Authors
Patients
Tumor diameter/volume (tumor number)
Dose/fraction size
Objective response rate (%)
Overall survival
Toxicity ≥G3 (%)
Ben-Josef et al. [11]
128 /Liver Malignancies
–
Median 60.75 Gy /1.5 Gy BID (40–90 Gy)
52
Median 15.8 months; 3 year 17%
35
Mornex et al. [15]
27 (16 CP-A/11 CP-B) /HCC only
Single tumor ≤5cm (22); 2 tumors ≤3 cm (5)
66 Gy /2 Gy
92
41% at 29 months
41
Liu et al. [103]
44 (32 CP-A/12 CP-B) /HCC only
<5 cm (16); 5–10 cm (16); >10 cm (12)
Median 50.4 Gy (39.6–60) Gy /1.8 Gy
61.4
Median 15.2 month; 1 year 61%; 2 year 40%
0
Liang et al. [17]
128(108 CP-A/20 CP-B) /HCC only
<125 mL (29), 125–1000 (87), >1000 (12)
Median 53.6 Gy (40 + 60 Gy)/Median 4.88 Gy (3–5 Gy)
55
Median 20 months, 1 year 65%, 2 year 43%
3
Kim et al. [104]
70 /HCC only
<8 cm (37), ≥8 cm (33)
Median 54 Gy (44–54 Gy) /2–3 Gy
54.3
Median 10.8 months, 1 year 43.1%, 2 year 17.6%
12.9
Oh et al. [105]
40 (36 CP-A/4 CP-B) /HCC only
<5 cm (18), ≥5 cm (22)
Median 54 Gy (30–54 Gy) /Median 3 Gy (2.5–5) Gy
62.8
1 year 72%, 2 year 45.6%
0
Seong et al. [16]
398 (294 CP-A/88 CP-B)/HCC only
Median 6.2 cm
Median 45 Gy/Median 1.5 Gy
–
Median 12 months, 2 year 27.9%
–
14.3.2 Stereotactic Body Radiotherapy
The promising results of fractionated conformal radiotherapy renewed interest in further dose-escalation, which was enabled by the development of SBRT, also known as stereotactic ablative radiotherapy (SABR). SBRT allows the delivery of ablative doses of radiation, with rapid dose fall-off at the periphery of the target. Early experience with this highly conformal technique demonstrated excellent local control and toxicity rates as well as acceptable impact on quality of life.
SBRT for the treatment of intrahepatic malignancies was first reported in 1995 by Blomgren et al. [18]. at the Karolinska Institute, Stockholm, where the stereotactic radiosurgery experience for treating brain tumors was adapted to treat extracranial targets. There has since been accumulating clinical experience with SBRT demonstrating sustained response in the majority of treated patients, even for large tumors. Several prospective and retrospective studies have shown excellent 1-year and 2-year local control in the 70–90% range with low toxicity (Table 14.2). A phase I dose-escalation study from Indiana University to determine the maximum tolerated dose of SBRT in selected patients with primary HCC showed that SBRT to doses up to 48 Gy in three fractions is safe in select Child–Pugh class A patients with HCC [19]. They reported their updated experience in 2011 [20] with treating Child–Pugh class A patients to a median of 48 Gy in three fractions and Child–Pugh class B patients to 40 Gy in five fractions, based on the results from their phase I dose-escalation trial. They reported 2-year local control, progression-free survival, and overall survival of 90, 48, and 67%, respectively. Eventually, 23 patients underwent transplant, with a median time to transplant of 7 months. There were no grade 3 or higher non-hematologic toxicities.
