The many advances in radiotherapy for squamous cell cancer of the head and neck described in this article will have significant effects on the ultimate outcomes of patients who receive this treatment. The technological and clinical advances should allow one to maintain or improve disease control, while moderating the toxicity associated with head and neck radiation therapy.
Key points
- •
Changes in the use and implementation of multiple types of imaging can lead to improvements in the targeting of radiation therapy for head and neck cancers.
- •
Therapeutic radiation for head and neck cancers is improving based on the use of image guidance and different techniques for treatment delivery.
- •
The unique biology of human papilloma virus (HPV)-related cancers opens up the possibility of delivering less radiation to less tissue while maintaining efficacy.
Introduction
The field of radiation therapy for squamous cell cancer of the head and neck (SCCHN) has been transformed over the past decade, but the basic elements of radiotherapy remain the same: determining the appropriate dose to deliver, accurately identifying the targets, and delivering treatment with minimal normal tissue toxicity. The current standard of care, intensity modulated radiation therapy (IMRT), delivers improved tumor doses compared with the historical 3-field/3-dimensional conformal approaches, which were both effective in treating SCCHN. Current studies assessing the efficacy of IMRT demonstrate excellent outcomes, while acknowledging a contribution from the changing nature of oropharynx cancers and the use of retrospective data. Much of the benefit derived from IMRT has been in the area of normal tissue sparing. The treating physician exercises substantial control over where the dose is (and is not) directed; thus IMRT has led to significant improvements in obtaining a differential between the doses delivered to the tumor related targets and normal tissue structures. These improvements were first clearly demonstrated in nasopharynx cancer where, because of anatomic considerations, the benefits are potentially the most significant. Results were derived from a combination of phase 2, 3, and retrospective studies. A phase 3 study that included oropharynx and hypopharynx compared IMRT and lateral opposed fields using 3-dimensional conformal methods and demonstrated similar results. For these sites, there is also a significant body of retrospective data highlighting the excellent normal tissue outcomes resulting from IMRT-based improved dose distributions. In order to achieve these distributions, one must precisely determine which structures need to be treated. This determination requires optimal imaging data and also requires a detailed knowledge of the disease behavior. The imaging component will be addressed in this article, while the second item emphasizes the importance of treatment at a high-volume center, which was demonstrated for 3-field treatment ; IMRT potentially increases the benefit expected from the expertise of the individual practitioner. The concept used to describe the balance between tumor control and normal tissue effects is the therapeutic ratio (TR), and improving the TR has been a longstanding goal of radiation therapy. Further improvements in TR have taken on increased importance, as it is known that there are myriad effective treatments for SCCHN occurring in a younger and healthier population.
Overview
Multiple pathways to improving the TR for radiation therapy SCCHN patients will be addressed in this article. Advances in imaging allow the physician to more accurately identify tumor and adjacent tissues that are at risk of containing microscopic disease. The approaches addressed here include improvements of existing technologies (eg, MRI, positron emission tomography [PET]) as well as the use of other modalities. A second approach for improving TR is to improve the methods used for delivering radiation to the patient. The topics included in this paradigm are modifying how standard IMRT—photon based—is given, as well as the use of proton therapy. Underlying the designation of appropriate targets is the understanding of the disease being treated and how that disease may be changing. Specifically, there is the ever-present influence of HPV-related disease and the questions it raises in radiation therapy, such as how much radiation is necessary and how big a volume needs to be treated.
This article focuses on radiation therapy alone and cannot address the many important relationships with surgery and systemic therapy.
