Radiation Oncology is the medical discipline that uses ionizing radiation with therapeutic intent in the management of cancer and selected benign disease. Compared to surgery, radiation is a modern medical treatment. Wilhelm Conrad Roentgen first described ionizing radiation as “a new kind of rays” or x-rays in 1895.1 The new modality was used for medical imaging within a month of its discovery and was applied to malignant disease within a few years of its discovery.
Radiation oncology encompasses a wide variety of treatment modalities and delivery methods including external beam radiation and brachytherapy, conventional fractionation and ablative therapies such as stereotactic radiosurgery (SRS), and stereotactic body radiation therapy (SBRT). Multiple types of radiation are used therapeutically including photons, electrons, protons, other particle therapy, and radioisotopes. The common mechanism of action is the deposition of energy in living tissue and subsequent damage to DNA. This chapter focuses on the more common modalities and treatments. The biology and physics of radiation therapy are well described elsewhere.2,3
Radiation oncology is an integral component of multidisciplinary cancer care. The goal of radiation therapy is to deliver the prescribed dose of radiation to a defined tumor target volume with minimal harm of the normal surrounding tissues. With advances in physics, treatment planning software, and treatment delivery systems, we have approached that goal in the last few decades.
The most common treatment delivery method is external beam radiotherapy (EBRT). EBRT is delivered with a medical linear accelerator or Cobalt machine. Modern linear accelerators treat with photons, high energy uncharged particles, and electrons, charged particles with limited depth of penetration.
Prior to the 1990s the predominant method of treatment planning and delivery was based on two-dimensional (2D) images and plans. The treatment volume was imaged with plain x-rays or fluoroscopy and a 2D treatment plan through a central plane was created (Fig. 11-1). For some sites (whole brain, pelvis, larynx), treatment fields were defined clinically by external landmarks and a port film was obtained to confirm the radiation field. This method of treatment planning and delivery is still widely used in low- and medium-resource countries. Advantages are simplicity and cost. Disadvantages are increased variation in treatment delivery (less accurate) and increased volume of normal tissue irradiated, with potential increase in long-term side effects, diminished quality of life, and future medical costs. The foundation of modern radiation oncology practice was established with 2D treatment planning and effective treatments were developed for multiple tumor sites including head and neck, cervix, lymphoma, and breast cancer.4–7
During the 1990s the wide availability of CT scanners and development of treatment planning software ushered in the era of 3D treatment planning. The simulation process, obtaining images for radiation planning, is done on a CT scanner. This provides a target volume instead of a 2D field. Treatment planning software displays dose-volume histograms (DVH) that provide information about dose to target volumes and organs at risk (OAR). Digitally reconstructed radiographs (DRR) based on CT slice data generate an image that can be used as a reference for verification of treatment accuracy. 3D-based treatment planning gives radiation oncologists volumetric dose information and the capability of conforming the radiation to the target, avoiding normal structures, and potentially reducing acute and chronic side effects.8,9 This is the predominant treatment method in developed countries.
Intensity-modulated radiation therapy (IMRT) became widely available during 2000 to 2010 and is now the preferred method of treatment planning for head and neck, prostate, and other selected sites.10,11 This method uses inverse planning. The process starts with an ideal dose to the tumor and constraints to normal tissues and OAR such as lung, kidney, and spinal cord. The planning program uses multiple iterations to produce the desired beam characteristics, and produces the best approximation of an ideal dose defined in a 3D array of dose voxels. IMRT allows a high degree of conformality, but steep dose gradients may create a higher risk of underdose or overdose to targets and OAR (Fig. 11-2). Safe implementation of IMRT requires reproducible immobilization methods and image guidance to confirm accurate treatment delivery as well as robust quality assurance protocols.12 At inception IMRT treatment planning and delivery were resource intensive, but advancements in treatment planning and delivery have reduced both planning and treatment time.
Image-guided radiation therapy incorporates imaging to plan, initiate, and monitor radiation therapy. Most contemporary radiation therapy in the United States is image guided in the sense that the treatment plan is based on a CT image. A variety of imaging modalities are available to plan and monitor treatment at the beginning of each treatment and sometimes during treatment delivery.13,14 IGRT is critical to safe implementation of IMRT and SBRT.