Table 14.2
Select SBRT series
Authors | Patients | No. lesions (No. of patients) | Dose/fractions | Median diameter or volume | Local control | Overall survival | Toxicity ≥G3 |
---|---|---|---|---|---|---|---|
Andolino et al. [20] | 60 (36 CP-A/24 CP-B) | 1(51), 2(7), 3(2) | 30–48 Gy/3 – CP-A 40 Gy/5 – CP-B | 3.1 cm | 2 year 90% | 2 year 67% | 35% |
Bibault et al. [106] | 75 (67 CP-A/8 CP-B) | – | 40–45 Gy/3 | 3.7 cm | 1 year 89.8% | 1 year 78.5%, 2 year 50.4% | 6.6% had decompensated cirrhosis |
Bujold et al. [23] | 102 (all CP-A) | 1(40), >1(62) | Median 36 Gy/6 (24–54 Gy) | 7.2 cm | 1 year 87% | Median 17 months | 36% |
Cardenes et al. [19] | 17 (6 CP-A/11 CP-B) | 1(12), 2(2), 3(3) | 36–48 Gy/3 | 4 cm | 100% | 1 year 75%, 2 year 60% | 18% |
Huertas et al. [107] | 77 (66 CP-A/11 CP-B) | 1(67), 2(10) | 45 Gy/3 | 2.4 cm | 1 year 99% | 1 year 81.8%, 2 year 56.6% | 4% RILD, 1 grade 5 toxicity from hematemesis |
Jang et al. [108] | 82 (74 CP-A/8 CP-B) | 1(71), 2(9), 3(2) | 33–60 Gy/3 | 3.0 cm | 2 year 87% | 2 year 63% | 9.8% |
Kang et al. [109] | 47 (41 CP-A/6 CP-B) | 1(39), 2(7), 3(1) | 42–60 Gy/3 | 2.9 cm | 2 year 94.6% | 2 year 68.7% | 10% |
Kwon et al. [110] | 42 (38 CP-A/4 CP-B) | 1(27), >1(15) | 30–39 Gy/3 | 15.4 cc | 1 year 72% | 1 year 93% | 2% |
Sanuki et al. [111] | 185 (158 CP-A/27 CP-B) | 1(185) | 35–40 Gy/5 | 2.7 cm (35 Gy group) 2.4 cm (40 Gy group) | 3 year 91% | 3 year 70% | 13% |
Seo et al. [112] | 38 (34 CP-A/4 CP-B) | – | 30–57 Gy/3 | 40.5 cc | 1 year 78% | 1 year 69% | 0% |
Tse et al. [21] | 31 (all CP-A) | – | Median 30.6 Gy (24–54 Gy)/6 | 173 cc (9–1913 cc) | 1 year 65% | 1 year 85% | 29% |
Wahl et al. [113] | 63 57 CP-A/24 CP-B/2 CP-Ca) | 83 lesions among 63 patients | 27–60 Gy/3–5 | 2.2 cm | 1 year 97.5% | 1 year 74% | 5% |
Yamashita et al. [114] | 79 (67 CP-A/9 CP-B/1 CP-C/2 unknown) | 1(79) | 40–60 Gy/4–10 | 2.7 cm | 18% had local progression | 2 year 53% | 4.6% |
Yoon et al. [115] | 93 (69 CP-A/24 CP-B) | 1(83), 2(10) | 30–60 Gy/3–4 | 2.0 cm | 3 year 92% | 1 year 86% | 6.5% hepatic toxicity |
The Princess Margaret Hospital (PMH) developed a protocol using an individualized six-fraction SBRT dose–allocation approach similar to that from the University of Michigan, in which the prescribed tumor dose was determined based on the effective volume of normal liver irradiated, with encouraging phase I results [21, 22]. In an updated analysis of the completed phase I and II PMH studies, Bujold et al. reported a total of 102 Child–Pugh A HCC patients who were ineligible for local-regional therapies and treated with SBRT to a median dose 36 Gy in six fractions [23] Despite the fact that 55% of patients had portal vein thrombosis, 12% of patients had extrahepatic disease, and 52% of patients had received prior therapies, clinical outcomes were impressive with one-year local control of 87% and median overall survival of 17 months. Also of note, despite the large tumor sizes included in this study (median gross tumor volume of 117 cc and median diameter of the largest lesion of 7.2 cm), tumor size and volume did not correlate with local control or overall survival. Minimum dose to the planning target volume correlated with local control on univariate analysis only (p = 0.02). Grade 3 and higher toxicity was seen in 36% of patients.