Introduction
The field of radiation therapy for squamous cell cancer of the head and neck (SCCHN) has been transformed over the past decade, but the basic elements of radiotherapy remain the same: determining the appropriate dose to deliver, accurately identifying the targets, and delivering treatment with minimal normal tissue toxicity. The current standard of care, intensity modulated radiation therapy (IMRT), delivers improved tumor doses compared with the historical 3-field/3-dimensional conformal approaches, which were both effective in treating SCCHN. Current studies assessing the efficacy of IMRT demonstrate excellent outcomes, while acknowledging a contribution from the changing nature of oropharynx cancers and the use of retrospective data. Much of the benefit derived from IMRT has been in the area of normal tissue sparing. The treating physician exercises substantial control over where the dose is (and is not) directed; thus IMRT has led to significant improvements in obtaining a differential between the doses delivered to the tumor related targets and normal tissue structures. These improvements were first clearly demonstrated in nasopharynx cancer where, because of anatomic considerations, the benefits are potentially the most significant. Results were derived from a combination of phase 2, 3, and retrospective studies. A phase 3 study that included oropharynx and hypopharynx compared IMRT and lateral opposed fields using 3-dimensional conformal methods and demonstrated similar results. For these sites, there is also a significant body of retrospective data highlighting the excellent normal tissue outcomes resulting from IMRT-based improved dose distributions. In order to achieve these distributions, one must precisely determine which structures need to be treated. This determination requires optimal imaging data and also requires a detailed knowledge of the disease behavior. The imaging component will be addressed in this article, while the second item emphasizes the importance of treatment at a high-volume center, which was demonstrated for 3-field treatment ; IMRT potentially increases the benefit expected from the expertise of the individual practitioner. The concept used to describe the balance between tumor control and normal tissue effects is the therapeutic ratio (TR), and improving the TR has been a longstanding goal of radiation therapy. Further improvements in TR have taken on increased importance, as it is known that there are myriad effective treatments for SCCHN occurring in a younger and healthier population.
Overview
Multiple pathways to improving the TR for radiation therapy SCCHN patients will be addressed in this article. Advances in imaging allow the physician to more accurately identify tumor and adjacent tissues that are at risk of containing microscopic disease. The approaches addressed here include improvements of existing technologies (eg, MRI, positron emission tomography [PET]) as well as the use of other modalities. A second approach for improving TR is to improve the methods used for delivering radiation to the patient. The topics included in this paradigm are modifying how standard IMRT—photon based—is given, as well as the use of proton therapy. Underlying the designation of appropriate targets is the understanding of the disease being treated and how that disease may be changing. Specifically, there is the ever-present influence of HPV-related disease and the questions it raises in radiation therapy, such as how much radiation is necessary and how big a volume needs to be treated.
This article focuses on radiation therapy alone and cannot address the many important relationships with surgery and systemic therapy.
Imaging advances
As head and neck radiation treatments have become more targeted, accurate target delineation has become more critical to avoid missing tumor and identifying areas at highest risk for microscopic tumor spread. Additionally, the steep dose gradients and high doses delivered in close proximity to critical organs such as the spinal cord and brainstem necessitate millimeter-level accuracy in the definition of these normal structures. Thus, it is crucial to incorporate all anatomic information available during the radiation treatment-planning process. In many cases, the clinical examination is the cornerstone of this process; however, diagnostic imaging modalities such as computed tomography (CT), PET, and MRI provide complimentary information and have been increasingly incorporated into clinical practice.
Computed Tomography Scans
Diagnostic CT imaging with intravenous contrast is recommended by national guidelines for the majority of SCCHN patients (Network 2015) and can aid in identifying primary lesions and pathologically involved lymph nodes. For subsites in close proximity to bone (eg, the mandible or clivus), CT can help determine the extent of tumor spread. In patients who do not undergo primary surgical treatment, diagnostic CT represents a significant improvement over clinical examination alone to identify pathologically involved lymph nodes. These radiographically positive nodes can then be irradiated to higher doses appropriate for gross disease. Similarly, diagnostic CT is commonly used to follow responses after the completion of radiation of involved lymph nodes to help determine whether a post-treatment neck dissection is warranted.
In addition to diagnostic CT scans, CT is also typically incorporated directly into the modern radiotherapy treatment planning process using CT simulators, which can produce images that approach diagnostic quality. The treating clinician uses these images, reformatted in the axial, coronal, and sagittal planes, to better identify areas either involved by disease or at risk of harboring microscopic disease spread. Ideally, images from multiple planes are used in tandem during the radiation treatment-planning process; over-reliance on axial images, for example, can lead to inadequate superior and inferior treatment margins.
PET, PET–Computed Tomography Imaging
The use of PET/PET-CT scans has increased dramatically over the last few decades, largely as a result of their utilization in oncology. For head and neck cancers, PET can be used alongside or in place of chest CT to evaluate for metastatic disease, with the benefit that PET also identifies metastases to unusual sites such as bone or soft tissue. PET can also serve as a useful adjunct in radiation target delineation. PET performed in addition to CT may have improved sensitivity and specificity for identifying pathologically involved lymph nodes and is also likely more cost-effective. Similarly, PET may help delineate the extent of primary disease; indeed, PET/CT-derived target volumes were the most reproducible in a study that compared various imaging modalities, and also the most accurate. However, caution is warranted, as the spatial resolution of PET scans is larger than the margins typically used in head and neck radiation treatment planning. Additionally, the absolute standard uptake values (SUVs) or other criteria such as adaptive thresholding best suited to identify malignancy has yet to be determined and can lead to large variability in tumor volume.