The term fractionation refers to the amount of radiation that is delivered in a single-treatment session. In the United States, the term “standard fractionation” refers to the delivery of 1.8 to 2 Gy per fraction per day. This dose has been determined empirically and supported by radiobiological principles to provide tumor control and respect normal tissue tolerance when delivered to an appropriate total dose in sequential daily treatments.15 Standard fractionation is used with 2D, 3D, and many IMRT treatments in multiple sites with curative intent, such as breast, prostate, pelvic, and GI malignancies. Ablative techniques such as SRS,16 SBRT,17 and intraoperative radiation therapy (IORT)18 do not use standard fractionation. In these situations, precise, focal radiation delivery with protection or avoidance of normal structures allow for higher ablative doses of radiation.
Modern cancer treatment is interdisciplinary. Approaches to systemic and local control, and concerns for access, cost, toxicity, and quality of life, require a multidisciplinary approach to cancer management.
Radiation can be used as definitive therapy (prostate cancer) or in conjunction with systemic therapy (head and neck, cervix cancer). It can be used preoperatively (rectal cancer) or postoperatively (following breast conserving surgery). Radiotherapy may provide an alternative to surgery for patients that are deemed medically inoperable (SBRT for early lung cancer).
This term covers a range of treatment modalities including electrons, low-energy photons and high-dose rate (HDR) brachytherapy. The common aspect is that the radiation is delivered during an operative procedure. Potential advantages are that the target and avoidance structures can be visualized in situ and with appropriate techniques the normal tissues can be shielded or moved away from the radiation field. Normal tissue protection allows higher effective dose of radiation to the tumor. IORT has been used in multiple tumor sites, often in combination with external beam radiation therapy (EBRT).
IORT has been extensively used in the treatment of patients with advanced and recurrent colorectal cancer, though no randomized prospective studies are available. Data from multiple trials suggest a benefit for local control in patients with fixed T3 and T4 tumors.19 Single-institutional data from the Mayo Clinic suggest survival and local control benefit for patients with recurrent colorectal cancer.20 In patients with unresectable pancreatic cancer a review of single-institutional studies found that the combination of IORT plus EBRT plus chemotherapy was associated with better pain control but had no significant benefit for median or overall survival.21 IORT has been used in the management of soft tissue sarcomas arising in multiple sites. One small, randomized trial demonstrated improved local control with IORT versus EBRT, but no improvement in freedom from metastases or overall survival.22 A review of IORT literature for soft tissue sarcomas suggests that IORT contributes to improved local control, particularly in patients with gross residual disease or positive margins.23
Several large randomized studies have tested the effectiveness of IORT in the treatment of breast cancer. The rationale for this procedure, and other forms of partial breast irradiation, is that the majority of local recurrences following radiation are adjacent to the index lesion. The phase 3 TARGIT-A trial used the Intrabeam treatment system with low-energy photons (maximum 50KV) to deliver 50 Gy to the breast tissue in contact with the applicator following lumpectomy, prior to completing the surgery. This single treatment was compared to standard EBRT. Criteria included age over 45 and defined low-risk features. The trial included over 2000 women. Patients treated with IORT found to have high-risk features on final pathology also received EBRT. At 4 years there was no significant difference in local control; morbidity was lower in the TARGIT group.24 Longer follow-up has shown a higher rate of in-field recurrence in the IORT-alone group (3.3% vs. 1.3%), but is still low with no difference in mortality.25 Intraoperative electron radiation therapy (IOERT) has been investigated in the ELIOT trial. 26 This study enrolled more than 1800 women with early breast cancer. The dose prescribed was 20 Gy to the 90% isodose line. Unlike the TARGIT trial, patients did not receive additional EBRT based on final pathology. After a median follow-up of 5.8 years the event rate of in-breast tumor recurrence (IBTR) was 4.4% in the IOERT group compared to 0.4% in the external beam whole-breast radiation group, but within the prespecified equivalence margin. Data from the TARGIT-A and ELIOT trials support the hypothesis that intraoperative radiation may be a reasonable alternative to whole-breast radiation in selected low-risk patients with breast cancer after breast conserving surgery. However, the updated results from TARGIT-A demonstrate continued increase in local recurrence with longer follow-up. Since women with early breast cancer may live decades after diagnosis and initial treatment, some caution is warranted in interpreting these early results.