14.3.2.1 Comparison with Other Modalities
Given these promising results, SBRT has rapidly become an accepted local therapy for treating patients with HCC. Until recently, there has not been any evidence directly comparing SBRT with other local treatment options for inoperable HCC, such as RFA. Local ablation is considered first-line treatment for localized HCC not suitable for surgery [2]. RFA induces coagulative necrosis of tumor through thermal ablation and is the preferred technique for treating small HCC lesions [24–26]. However, RFA is an invasive procedure, often requiring general anesthesia, and control rates for larger lesions are suboptimal [27, 28]. A recent retrospective study from the University of Michigan demonstrated improved local control for SBRT relative to RFA, particularly for larger lesions. In this series, 224 patients with inoperable HCC were treated with either RFA (161 patients, 249 tumors) or SBRT (63 patients, 83 tumors). SBRT dose used ranged from 27–60 Gy in 3–5 fractions. Freedom from local progression (FFLP) was defined as absence of progressive of disease within or at PTV margin or ablation zone. Repeat RFA was allowed, and tumors requiring multiple RFAs for residual disease were not counted as failure until after all tumors were successfully treated. One and 2-year FFLP for tumors treated for RFA were 83.6 and 80.2%, respectively, while 1- and 2-year FFLP for tumors treated with SBRT were 97.4 and 83.8%, respectively. For tumors that were 2 cm in diameter or larger, RFA was associated with significantly worse local control compared to RFA (hazard ratio for local progression 3.35, p = 0.025). Acute grade 3 and higher complications occurred after 11 and 5% of RFA and SBRT treatments, respectively (p = 0.31). There was no difference in overall survival between patients treated with SBRT or RFA. Based on these results, the authors recommended that SBRT be the preferred treatment for larger lesions.
These same investigators also conducted a similar retrospective study comparing 125 patients receiving SBRT with 85 patients who received TACE [29]. The 1- and 2-year LC with SBRT was 96.5 and 91% compared to 47 and 23% for TACE (p < 0.001). In addition, the 1- and 2-year freedom from hepatic progression for SBRT was 56.5 and 27% compared to 36 and 11% for TACE (p < 0.001).
14.3.2.2 Toxicity
Through precisely targeting tumors and minimizing dose to normal liver, SBRT has not been associated with increased rates of radiation-induced liver disease compared to fractionated, conformal radiotherapy despites its high doses. Patient selection is important, however, as a recent phase I/II study demonstrated increased grade III/IV liver toxicity in Child–Pugh class B patients [30]. In this study, 38 Child–Pugh class A patients and 21 Child–Pugh class B patients were treated with SBRT to 24–48 Gy in three fractions. While only 11% of the Child–Pugh class A patients experienced ≥grade III liver toxicity, 38% of the Child–Pugh class B patients experienced ≥grade III liver toxicity. The investigators found that the volume of liver receiving doses as low as 2.5 Gy to be correlated with developing toxicity in Child–Pugh class B patients. Additionally, there are additional toxicity concerns unique to SBRT given that its safety is predicated on avoiding organs at risk in the delivery of ablative doses of radiation. Gastrointestinal ulceration and perforation as well as hepatobiliary toxicity, including biliary obstruction and stricture, have been reported in patients treated with SBRT for liver lesions [31–34]. Bae et al. found V25 > 20 cc and maximum point dose of 35 and 38 Gy (over three fractions) predicted for severe gastroduodenal toxicity. Similarly, Osmundson et al. found a dose-dependent relationship between hepatobiliary toxicity and the volume of the central hepatobiliary tract treated with SBRT, and recommended dose constraints for the central hepatobiliary tract of V40 (in five fractions) < 21 cm and V37 < 24 cm. Extra caution is warranted when SBRT is combined with new biologic and anti-angiogenic agents. Multiple reports have reported unanticipated toxicities arising after the combination of SBRT and angiogenesis-targeting agents, particularly of late luminal gastrointestinal toxicities [35]. Significant toxicity including grade 3 and grade 4 bowel toxicities and tumor rupture was recently reported in a phase I trial from Princess Margaret Hospital with the use of concurrent sorafenib and SBRT among patients with Child–Pugh Class A HCC [36]. Additionally, half of the patients in the high effective irradiated liver volume group had worsening of Child–Pugh liver function class. Based on these results, the authors recommended against concurrent SBRT with sorafenib outside a clinical trial.
Although the majority of the clinical reports of SBRT have highlighted its excellent local control and toxicity rates, SBRT can also offer potential favorable impact on the dimension of quality of life as well. SBRT is a noninvasive outpatient therapy, requires minimal pre-medication, and involves a limited number of treatments that may be more convenient and appealing for patients.