In order to best utilize the biologic information provided by diagnostic PET scans, PET images can be viewed alongside treatment-planning CT scans, or fused to these CT scans so the 2 scans can be viewed simultaneously. A potential issue when incorporating PET scans into the radiation-planning process is the variability in position that occurs between diagnostic PET and treatment-planning CT scans. Deformable registration is one technique that can help address this limitation by more accurately mapping the diagnostic PET scan using adjustable landmarks. Deformable registration may also be helpful for addressing changes in anatomy that occur following induction chemotherapy or surgery, because current guidelines specify treatment fields should be based on the pretreatment disease extent.
MRI Imaging
Similar to PET, MRI is being increasingly incorporated into radiation treatment planning, using fusion or deformable registration techniques. MRI can be particularly useful for identification of pathologic retropharyngeal lymph nodes or defining the extent of primary disease in critical areas such as the nasopharynx, sinuses, oral tongue, or parotid. MRI can also provide better resolution for targeting tumor-invading cranial nerves or critical structures such as the brainstem, optic nerves, and optic chiasm. Finally, MRI can aid in target delineation when CT scans are limited by dental artifact. In head and neck treatment planning, evidence suggests that MRI may be complimentary with PET and CT for target volume delineation.
Recent advances are expanding the ways various imaging modalities can be incorporated into radiation treatment planning. SUVs or metabolic tumor volumes (MTVs) obtained by PET may be prognostic, with higher initial SUV or larger MTV associated with local recurrence in patients treated with standard radiation with or without chemotherapy. Therefore, next-generation dose-painting approaches could attempt to incorporate this information to escalate radiation dose to biologically active or bulky regions within a tumor and perhaps decrease the radiation dose delivered to those areas of a tumor that are less active. PET is also increasingly used to follow treatment response of SCCHN. For example, patients with PET-negative lymph nodes following radiotherapy may not benefit from a neck dissection, even if they remain visible on CT.
MRI scans also have the potential to change the practice of head and neck oncology. Conventional T1 or T2 weighted MRI sequences provide high-quality imaging of areas of the head and neck that were previously difficult to assess, such as the maxillary and sphenoid sinuses.
Additional MRI sequences have been investigated for their ability to identify tumors and monitor response, with promising early results. For example, diffusion weighted MRI (DWI) can help differentiate involved lymph nodes from benign reactive lymphadenopathy because of the increased cellularity of lymph nodes with tumor infiltration, and have demonstrated superior sensitivity and specificity for this purpose when compared with CT or traditional MRI sequences in small studies. Dynamic contrast-enhanced (DCE)-MRI provides information on vascular perfusion and permeability that could also add complimentary information in the identification of regions in head and neck tumors at risk for relapse because of poor baseline functional vasculature.
Future developments will likely further integrate diagnostic imaging and radiation treatment planning. MRI- and PET-based simulators and simulation techniques are increasingly being developed and refined for radiation oncology practice. These range from careful use of the same immobilization devices for both radiation simulation and diagnostic scan to fully integrated treatment-planning devices that offer the capabilities for multiparametric imaging. PET-based delineation of treatment volumes can also potentially be automated ; however, this technique would remain dependent on the validity of parameters used to define PET positivity.
Novel functional imaging techniques allow physicians and researchers to better identify disease at the onset of treatment and track parameters that may be important predictors of treatment response. Areas of hypoxia in head and neck cancers may promote radioresistance. Therefore, identifying these regions could allow for tailoring of radiation plans and monitoring the effects of radiation on this aspect of tumor response. Fluorine-18-labeled fluoromisonidazole PET (18F-FMISO-PET) is one potential imaging modality capable of identifying hypoxic areas within a tumor. Early studies have incorporated 18F-FMISO-PET based treatment planning for SCCHN to show the feasibility of dose escalation to the hypoxic component of tumor volumes. 18F-FMISO-PET is somewhat limited by relatively slow diffusion and uptake; therefore, other next-generation nitroimidizaole derivatives are also being explored for their ability to identify hypoxic tumor regions. In addition to hypoxia, other potential PET tracers that may eventually be useful for radiation target delineation are being explored for their ability to detect tumor cell proliferation (18FLT), protein synthesis (18FET, 11C-MET), or anabolic pathway metabolism in cancer tissues (11C-ACE).
