© Springer International Publishing AG 2017
Gokhan Ozyigit and Ugur Selek (eds.)Principles and Practice of Urooncologyhttps://doi.org/10.1007/978-3-319-56114-1_2626. Quality Assurance of Modern Radiotherapy Techniques in Prostate Cancer Treatment
(1)
Department of Radiation Oncology, American Hospital Texas MD Anderson Cancer Center, Istanbul, Turkey
(2)
Department of Radiation Oncology, The University of Texas MD Anderson Cancer Center, Houston, TX, USA
Abstract
Prostate cancer is one of the most prevalent malignant diseases that occur among men with a new case diagnosed every 2.2 min, affecting one in six men their lifetime. Radiotherapy has been a vital part of the treatment of prostate cancer with three-dimensional conformal radiotherapy (3DCRT ) as the historical standard. By utilizing techniques such as intensity-modulated radiation therapy (IMRT ) or volumetric modulated arc therapy (VMAT ) with image-guided radiotherapy (IGRT ), the amount of normal tissue treated can be reduced and thus limit this increased toxicity. These types of treatments use the multi-leaf collimator to create a sequence of small apertures to deliver a high dose to the tumor. The treatment plans are created so that a high dose is given to the target while keeping dose to organs at risk low. This requires a treatment planning system (TPS) that has the capabilities of inverse planning to generate such treatment fields. The inverse planning engine uses as input contours of the targets and OARs to generate an optimal plan. With new technologies comes a new set of quality assurance (QA ) tasks that need to be performed to ensure that what is being planned in the TPS is actually what is delivered to the patient. In this chapter, we will encompass the simulation, planning, and treatment of tumors located in the prostate region. In addition we will discuss the QA methods of each component of the radiation therapy process when treating tumors in the prostate region.
Keywords
RadiotherapyProstate cancerQuality assurance26.1 Patient Simulation
26.1.1 Setup and Immobilization
Prior to beginning radiation treatment, all patients undergo a 3DCT simulation. A few days before CT simulation, several marker seeds are be inserted into prostate tumor volume. These markers help to more precisely locate prostate during each radiation treatment session. CT simulation allows us to visualize the tumor and other anatomy in 3D. CT numbers are proportional to electron density, which is necessary in heterogeneous dose calculation algorithms. Patients are brought to the simulator and positioned on the CT couch. At this point, immobilization devices are created to make sure that the patient can be set up in a reproducible position for treatment. Patient immobilization is a key component to the radiation treatment process. Complex treatments are good only if they are treating the area that is intended. That includes setting the patient up in an accurate, reproducible, and comfortable manner for the patient so that they can be positioned in the exact same way each day they come for treatment. Patients are simulated with CT in supine position on a flat tabletop. A custom-formed Vak-Loc bag is utilized to ensure consistent setup and stabilization. The bladder at time of simulation is filled to a degree that are maintainable and reproducible for daily treatment [1]. These immobilization devices must comfortably allow the patient to be positioned reproducibly each day during the course of radiation therapy [2–4].
26.2 Patient Planning
Historically prostate tumors were treated, with either an AP/PA beam arrangement and/or a series of oblique beams. Treatment plans were run utilizing manually collected single contours and calculations were performed with the use of heterogeneity corrections. As technology has evolved, so have the treatment planning strategies. Complex treatment plans using intensity-modulated radiation therapy techniques (IMRT ) and volumetric-modulated arc therapy (VMAT ) with full heterogeneity corrections has now become the standard [5–7]. The advances in imaging techniques and delivery techniques have aided in supporting the evolvement of treatment of tumors in the pelvic region. The advances in technology of imaging have been complemented by the improvements and advanced technology in treatment delivery. The primary focus on this improved technology has been the advent and development of the multi-leaf collimator (MLC) . IMRT and VMAT utilize the MLC on the linear accelerator to create small and complex beam apertures to deliver a high dose to the target while minimizing dose to surrounding tissues. These delivery methods are now standard of care for patients with prostate cancer and other pelvic malignancies. All of these advanced delivery techniques require a treatment planning system that can perform accurate dose calculations utilizing full heterogeneity corrections.
26.3 Consideration of Tumor Margins During the Planning Process
The type of imaging modality used at simulation, the treatment method to be used, the amount of tumor motion, and setup uncertainties should dictate the size of the margins placed on the tumor volume when generating the treatment plan. These margins are necessary to account for the fact that the tumor is not in the exact position it was at simulation for the reasons described above. Accounting for these uncertainties and/or tumor motion will ensure that the tumor will receive the full prescribed dose. ICRU 62 [8] describes a series of target volumes that are generated during the planning process. The first of which is the gross tumor volume (GTV), which is the gross disease visible on the CT data set. The clinical target volume (CTV) is generated by adding a margin around the GTV to account for microscopic disease that is not visible on the CT scan. An internal margin (IM) is added to the CTV to account for tumor and physiological motion to generate the internal target volume (ITV). Finally, expanding the ITV by a margin to account for geometric uncertainties in treatment generates the planning target volume (PTV).
