiring amputation. In a matched case-control study of patients with lower extremity STS undergoing amputation (n = 12) or a limb-sparing approach (n = 24), there was a trend toward increased disability and handicap for those in the amputation group. Of the 12 amputees, 7 reported ongoing problems with the soft tissue overlying the stump. A Swedish study showed similar results with amputees having a significantly lower Musculoskeletal Tumor Society (MSTS) scoring system score than those with limb-sparing surgery ( P <.001), but there was no difference for the Toronto Extremity Salvage Score (TESS). Tumor localization above knee level resulted in significantly lower MSTS scores and TESS ( P = .003 and P = .02, respectively). There were no significant differences in quality of life between amputees and those with limb-sparing surgery except in physical functioning; 11% of all patients did not work or study. In multivariate analysis, amputation, tumor location above the knee and having muscular pain were associated with low physical function. In the few studies that have assessed quality-of-life issues in STS patients treated with amputation and chemotherapy compared with patients who underwent limb salvage with RT and chemotherapy, contrary to expectations, there were no significant differences in measures of psychological outcome. Thus, a psychological advantage of limb-salvage surgery over amputation has yet to be demonstrated.
Treatment of Local Recurrence
Approximately 10% to 15% of patients with extremity STS who are treated with complete resection and adjuvant RT develop a local tumor failure, the majority within the first 2 years. The approach to patients with an isolated LR is similar to that for those with primary disease, with some modifications. As with primary treatment, the goal is to achieve tumor control, and, if possible, limb salvage with conservative resection. Approximately 10% to 25% of patients with LR disease, however, require amputation. Surgery is an important component of successful salvage therapy. For patients whose primary treatment was surgery alone, re-excision combined with adjuvant RT is the treatment of choice. If RT was used as part of the primary treatment, further RT may not be possible because the maximal tolerance for adjacent normal tissues would have to be exceeded, resulting in a significant risk for problems with wound healing or radiation fibrosis. Additional RT given by BRT (mean dose 47.2 Gy) has been used, however, in some of these patients, with 52% LC and a 33% disease-free survival rates in one series of 26 patients.
Optimal treatment of LR may require both surgery and RT. This was illustrated in one report of salvage therapy using surgery alone or surgery plus reirradiation for 25 patients with LR extremity STS. In the 18 patients undergoing surgery alone, 11 were treated by a conservative procedure and 7 required amputation. Of these 18 patients, 7 relapsed. Of the 10 patients treated with surgery plus RT, none experienced relapse with a median follow-up of 24 months. Six patients (60%) experienced significant wound healing complications, but 3 recovered completely.
Treatment of Locally Advanced Soft Tissue Sarcoma
In patients with advanced STS in whom the tumor has progressed to a point that the functional morbidity of an attempted limb salvage resection is too great for the patient, nonamputative treatment options depend on the site of tumor involvement. For patients with this type of disease limited to the extremity, isolated limb perfusion protocols have been applied with some success. Selected patients can also have their tumors controlled with RT with or without chemotherapy, especially when the tumors are small. For patients treated with a dose of 6400 cGy or greater, Tepper and Suit reported control of unresected STSs in 87.5% of cases of tumors that less than 5 cm in diameter. Treatment was less effective for larger tumors, with LC falling to 53% for lesions 5 cm to 10 cm diameter (53%) and 30% for those greater than 10 cm. Kepka and colleagues recently updated and expanded this experience, reporting on the efficacy of RT on 112 patients with unresected STS. For patients receiving 63 Gy or more, LC at 5 years was 72.4% in patients with lesions 5 cm or less, 42.4% for lesions 5 cm to 10 cm, and 25.4% for lesions greater than 10 cm.
Several centers have reported LC rates of approximately 50% with fast neutron irradiation of inoperable STSs. In addition, several RT sensitizers have been used to treat patients with extensive STS with promising early preliminary results. Trabectedin (ET-743), a novel antitumor agent, has also shown promise in advanced STS, both as a single agent and in combination with other agents.
Summary: Extremity and Truncal Soft Tissue Sarcomas
Treatment of extremity STS requires individual tailoring of the approach because of the wide variety of clinical situations that can arise from tumors that involve various anatomic sites with a range of histologies of differing grades and sizes. Nevertheless, the following suggestions can serve as useful guides. Surgery is always indicated, but the use of adjuvant therapy can vary according to the anatomic site, size, and histologic grade.
- •
In general, patients with superficial, low-grade tumors that are less than 5 cm in diameter can be treated with surgical excision alone when negative margins of greater than 1 cm or an investing fascial barrier or other high-quality tissue plane, such as periosteum, is resected with the tumor. Such patients can expect excellent LC and survival rates approximating 90%.
- •
In patients with intermediate-grade lesions, surgical excision with negative margins in combination with RT has achieved excellent LC with OS rates approximating 80%.
- •
For larger, deep-seated tumors, preoperative RT or postoperative RT seem to have similar efficacy in preventing LR, although postoperative RT generally treats a larger target volume and requires higher doses to the tumor bed. Acute wound healing complications are higher with preoperative RT for lower extremity lesions, but generally irreversible late complications, including grades 3 and 4 fibrosis, are more common in those patients receiving postoperative RT.