Advances in radiation delivery
Appropriately identifying clinical targets via physical examination and imaging represents an important element in treating SCCHN. It is also essential to deliver radiation therapy in as accurate and conformal a manner as possible to those targets.
Image Guidance
The sharp dose gradients present in IMRT dose distributions can be significantly altered by small variations in the day–to-day patient position/setup ; thus, it is essential to minimize the variation. This is achieved by several means:
- •
With reliable, reproducible daily positioning
- •
By building in an additional margin of uncertainty for setup variability (the planning target volume, PTV), typically a 3 to 5 mm volumetric expansion of the target volume
- •
Using image guidance
Image guided radiation therapy (IGRT) refers to the use of images acquired during treatment to guide delivery of RT principally by ensuring patient positioning prior to treatment. Major advancements in 2-dimensional and 3-dimensional image guidance occurred when kilovoltage (kV) X-ray units and image detectors were built into newer linear accelerators. Other forms of setup verification include the use the megavoltage (MV) treatment beam itself to construct an image, although these have inferior image quality to diagnostic kV beams. Once the patient is setup, kV images are taken in orthogonal planes and compared with the digitally reconstructed radiographs from the initial planning CT. The patient is then shifted in order to align bone landmarks, with the assumption that the soft tissues will track with the bone. The positioning of both soft tissue and bone can be assessed using cone beam CT (CBCT), which provides 3-dimensional IGRT. In kV CBCT, the gantry makes a single rotation around the patient and provides 3-dimensional images, although several factors contribute to the CBCT image quality being inferior to diagnostic-quality CT images. One of the challenges in utilizing IGRT in SCCHN is that different regions within the treated area have different daily variability in positioning. Therefore, the clinicians must decide upon the critical areas to use for any shifts in the treatment positioning during IGRT.
Adaptive Replanning
In addition to setup variability, there are also changes in patient and tumor anatomy that occur as tumors respond, patients gain or lose weight, or postoperative anatomy evolves. The changes can lead to reduced tumor dose and increased normal structures dose. These changes have led to the growing recognition of the importance of adaptive replanning (AR), which refers to adapting the plan to anatomic changes that occur during treatment.
The AR concept seems straightforward, yet the practical application is complex. One issue is that gross tumor volumes generally do not shrink symmetrically and may change position over time. One approach is deformable image registration, which uses the original delineated tumor and normal tissue volumes deformed onto the new image set. However, the most common method of deformable registration does not consistently capture the change in tumor over time, even though it does well in capturing changes in normal tissue positioning.
There are additional challenges to clinically implementing AR:
- •
There are no clear-cut criteria to select patients for AR; an important line of research is to use the 3-dimensional CBCT images to reconstruct the radiation dose distribution for that day
- •
AR has the potential to be prohibitively resource intensive, with contouring a standard IMRT case taking an average of 144 minutes, without factoring in the time for IMRT planning and quality assurance
Therefore, AR algorithms are essential to facilitate routine RT replanning.
Alternative Photon Delivery
Standard IMRT delivers radiation through static fields at different angles around the patient, either using fixed multileaf collimators (MLCs) or dynamic MLC that move during treatment. Two new approaches to photon delivery involve radiation that is delivered in a 360° rotation or in smaller arcs around the patient: helical tomotherapy (HT) and volumetric modulated arc therapy (VMAT). Despite being advertised as superior, there are no randomized prospective data showing a clinically significant difference in oncologic or toxicity outcomes. Multiple dosimetric studies have compared the different methods of delivering photons with variable claims of superiority. These studies need to be interpreted with care due to bias introduced by variation in planner experience with the different planning strategies, limitations in different optimization software packages, and different emphasis on which metrics are to be used in assessing clinically significant differences (eg, homogeneity index, conformality index, and dose to a specific organ at risk).
Helical Tomotherapy
Helical tomogherapy (HT), first used at the University of Wisconsin, combines the properties of a helical CT scanner with a megavoltage photon linear accelerator that delivers RT by rotating around the ring gantry while the patient moves through the bore of the machine. There are distinguishing features of HT:
- •
Image guidance is built into the treatment itself as exiting radiation is detected for image reconstruction
- •
The delivery is given over the full 360° and can provide homogeneous tumor dose while providing steep gradients between tumor and critical organs
Limitations of HT are that noncoplanar treatment angles are typically not used, and it results in more low-dose irradiated tissue than some IMRT plans, although the clinical significance of this is unknown.
