Fig. 19.1
Dose deposition characteristics of protons vs. X-rays
Because of the absence of an exit dose, proton therapy can be used to treat cancer patients with less scatter radiation dose compared to X-rays. For prostate cancer patients treated with protons, the most common beam arrangement used for treatment is two beams coming in from the right lateral and left lateral directions. In contrast, X-rays with intensity-modulated radiation therapy (IMRT) use multiple angles or arcs to be able to concentrate the radiation dose around the prostate. This means that there is low-dose scatter to the rest of the pelvis, including to the bladder and rectum.
19.3 Modes of Proton Delivery
Currently there are two types of proton therapy delivery: passively scattered proton therapy and pencil beam proton therapy . Passively scattered proton therapy consists of a monoenergetic beam of protons, which is scattered by a snout to a square-shaped beam. As mentioned before, the Bragg peak is usually spread out to cover the entire tumor with margin in the direction of the beam using a range modulator wheel. This beam is shaped by a custom aperture (usually made of brass) and a custom tissue compensator (usually made of acrylic or wax), unique to the patient’s anatomy. The aperture determines the shape of the radiation field (akin to multileaf collimators for X-rays), and the compensator shapes the distal edge of the proton beam dose to the shape of the target volume (Fig. 19.2).


Fig. 19.2
Hardware used for passively scattered protons (from left, range moderator wheel, aperture, tissue compensator)
Pencil beam proton therapy (also known as actively scanned proton therapy or spot-scanning proton therapy) uses a small proton beam (“pencil beam”) to deliver dose by sequentially layering multiple pristine Bragg peaks (or “spots”). An electromagnetic field is used in the pencil beam to scan the protons in both directions perpendicular to the beam direction (i.e., X and Y axes) without the need for a scattering device [6–8]. Dose delivery in the distal and proximal directions (i.e., Z axis) is achieved by changing the energy of the pencil beam and delivering multiple layers of dose, instead of using a range-modulator wheel. Because this layering of dose allows for better conformity of the proximal edge of the target volume compared to passively scattered proton therapy , there is improved normal tissue sparing.
Pencil beam proton therapy has several advantages over passively scattered proton beam therapy. Pencil beam allows for improved conformality of the proton dose with improved tumor coverage and normal tissue sparing, especially around curved structures. The pencil beam does not require the use of brass apertures and acrylic compensators, which saves time and effort normally needed to make such hardware. It also makes it easier and faster for radiation therapists to treat the patient, as they no longer need to mount the hardware on the treatment machine. Because each beam angle with the passively scattered proton therapy needs its own set of aperture and compensator, the number of beam angles that can be used per patient is limited. With the pencil beam, there is less of a limit on how many angles can be used for the treatment. Furthermore, the hardware in the pathway of the passively scattered proton therapy (which includes the range-modulator wheel, aperture, and tissue compensator) leads to increased neutron production. Therefore, the pencil beam proton therapy also has the advantage of decreased neutron dose, which should decrease the risk of affecting implanted medical devices and the risk of secondary cancers. Finally, the pencil beam is required for intensity-modulated proton therapy (IMPT) using multifield optimization. IMPT allows for further improvements in dose conformality and simultaneous integrated boosts (SIB) of the gross tumor. However, the pencil beam plans require more rigorous quality assurance to make sure that the dose delivery matches the treatment plan.
The main difference between a pencil beam plan and a passively scattered plan for treatment of a prostate cancer patient is that the radiation dose lateral and posterior to the prostate is much more conformal with the pencil beam. Most of the proton therapy centers now have pencil beam available for treatment. In fact, the newest proton therapy centers are being built with only pencil beam capability (and will no longer have passively scattered proton beam). Figure 19.3

shows the difference between plans using passively scattered and pencil beam proton therapy.

Fig. 19.3
Difference between treatment plans using passively scattered (a) and pencil beam (b) proton therapy
19.4 Clinical Outcomes
Several studies have shown the benefit of dose escalation in the treatment of prostate cancer. Kuban et al. randomized 301 patients with stage T1b–T3 prostate cancer to either 70 or 78 Gy using X-ray therapy. At a median follow-up of 8.7 years, the patients who received 78 Gy had an improved freedom from biochemical or clinical failure of 78% compared to 59% in patients who received 70 Gy (p = 0.004) [4]. There was also improvement in the clinical failure-free survival in the 78 Gy arm (93% vs. 85%, p = 0.014), but there was no overall survival benefit. Unfortunately, the 78 Gy arm was also associated with higher toxicity, with the rate of RTOG grade 2 or higher gastrointestinal (GI) toxicity being 26% vs. 13% (p = 0.013). There was no significant difference in the RTOG grade 2 or higher genitourinary (GU) toxicity (13% vs. 8%).