Adapted from the concepts of intracranial SRS, stereotactic body radiation therapy (SBRT) was developed in the 1990s to deliver ablative doses of radiation in a few fractions to extracranial tumors to optimize their local control following irradiation.27 In SBRT, a sharp dose gradient is generated at the periphery of the tumor target volume to ensure adequate normal tissue sparing. Tumor target localization is essential for the accurate and safe delivery of stereotactic ablative radiotherapy (SABR), as very small setup margins are used in this setting to minimize normal tissue toxicity. This is achieved through accurately accounting for internal target motion (physiological motion, such as normal breathing) of the tumor and appropriate patient immobilization to minimize potential setup errors during treatment planning and the daily delivery of treatments,28 as well as daily image guidance to verify tumor localization for each treatment fraction.29
In the thorax, SBRT has emerged to become an accepted alternative to lobectomy for the treatment of early-stage non-small-cell lung cancer (NSCLC). Local failure has been found to be less than 20% in general with most studies reporting excellent local control of 90% or above following SBRT in stage I NSCLC.30 In the first North American multicenter phase II study (RTOG 0236), 54 Gy was delivered in 1.5 to 2 weeks in three daily fractions in medically inoperable patients with biopsy proven peripheral T1-2, N0, M0 NSCLC <5 cm in diameter.31 In 55 of the 59 evaluable patients enrolled on this study, only one local failure was observed after a median follow-up of 34.4 months (T2, N0, M0), while 3 patients recurred within the involved lobe. At 3 years, the local control and lobar control rates were 97.6% and 90.6%, respectively. Only two regional failures were observed at 33 and 36.1 months following treatment, while distant metastasis was observed in 22.1% of patients at 3 years. These findings have been fairly consistent with what has been observed by other investigators.30 Multiple dose fractionation schedules are used for SBRT in the treatment of early-stage NSCLC. Multi-institutional experience from Japan, North America, and Europe has suggested that the most optimal local control of above 90% is achieved when the biologically effective dose (BED) that is delivered to the tumor is greater than 100 Gy10.32,33 In this setting, Gy10 is the radiation unit Gray calculated with a α/β ratio of 10 Gy. The α/β ratio is a parameter used in the calculation of the BED that may likely represent the ratio of intrinsic tissue radiosensitivity and the same tissue’s repair capacity. The details of BED calculation have been previously described.34 It is the total dose required to give the same amount of tumor cell killing as a specific dose fractionation schedule, at an infinitely low dose rate or with infinitely small fractions well-spaced out.34,35 BED serves as a common dose parameter in the comparison of various dose fractionation schedules.
In thoracic SBRT, which is also known as stereotactic ablative radiotherapy (SABR), tumor location has been classified as central or peripheral. Central location has been previously defined as 2 cm in all directions from the proximal bronchial tree, which is the distal 2 cm of the trachea, carina, and lobar bronchi up to their first bifurcation in previous RTOG trials. SBRT for central lung lesions has been controversial due to previously reported treatment-related deaths.30 In a phase II study from Indiana University, medically inoperable patients with T1-2, N0, and M0 NSCLC up to 7 cm were treated with 60 to 66 Gy in three fractions.36,37 Six treatment-related deaths were reported after a median follow-up of 17.5 months in this study, and central tumor location was found to have an 11-fold increase in the risk of severe toxicity when compared with peripheral location.36 No significant increase in severe toxicity was observed in this study after a median follow-up of 50.2 months, which was most likely due to the low incidence of severe toxicity observed overall.37 However, the incidence of severe toxicity was 27% versus 10.4% favoring peripheral tumor location after long-term follow-up, despite the lack of statistical significance. SBRT has been shown to be feasible and safe for the treatment of centrally located lung tumors when fractional dose has been kept low and dose constraints have been strictly respected to exclude critical thoracic organs immediately adjacent to the tumors from the high dose volume.38–41
When compared to surgery, SBRT has been found to be at least equivalent to lobectomy in multiple studies in the treatment of early-stage NSCLC.42–44 As shown in a Japanese multi-institutional study of operable patients with stage I NSCLC, the 5-year survival for stage IA and IB patients were 72% and 62%, respectively, which appears to be at least equivalent if not better than historical surgery data.42 More recently, a multi-institutional study comparing video-assisted thoracic surgery (VATS) lobectomy and SBRT for stage I–II NSCLC has demonstrated the locoregional control to be superior after SBRT (93.3% vs. 82.6% at 3 years, p = 0.04) while overall survival has been equivalent between the two treatment modalities.44 When compared to wedge resection for stage I NSCLC in a single-institutional study, SBRT has been shown to be associated with significantly lower incidence of local recurrence (5% vs. 24%, p = 0.05) after a median follow-up of 2.5 years.45 Current evidence suggests SBRT is comparable to lobectomy and may be superior to wedge resection in operable patients with stage I NSCLC.