VMAT currently includes 2 major delivery units, the Elekta VMAT (Stockholm, Sweden) and Varian RapidArc (Palo Alto, CA). Unlike HT, where the beam of radiation is a fan and the volume is treated slice by slice, VMAT uses a cone beam, and apertures are designed to account for the entire treated volume. Challenges of arc therapy include the relative complexity of the plan optimization software, which can make treatment planning more tedious than for static field IMRT. VMAT provides the fastest treatment time and the lowest number of monitor units, which may theoretically lead to a decreased risk of second malignancy. However, there are no supportive clinical data at this point. The high dose rate to the tumor provided by both forms of rotational therapy (VMAT and HT), compared with static-field IMRT, has led some researchers to postulate an increase in tumor control probability.
Proton Therapy
Proton therapy holds promise for maximizing the TR in SCCHN. Protons and photons have similar biologic properties, but the difference in physical dose distribution distinguishes them and may have an effect on clinical outcomes. The dose from a photon beam decreases exponentially with depth in tissue resulting in nonessential radiation beyond the target. In contrast, a proton beam delivers most of its dose at a specified range in tissue with essentially no dose beyond the point of maximum dose deposition, known as the Bragg Peak, which can be specified through the treatment planning process.
Over the last 10 years there has been dramatic expansion of the number of proton facilities worldwide. For example, there were only 29 operational facilities in 2008 compared with 46 facilities as of November 2014. This number will likely continue to increase due to the development of smaller and less costly delivery units.
Clinical Experience
There are no completed randomized trials comparing proton and photon radiotherapy for SCCHN. Currently, a phase 2/3 randomized trial sponsored by the University of Texas MD Anderson Cancer Center (UTMDACC) is accruing and will compare intensity-modulated proton beam therapy (IMPT) with IMRT for oropharyngeal cancer with a primary outcome of toxicity ( NCT01893307 ).
Sinonasal malignancies
The Massachusetts General Hospital treated 102 patients with advanced sinonasal cancers and a range of histologies using proton therapy from 1991 to 2002. The 5-year local control (LC) was 86%, which is impressive considering the advanced stages and inherent challenge of treating cancers so close to the brain, brainstem, and optic structures. Researchers from Japan published outcomes with hypofractionated proton therapy for unresectable T4 nasal cavity tumors with favorable 1-year LC of 77%, with 1 treatment-related death and 12.8% grade 3–5 toxicity.
Nasopharyngeal cancer
Early prospective data suggest that proton therapy for nasopharyngeal carcinoma may result in a meaningful reduction in acute and possibly late toxicity. The first prospective phase 2 study of proton therapy for nasopharyngeal carcinoma showed that swallowing function compared favorably with historical outcomes, especially given the advanced nature of the tumors. A recent case–control study of patients treated with IMPT and IMRT at UTMDACC and Linkou Chang-Gung Memorial Hospital, Taiwan, showed a significantly lower proportion of gastrostomy tube insertion in the IMPT group (23.1% vs 57.7%).
Oropharyngeal cancer
Loma Linda University used protons as a boost after a predominantly photon-based treatment for stage 2 to 4 oropharyngeal cancer. The 2-year LC was 93%, and the 2-year RTOG grade 3 toxicity was 16%; it was difficult to interpret the relative contribution of protons, because most doses were given with photons. A UTMDACC abstract of a case–control study comparing 26 IMPT cases and 26 IMRT cases showed a lower rate of grade 3 dysphagia for IMPT.
Intensity Modulated Proton Therapy
The true potential for proton therapy to spare uninvolved tissue has not yet been realized, but there is promise in the form of optimized IMPT. Therapeutic proton beams are generated using passive scattering of a mono-energetic beam or with pencil beam scanning where magnets are used to steer the positively charged proton beam. IMPT, based on this scanning technology, has the potential to provide superior dose conformality and normal tissue sparing than other forms of proton or photon delivery. In IMPT, a small circular proton beam, characterized by the beam spot size, is scanned across the defined treatment field with varying beam energy and intensity. The conformality of an IMPT treatment plan has a direct dependence on the spot size and also on the strategy of how spots are placed within the patient. Current research is aimed at optimizing the ability to deliver proton therapy by accurate manipulation of the pencil beam, and in minimizing the effective spot size of the beam to increase the dose conformality and minimize the amount of normal tissue receiving radiation.