In 2008, Mendez Romero et al. prospectively assessed the impact of SBRT on the quality of life of 28 patients with liver tumors (19 had liver metastases, 9 had HCC) using the EQ-5D index, EQ-5D VAS and European Organization for Research and Treatment of Cancer Quality of Life Questionnaire Core-30 (QLQ C-30) global health status instruments at time points of directly before and 1, 3, and 6 months after treatment, with high response rates (70–100% across all time points) [37]. They found that quality of life was not significantly influenced by treatment with SBRT, and that patients maintained pretreatment level quality of life in the six months period after treatment.
Klein et al. subsequently published their quality of life outcomes that were collected prospectively over a ten-year period from 222 patients treated with SBRT for liver cancer, about half of whom had HCC [38]. The majority of HCC patients had Child–Pugh A liver function and received 24–60 Gy in six fractions. Quality of life was assessed using the QLQ-C30 and/or Functional Assessment of Cancer Therapy—Hepatobiliary Cancer (FACT-Hep, version 4) questionnaires at baseline and 1, 3, 6, and 12 months after treatment. They found that liver SBRT temporarily worsened appetite and fatigue at one month but that this recovered by three months. Patients with small tumors 6 cm or less in diameter did not experience a clinically significant decline in QOL at any time point. Overall quality of life was unchanged for all patients.
Although these quality of life results compare favorably to other treatment approaches, including resection, RFA and TACE, for HCC, [39–42] no direct comparisons have yet been published. Health-related quality of life in HCC is understudied, but is increasingly recognized as an important endpoint, and should be an important factor in the treatment decision for patients and their physicians.
14.3.3 Particle Therapy
Given the need to limit radiation dose to the liver in patients with HCC due to underlying liver disease and decreased functional reserve, the dosimetric characteristics of heavy particle radiotherapy, such as protons, are appealing. The Bragg peak phenomenon results in decreased integral dose to the uninvolved portion of the liver. Thus, proton therapy is ideal for safe dose-escalation to tumors in located in critical, radiosensitive organs.
Most of the published clinical experiences of proton therapy are retrospective series predominantly from Asia. One of the first clinical series of proton therapy for treating HCC was reported from the University of Tsukuba in Japan [43]. In this series, 162 patients with 192 HCCs lesions were treated from 1985–1998 with proton radiotherapy to a median dose of 72 Cobalt Gray Equivalent (CGE) in 16 fractions (50–88 in 10–24 fractions). The median tumor size was 3.8 cm (ranging from 1.5–14.5 cm), 25 patients (15%) had portal vein tumor thrombus, and 72 patients (44%) had Child–Pugh Class B and C cirrhosis. Overall survival and local control at five years were 23.5 and 86.9%, respectively. Only 3% of the patients experienced grade 2 or higher late toxicity. There was no treatment discontinuation due to liver toxicity and no treatment-related deaths.
Several prospective single-institution proton series have also reported five year local control of 81–88% and five year overall survival of 25–39% [44–47] (Table 14.3). In a phase II trial at Loma Linda, 76 patients (22 patients had Child–Pugh A, 36 patients had Child–Pugh B, 18 patients had Child–Pugh C cirrhosis) were treated to 63 Gy in 15 fractions. Of these patients, 18 eventually underwent a liver transplant; 6 (33%) of these patients had a complete response and 7 (39%) had microscopic residual disease. Median progression-free and overall survival was 36 and 18 months, respectively. No grade 3 or higher toxicity was reported. Because of these promising results, Loma Linda conducted a randomized trial comparing transarterial chemoembolization and proton beam radiotherapy with the primary endpoint of progression-free survival [48]. Interim analysis results showed a trend toward improved 2-year progression-free survival (48 vs. 31%, p = 0.06) with proton beam. There were also significantly fewer hospitalization days after proton treatment compared to TACE (166 vs. 24 days, p < 0.001). The 2-year overall survival for all patients was 59%, and was not significantly different between treatment groups.