The Dutch Multicenter Trial randomized 669 patients with localized prostate cancer to either 68 or 78 Gy using X-ray therapy. After a median follow-up of 70 months, the 7 year freedom from failure was improved in the 78 Gy arm compared to 68 Gy (54% vs. 47%, p = 0.04) [9]. Once again, there was an increased late grade 2 or higher GI toxicity seen in the 78 Gy arm compared to the 68 Gy arm (35% vs. 25%, p = 0.04). There was no significant difference in the late grade 2 or higher GU toxicity (40% vs. 41%, p = 0.6).
The Proton Radiation Oncology Group (PROG) 95–09 study randomized a total of 393 patients with stage T1b–T2b with PSA <15 ng/mL at either Loma Linda University Medical Center (LLUMC) or Massachusetts General Hospital (MGH). All patients received 3D conformal X-ray therapy to the prostate and seminal vesicles to a dose of 50.4 Gy, followed by either a proton boost of 28.8 cobalt Gy equivalent (CGE) or 19.8 CGE. Therefore, the patients were randomized to either 79.2 CGE or 70.2 CGE. At LLUMC, < patients were treated in the supine position using opposed lateral 250 MeV proton beams. At the MGH, patients were treated in the lithotomy position using a single transperineal 160 MeV proton beam. At a median follow-up of 8.9 years, patients who received the 79.2 CGE were significantly less likely to have local failure with a hazard ratio of 0.57. The 10 year biochemical failure rate using the ASTRO definition was 16.7% for the 79.2 CGE arm and 32.4% for the 70.2 CGE arm (p = <0.0001). The patient reported outcomes using the prostate cancer symptom indices (PCSI) were published in a separate publication. At a median of 9.4 years, there was no difference in urinary obstruction/irritation (p = 0.36), urinary incontinence (p = 0.99), bowel problems (p = 0.70), or sexual dysfunction (p = 0.65) [10]. Unfortunately, the PROG 95–09 was not a randomized study comparing protons to X-rays; all of the patients received a combination of photons and X-rays. However, it is interesting to note that there is no increased GI toxicity seen with dose escalation in this study where proton therapy was used.
Several single-institution reports on the outcomes of prostate cancer patients after proton therapy have been published. Slater et al. published the LLUMC experience of 1255 patients treated between October 1991 and December 1997. Patients with 15% or greater risk of having pelvic lymph node metastasis by the Partin tables were treated with a conformal “boost” using protons to a dose of 30 CGE in 15 fractions which was given to the prostate and seminal vesicles, followed by a conformal treatment using X-rays to a dose of 45 Gy to the prostate, seminal vesicles, and the first- and second-echelon lymphatics. Patients who did not have this risk were treated with proton therapy only to a total dose of 74 CGE in 2 CGE fractions. These patients were treated with a rectal balloon placed daily, usually with one field per day. With a median follow-up of 62 months, the overall biochemical disease-free survival was 73% [11]. In patients with initial PSAs ≤ 4.0, it was 90% and in patients with posttreatment PSA ≤0.5, 87%. The actuarial 5 year and 10 year rates for freedom from grades 3 and 4 GI toxicity were both 99%. The actuarial 5 year and 10 year rates for freedom from grades 3 and 4 GU toxicity were also both 99%.
Bryant et al. published the outcomes of 1327 patients treated between 2006 and 2010. With a median follow-up of 5.5 years, the 5 year freedom from biochemical progression was 99%, 94%, and 74% in low-risk, intermediate-risk, and high-risk patients, respectively [12]. The actuarial 5-year rates of late grade ≥ 3 Common Terminology Criteria for Adverse Events, version 4.0 (CTCAE 4.0), GI and GU toxicities were 0.6% and 2.9%, respectively. There was no significant change in median and mean expanded prostate cancer index (EPIC) summary scores for the bowel, urinary irritative/obstructive, and urinary incontinence domains. Only the sexual function summary scores in patients who did not get hormone ablation therapy significantly declined from baseline to 5 years.