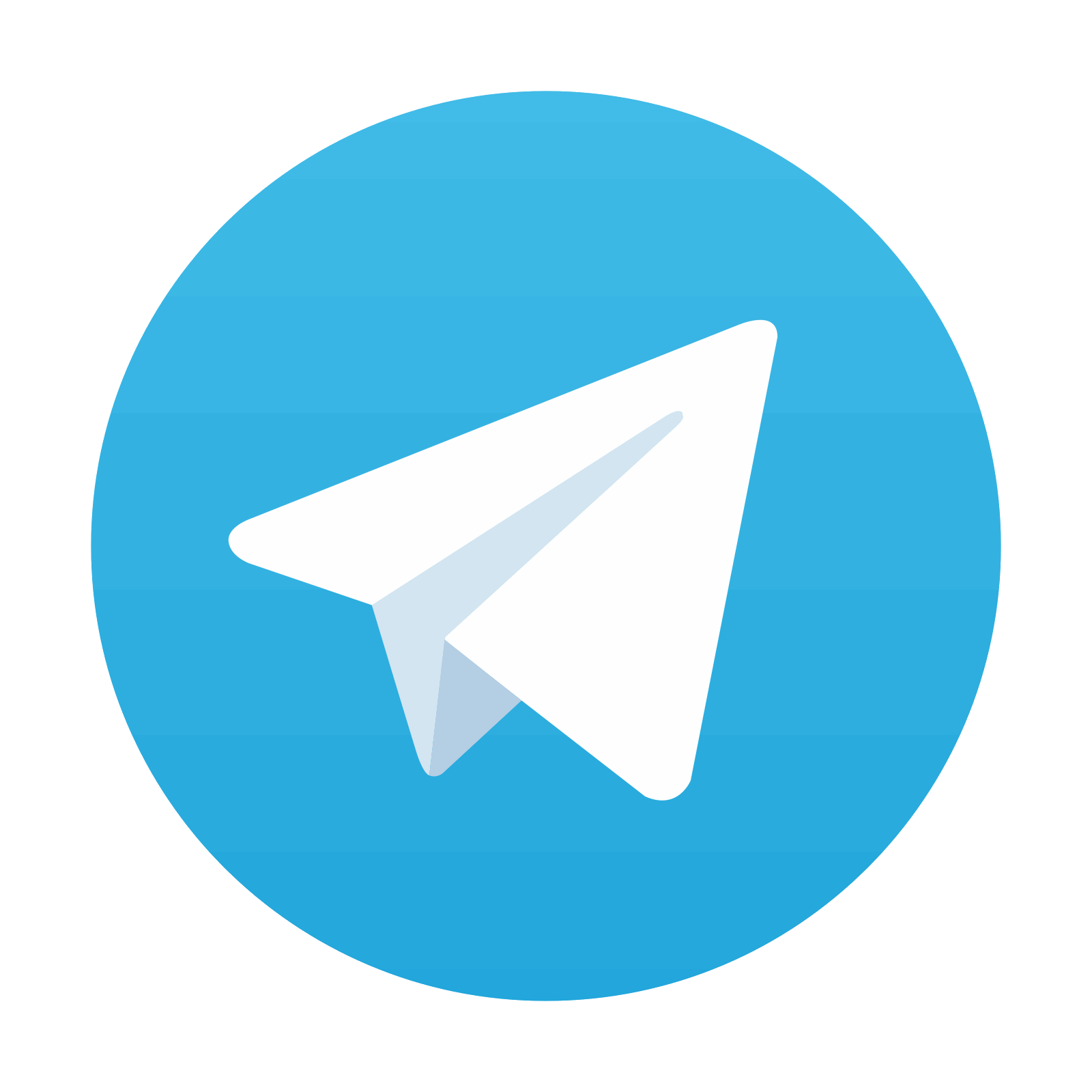
Stay updated, free articles. Join our Telegram channel

Full access? Get Clinical Tree
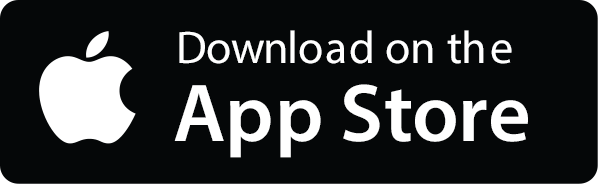
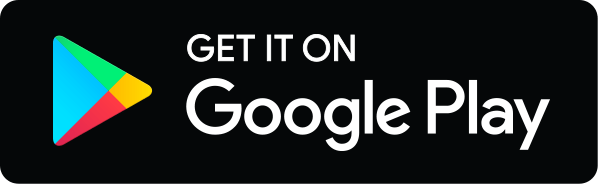