Table 14.3
Select prospective studies of charged particle therapy for hepatocellular carcinoma
Authors | Patients | Particle | Dose/fractions | PVT (%) | Local control | Survival | Toxicity ≥G3 (%) |
---|---|---|---|---|---|---|---|
76 (47% CP-B, 24% CP-C) | P | 63 GyE/15 | 5 | 2 year 75% | 18.4 months (median) | 0 | |
Fukumitsu et al. [45] | 51 (20% CP-B, 0% CP-C) | P | 66 GyE/10 | 0 | 3 year 94.5% | 5 year 39% | 2 |
Kawashima et al. [44] | 30 (33% CP-B, 0% CP-C) | P | 76 GyE/20 | 40 | 2 year 96% | 3 year 62% | 40 |
Kato et al. [116] | 24 (33% CP-B, 0% CP-C) | C | 49.5–79. GyE/15 | 58 | 1 year 92%, 5 year 81% | 5 year 25% | 26 |
Hong et al. [52] | 44 (32 CP-A, 9 CP-B, 3 no cirrhosis) | P | 58.0 GyE/15 | 29.5 | 2 year 94.8% | 1 year 76.5% | 2.3 |
Other particles including carbon ions have also been explored in the treatment of HCC. Carbon ion therapy has the additional advantage over proton therapy of a narrower penumbra, increased relative biological effectiveness (RBE), and lower oxygen enhancement ratio (OER). Thus, carbon ion beams are expected to provide a beneficial dose distribution that leads to improved therapeutic ratio, more so than with proton beam. A phase I–II trial from Japan delivered 49.5–79.6 GyE in 15 fractions using carbon ions to 24 patients (16 had Child–Pugh A and 8 had Child–Pugh B cirrhosis), and reported 5-year local control of 81% and 5 year overall survival of 25%. One patient had a grade 3 early skin reaction while five patients experienced grade 3 early hematological toxicity and three patients experienced late grade 3 hematological toxicity. Others have also reported the safety and effectiveness of hypofractionated carbon treatment (52.8 GyE in four fractions) [49]. However, longer follow-up is required to ensure that these large doses per fraction do not result in increased late toxicity.
The excellent conformality of particle therapy is particularly advantageous in situations where there is high risk of developing toxicity with radiation. For example, many patients with portal venous thrombosis have poor functional liver reserve but need large volumes of their liver irradiated. Hata et al. reported treating 12 patients who had tumor thrombus in the main trunk or major branches of the portal vein with a total of 50–72 Gy in 10–22 fractions using protons [50]. They reported 2-year progression-free survival of 67%, and no grade 3 or higher toxicity. Two patients were alive with no evidence of disease at 4.3 and 6.4 years after therapy. Another proton series of 35 patients with HCC portal venous thrombosis were treated with 50–72 CGE with local control rates of over 45% at 2 years. Only three patients developed severe acute toxicity [51]. Recently, a multi-institutional phase II trial of proton therapy for unresectable HCC and intrahepatic cholangiocarcinoma treated tumors to a mean dose of 58 GyE in 15 fractions [52]. With this regimen, the results showed a very promising 2-year local control of 94.8% for HCC despite nearly 30% of patients having vascular thrombus.
The Tsukuba proton radiotherapy group has also reported on the efficacy and safety of HCC retreatment for either progressive or synchronous HCC lesions in a series of 27 patients with 68 total lesions [53]. The median interval was 24 months between the first and second course of treatment and median dose delivered was 72 Gy in 16 fractions and 66 Gy in 16 fractions for the first course and rest of the courses, respectively. The 5-year local control rate and overall survival were 87.8 and 56%, respectively. One patient with Child–Pugh Class B and another patient with Child–Pugh Class C cirrhosis experienced acute hepatic failure.
While the reported clinical experience with proton and carbon ion therapy have been associated with some of the best outcomes so far, particularly for patients who are at high risk of hepatic insufficiency with radiation, particle therapy is an expensive technology available at few facilities worldwide. Additionally, there is increasing evidence demonstrating comparable clinical efficacy and safety with photon SBRT. Further comparative evaluation of particle therapy is needed to determine whether its theoretical advantage translates into a clinically significant advantage over other less expensive and more accessible therapies, and if so, which patient subgroups could benefit most from this treatment modality.
14.3.4 Radioembolization
Trans-arterial radioembolization [TARE, also known as selective internal radiation therapy (SIRT)], involves injection of radioactive microspheres for delivery of internal radiation. The microspheres are loaded with a radioactive compound, such as Yttrium-90 (Y-90), which is a beta-emitter with a short half-life (2.67 days) and limited tissue penetration (average 2.5 mm, maximum 11 mm). Selective catheter-based administration of Y-90 microspheres via the hepatic artery preferentially delivers high-dose radiation to the tumor-associated capillary bed while sparing normal liver tissue, allowing for delivery of doses up to 50–150 Gy [54, 55] without developing the clinical complications of conventional external beam radiotherapy [56]. In contrast to TACE, the dominant mechanism of occlusion is microvascular rather than macrovascular occlusion, and tumor necrosis is believed to be predominantly a result of radiation rather than ischemia [57]. Two common commercial microspheres available are TheraSphere®, made of glass, and SIR-Spheres, made of resin; although they differ in several characteristics including the activity per microsphere and number of microspheres typically injected in a treatment, clinical outcomes are similar between the two [58]. In the United States, FDA has approved the use of Sir-Spheres for treatment of unresectable colorectal cancer metastases to the liver, and the use of TheraSphere® for treatment of unresectable HCC under a Humanitarian Device Exemption provision.