Lung SBRT has also been used to treat isolated recurrences of NSCLC and metastases from various origins. In a phase I/II study, patients with up to three lung metastases from various origins were treated with 48 Gy to 60 Gy delivered in three fractions without any grade 4 and 5 toxicity.46 An actuarial local control of 96% was achieved at 2 years in this study. In a phase II study from Europe, 3-year local control and overall survival of 83.5% and 52.5%, were achieved following SBRT in patients with one to two lung metastases and no active extrathoracic disease, which also supports the use of SBRT for selected patients with limited metastasis to the lungs.47
More recently, SBRT has also been adopted to treat oligometastases and solid tumors in extrathoracic locations, such as the spine, the abdomen, and the pelvis. Recent experience demonstrated rapid and durable pain relief with excellent local control for patients with painful spinal metastases following SBRT.48,49 Spinal SBRT may be a good treatment option for patients with limited spinal metastases. However, there is a risk of vertebral compression fraction associated with this treatment approach which can be as high as 20%.50,51
In the abdomen, SBRT has been used for the treatment of metastases to the liver. Excellent local control with acceptable toxicity profile has been reported in single-institutional and early-phase studies.52–54 This approach may be beneficial for medically inoperable patients with limited number of liver metastases from a colorectal primary. SBRT has been used to treat adrenal metastases and abdominal nodal metastases with excellent local control and toxicity profile.55,56
In the definitive treatment of abdominal tumors, SBRT has been used sequentially with gemcitabine to achieve a local control of 94% at 1 year in patients with locally advanced nonmetastatic pancreatic cancer.57 It was also found to be able to convert 56.1% of borderline resectable pancreatic cancer (BRPC) patients to resectable with 96.9% of them achieving R0 resection in a single-institutional study by Chuong et al.58 In this study, the BRPC patients who underwent R0 resection were found to have an increase in median survival of 7 months (p = 0.03). Excellent response rates were observed when SBRT was initially used to treat hepatocellular carcinoma (HCC) in the 1990s.27 An increasing number of reports on this subject have been published since then to characterize the application of SBRT in the treatment of HCC.59–62 Good local response with low incidence of radiation-related toxicity has been observed in general. In a recent prospective phase I/II study from Princess Margaret Hospital, local control of 87% was observed following SBRT for 102 Child-Turcotte-Pugh class A patients with stage I–IV HCC.62 In this study, over 70% of the patients had stage III–IV disease, and the 1-year survival rate of 55% was found to be at least comparable to that following sorafenib in similar patient populations. However, seven possibly treatment-related deaths were observed, which warrant further investigation. Given its excellent results, a prospective randomized phase III trial comparing sorafenib and SBRT followed by sorafenib in patients with HCC is currently ongoing to investigate the benefit of combining SBRT with sorafenib in the treatment of HCC (RTOG 1112).