- •
In patients with high-grade STS greater than 5 cm, excellent LC can be achieved with surgery and RT, but at least 50% of these patients develop metastatic disease. In this setting, the use of neoadjuvant chemotherapy may benefit some and should be considered in the context of a clinical trial, to be followed by definitive surgery combined with either preoperative or postoperative RT or BRT.
- •
BRT can provide excellent LC and functional results in appropriately selected patients.
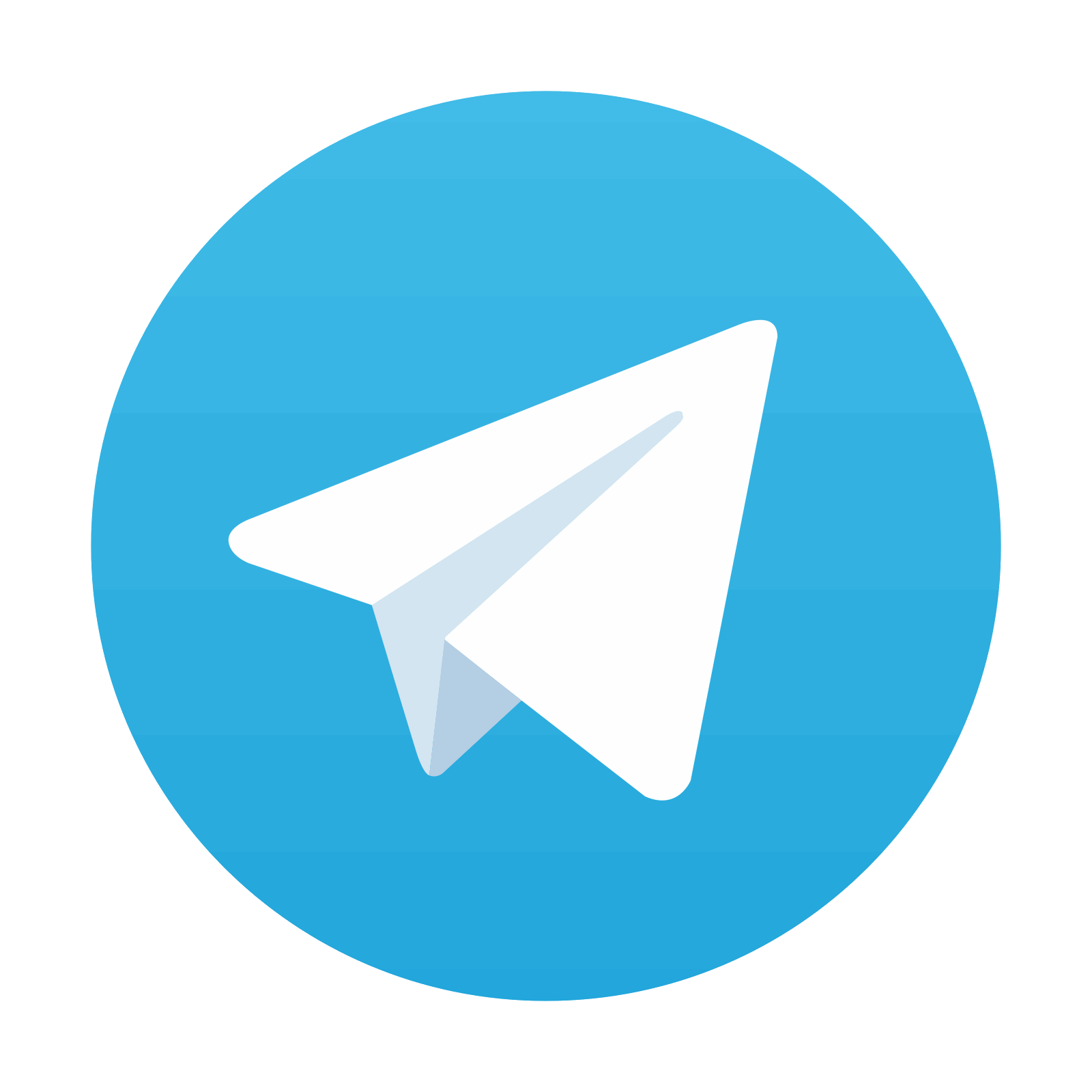
Stay updated, free articles. Join our Telegram channel

Full access? Get Clinical Tree
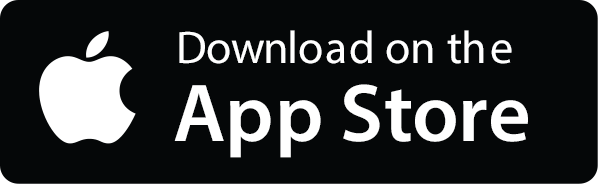
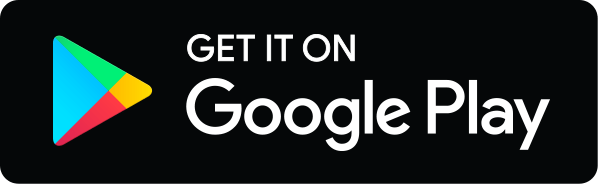