TARE has traditionally been used in clinical practice for patients with advanced-stage HCC, patients felt to be poor candidates for TACE, early stage patients as a bridge to liver transplantation, or for palliation of non-ablatable tumors. Long-term survival outcomes support the safety and efficacy of TARE for patients with HCC, including those with advanced disease and limited treatment options. Salem et al. analyzed outcomes of 291 patients (526 treatments) treated with Y-90 as part of a single-center prospective longitudinal cohort study, and reported response rates of 42 and 57% based on WHO and EASL criteria, respectively [59]. The overall time to tumor progression (TTP) was 7.9 months, with median survival of 17.2 months for Child–Pugh class A, and 7.7 months for Child–Pugh class B disease. In 108 consecutive patients (159 treatments) with advanced HCC and liver cirrhosis treated with Y-90 microspheres, Hilgard et al. reported a 40% response rate by EASL criteria, time-to-progression of 10.0 months, and median survival of 16.4 months [60]. The most common side effect was a grade 1/2 transient fatigue syndrome. Sangro et al. conducted a European multicenter retrospective analysis on prognostic factors driving survival in patients treated with TARE using Y-90 microspheres [61]. The majority of patients were Child–Pugh class A (82.5%), had underlying cirrhosis (78.5%), but many had multinodular disease (75.9%) and/or portal vein occlusion (13.5% branch, 9.8% main). Median overall survival of all patients was 12.8 months, which varied by Barcelona Clinic Liver Cancer (BCLC) staging—(BCLC A, 24.4 months; BCLC B, 16.9 months; BCLC C, 10.0 months). Tumor burden and liver function were predictive of survival within the context of BCLC staging. Treatment was well tolerated with the majority of adverse events (fatigue, nausea/vomiting, and abdominal pain) being mild.
While two randomized controlled trials established TACE as the standard of care in BCLC intermediate-stage disease [62, 63], demonstrating a survival benefit for TACE compared with conservative management with best supportive care, there has been few published randomized evidence comparing TARE and TACE. A phase II randomized trial comparing TACE and TARE showing improved time to progression with TARE compared to TACE (median >26 vs 6.8 months, p = 0.007), but no difference in overall survival (18.6 vs. 17.7 months, p = 0.99) [64]. Nonrandomized, retrospective analyses have attempted to compare outcomes of patients with HCC treated with TACE or TARE [65–67]. Salem et al. reported less toxicity and longer time to tumor progression (13.3 months vs. 8.4 months) with TARE compared with TACE [66]. El Fouly et al. conducted a prospective non-randomized controlled trial comparing TARE with TACE for patients intermediate-stage HCC, and found no differences in median survival and time-to-progression despite higher tumor burden in the TARE cohort, as well as significantly higher rate of adverse event and hospitalization time in the TACE cohort [68]. The pilot study SIRTACE was the first to compare single session TARE with multi-session TACE for unresectable HCC. Both modalities were well tolerated without any significant differences in health-related quality of life. Local control was similar with best overall response rate for target lesions of 13.3 and 30.8%, and disease control rates of 73.3 and 76.9% for TACE and TARE, respectively [69], suggesting that TARE may be an appropriate alternative option for patients who are eligible for TACE. Three ongoing randomized controlled trials are further evaluating TARE versus TACE for patients with intermediate-stage HCC (NCT00956930, NCT01381211, NCT02004210).