Similar to the imaging process, the type of treatment and the immobilization devices used will also play a role in how treatment margins will be used to generate the PTV [9–11]. If margins are going to be reduced during the planning process, an increase in the imaging used to verify this reduced margin. The use of daily setup verification images will become necessary.
26.4 Practice of Plan Quality Evaluation
It is the physician’s responsibility to make clinical decisions regarding plan generation and plan evaluation. Upon completion of the treatment planning process, it is the physicist’s responsibility to ensure the overall quality of the treatment plan [12]. This involves several tasks, (1) overall physics evaluation of the treatment plan, (2) evaluation of planning criteria and dose volume constraints, and (3) independent verification of the dose calculation.
To evaluate the plan from a physics perspective, one must understand the limitations of the dose calculation algorithm. While this is not a comprehensive list of questions to ask for each treatment plan, the following provide examples of what should be evaluated as part of a physics plan check. Is the proper dose prescribed by the physician displayed on the CT slices in the treatment plan? Is the proper dose per fraction and number of fractions correct along with the correct energy? Is the dose normalization point located within the beam apertures and positioned where there is electronic equilibrium? Are the beams entering the patient on an overly sloped surface? Are the beam apertures sufficiently sized or overmodulated to ensure an accurate dose calculation? Was the correct CT data set used for dose computation? Were heterogeneous dose calculations used? These by no means are a complete list of items to look for, but it is the physicist’s responsibility to ensure that what is generated by the TPS accurately depicts the intentions of the directives given by the physician. Published DVH criteria are available for reference [13]; however, an institution may wish to develop and implement their own set of DVH criteria. In this case these criteria must be spelled out in the treatment planning directives. While there are circumstances where the DVH criteria may not be met, good clinical practice should include a review of the DVH constraints of each plan that is generated. If a DVH criteria is outside of those used in clinical practice, this should be discussed with the physician before the initiation of treatment.
26.5 TPS Dose Calculation Verification
Generating a treatment plan that is an accurate representation of what the linear accelerator can actually deliver first requires accurate imaging information and secondly requires accurate beam modeling. Validating the treatment planning system’s beam model is a critical component to the radiotherapy process [14, 15]. This task is completed prior to the release of the treatment planning for use in the clinical environment. A major component of the beam model verification and commissioning involves the verification of the dose computation algorithm [16, 17]. For static beams, common practice is to perform a hand calculation of the monitor unit settings based on basic machine parameters, percent depth doses (PDDs) or tissue-maximum ratios (TMRs) , output factors, inverse square correction, etc. This can be done either manually or with the use of automated software to perform such tasks. For more complex delivery techniques such as IMRT or VMAT deliveries where many irregularly shaped beam apertures are used in the patient’s treatment, measurements should be performed by delivering actual treatments planned with the linear accelerator. Several software vendors make products that can compute dose for IMRT and VMAT fields as a second check on the MU settings and the dose delivered. Once the commissioning of the beam modeling process is complete a routine QA program should be implemented. This comprehensive QA program should verify that the treatment planning system continues to perform as it did at the time of acceptance and commissioning of the system. The initial commissioning of the system as well as the QA program should include the following: (1) verification that the CT to density table is generated properly, (2) the TPS is able to accurately compute dose to simple and complex geometry fields as compared with measured data, (3) output factors generated by the TPS are accurate, (4) the contouring tools still perform accurately, (5) DVH generation remains accurate, and (6) data transfer to the record and verify system maintains the integrity of the intended treatment fields. These tests should be performed on a routine basis and are particularly important after an upgrade to the TPS software is made. Finally, in all cases, every patient and independent check of the treatment plan should be performed. This can be done with simple manual calculations of MU settings for simple cases. These manual calculations are prone to errors in that a typical photon beam in the prostate cavity traverses different areas of density often traveling between areas having different densities. In addition, irregularly shaped, small fields will lead to in accurate hand calculation of MU settings. Recently, secondary check software programs have been developed that are capable of a full 3D heterogeneous dose calculation. Such systems similar to the TPS will perform a full 3D dose calculation and independently compute the MU settings. These systems can provide information on both MU and dose calculations as well as DVH information. Such comprehensive systems are a valuable tool in verifying the TPS dose calculation [18, 19].
26.6 Patient-Specific QA
One of the most important part of prostate treatment with IMRT or VMAT is patient-specific QA . IMRT and VMAT treatment techniques have highly conformal dose distributions though MLC shape, gantry speed, and dose rate. Patient-specific QA is required to deliver the actual dose with accuracy [20]. There are several methods for patient-specific QA. Generally routine QA method for plan verification is done on a LINAC in a specific phantom using small volume ion chamber and an electrometer to measure the absolute dose or film dosimetry for relative dose [21]. Also two dimensional array (2D) and electronic portal imaging devices (EPID) which is known as portal dosimetry are used for performing patient-specific QA. New-generation EPID systems has amorphous silicon detectors. This system uses the portal imager to acquire fluence patterns of the patient treatment fields [22]. This data can be used to compute the dose and compare it to the TPS [23]. Recently, three-dimensional (3D) verification system which provide patient anatomical structure information is being start to use for patient-specific QA. These system captures the log files generated from the treatment machine and compute dose to the patient CT data set, which can be compared to the dose computed in the TPS.