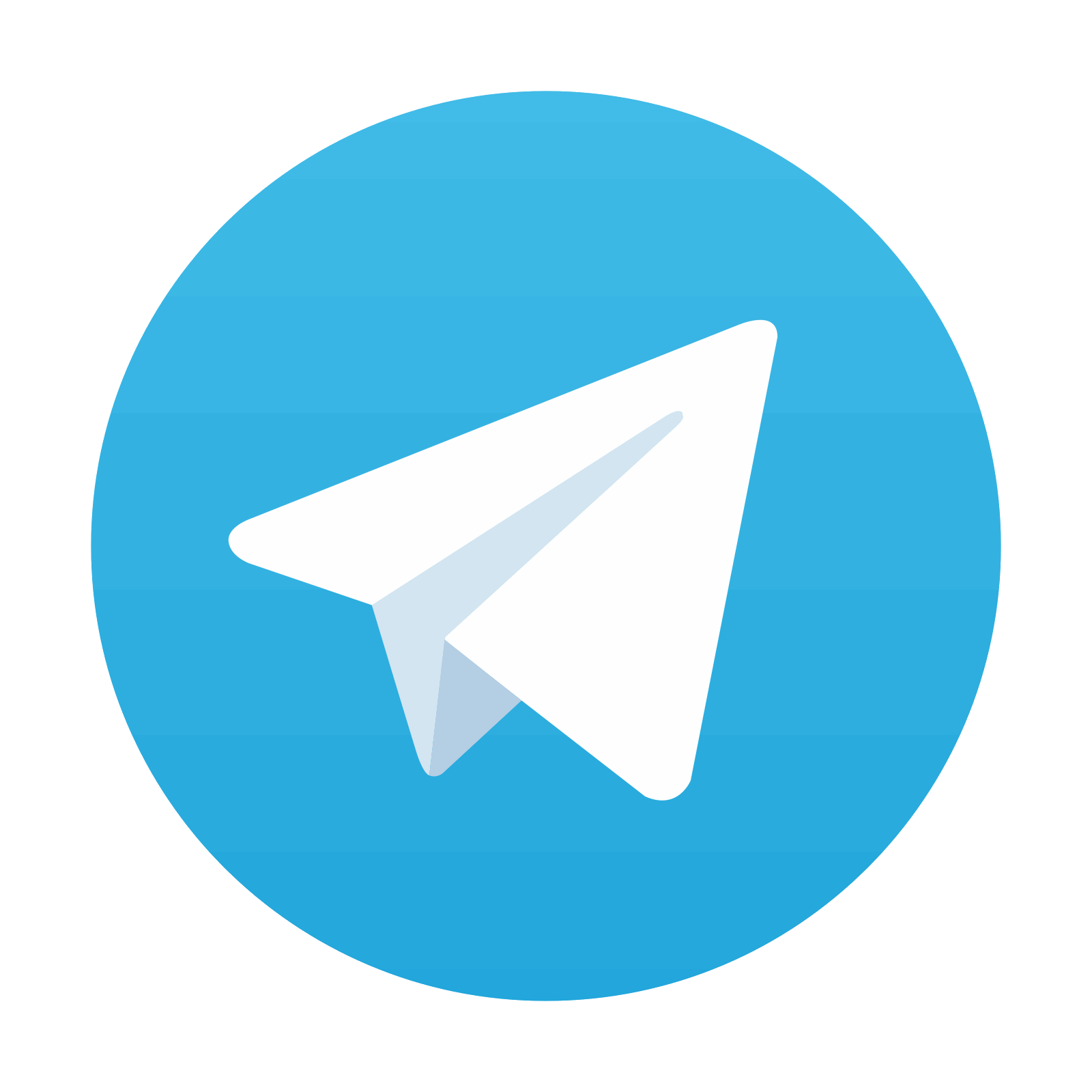
Stay updated, free articles. Join our Telegram channel

Full access? Get Clinical Tree
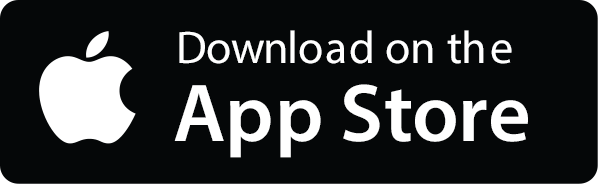
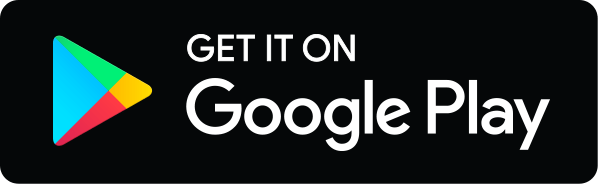