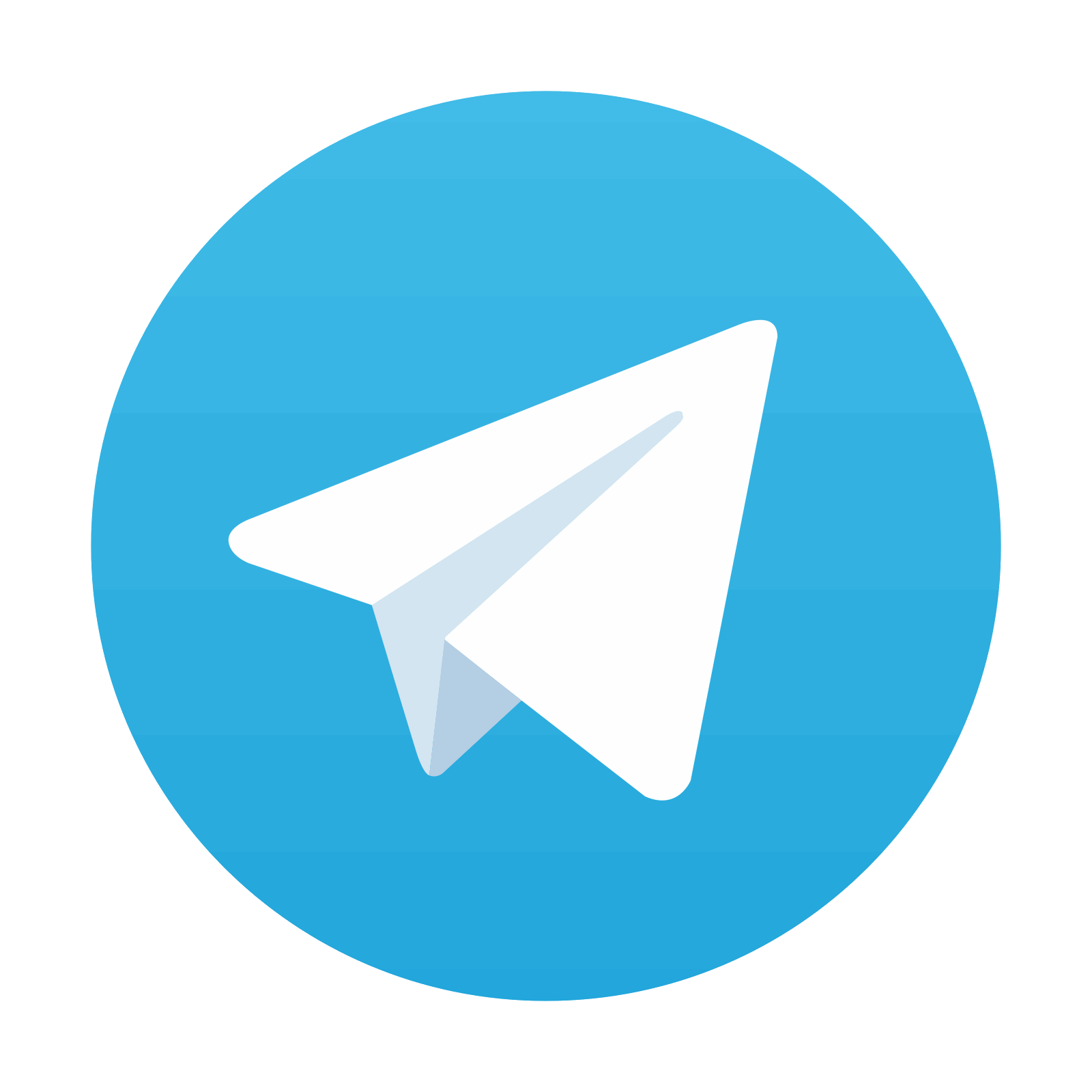
Stay updated, free articles. Join our Telegram channel

Full access? Get Clinical Tree
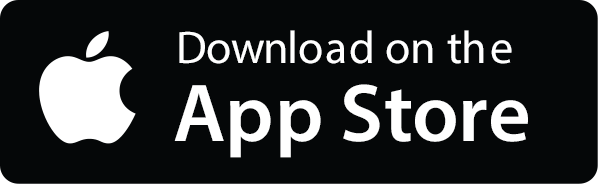
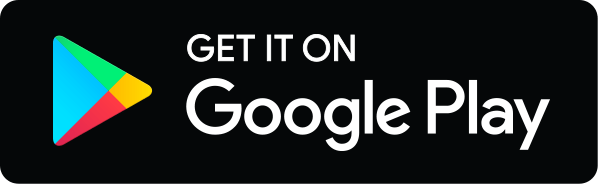