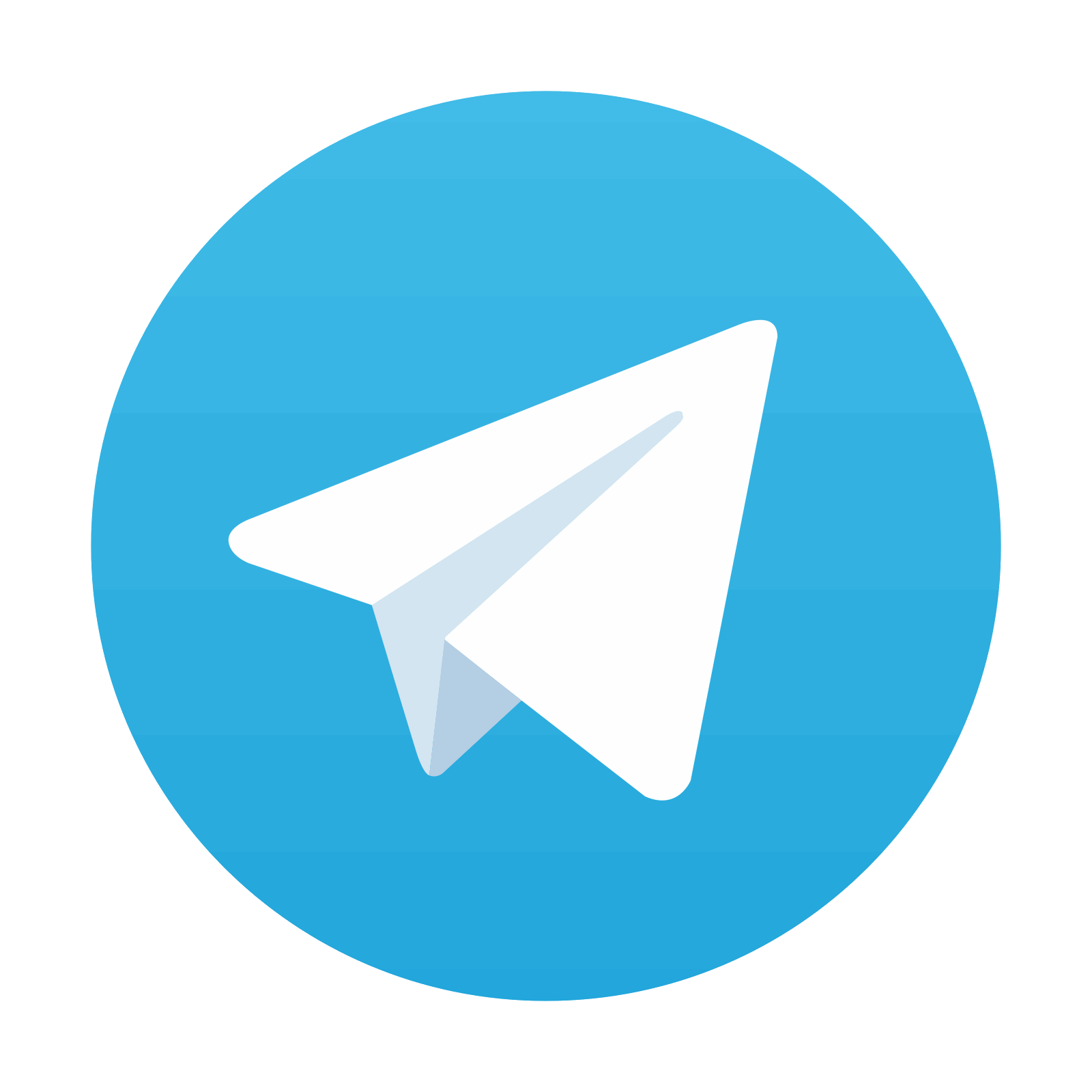
Stay updated, free articles. Join our Telegram channel

Full access? Get Clinical Tree
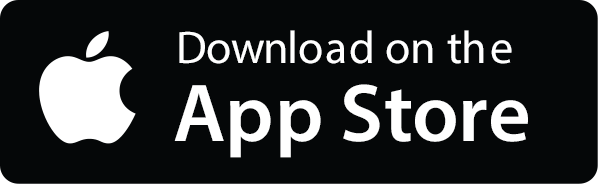
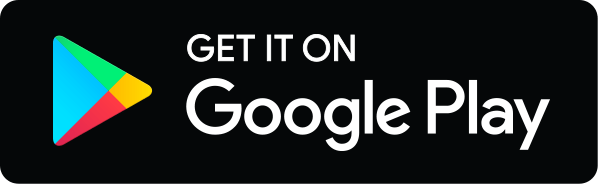