Pugh et al. reported the patient-reported outcomes of 291 patients after proton therapy from the MD Anderson Cancer Center (MDACC) who had a minimum follow-up of 2 years. All patients were treated with opposed lateral beams to a total dose of 76 CGE in 2 CGE fractions. Interestingly, 226 patients were treated with passively scattered proton therapy, and 65 patients were treated with pencil beam proton therapy . Cumulative grade ≥ 2 GU and GI toxicities at 24 months were 13.4% and 9.6%, respectively [13]. There was 1 grade 3 GI toxicity, but no grade ≥ 3 GU toxicity. There was slight higher incidence of argon plasma coagulation application in the passively scattered proton therapy compared to pencil beam proton therapy, but it was not statistically significant (4.4% vs. 1.5%, p = 0.21).
There have been several retrospective comparisons between protons and IMRT. A Surveillance, Epidemiology, and End Results (SEER)-Medicare analysis by Sheets et al. showed a lower rate of GI morbidity (RR 0.66) with IMRT compared to protons in a propensity score-matched comparison. However, this analysis was severely limited as any GI procedure after the treatment was coded as a morbidity. Hoppe et al. performed a comparison of patients undergoing either proton therapy or IMRT using prospectively collected quality of life (QoL) data using the EPIC. There were 1243 proton patients treated with 76–82 CGE and 204 IMRT patients treated with 75.6–79.4 Gy. There was no difference seen between the two groups for the bowel, urinary incontinence, urinary irritative/obstructive, and sexual domains [14]. However, more patients in the IMRT group reported moderate/big problems with rectal urgency (p = 0.02) and frequent bowel movements (p = 0.05) compared to patients in the proton therapy group. Fang et al. compared 181 proton therapy patients and 213 IMRT patients treated between 2010 and 2012 using maximum acute and late GI/GU CTCAE-graded toxicities. On multivariate analysis, there were no statistically significant differences in the acute/late grade ≥ 2 GU and GI toxicities between the two groups [15]. Yu et al. performed an analysis using the Medicare database of 27,647 men. In this analysis, patients who received proton therapy had significant less GU toxicity at 6 months compared to IMRT (5.9% vs. 9.5%, p = 0.03); however, the difference disappeared by 12 months (18.8% vs. 17.5%, p = 0.66) [16]. There was no difference in GI or other toxicities at 6 or 12 months.
Proton therapy may also reduce the risk of secondary cancer when compared to IMRT. Several analyses have predicted that IMRT would increase the risk of secondary cancer over conventional 3D conformalradiation therapy due to the increased scattered dose, while proton therapy would decrease the risk [16–19]. A retrospective matched cohort analysis of 558 proton patients and 558 X-ray patients matched using the SEER registry published by Chung et al. showed that there is a significant reduction of secondary cancer risk (RR 0.52, p = 0.009) in patients treated with proton therapy compared to X-ray therapy. However, due to the limitation of this retrospective study, the authors stated that these results should only be considered as hypothesis generating.
These results show that proton therapy is an effective and safe method for the treatment of prostate cancer. However, as there has been no randomized trial comparing proton therapy to IMRT, it is difficult to know if protons are superior to IMRT in terms of efficacy and/or risk of side effects. There is one randomized trial currently ongoing called the Proton Therapy vs. IMRT for Low or Intermediate Risk Prostate Cancer (PARTIQoL). However, we will need to wait several years until the results of that trial are available.
19.5 Proton Treatment at MD Anderson Cancer Center
19.5.1 Patient Selection
Although the results of the PARTIQoL trial are not yet available, there are probably several specific instances where proton therapy may be especially beneficial. For instance, we recommend proton therapy in younger patients (although the definition of “younger” can vary significantly among clinicians) and patients with larger prostates, especially with a large median lobe. Trying to cover a large median lobe using IMRT usually leads to giving radiation dose to a much larger area of the bladder. However, if a patient has a very large median lobe, it might be worthwhile to consider having the patient undergo a transurethral resection of the prostate (TURP) or greenlight laser enucleation of the median lobe before the radiation therapy. If a patient were to undergo such a procedure, we recommend waiting 2–3 months before the start of the radiation therapy to allow for adequate healing to minimize the risk of urinary incontinence after the radiation therapy.
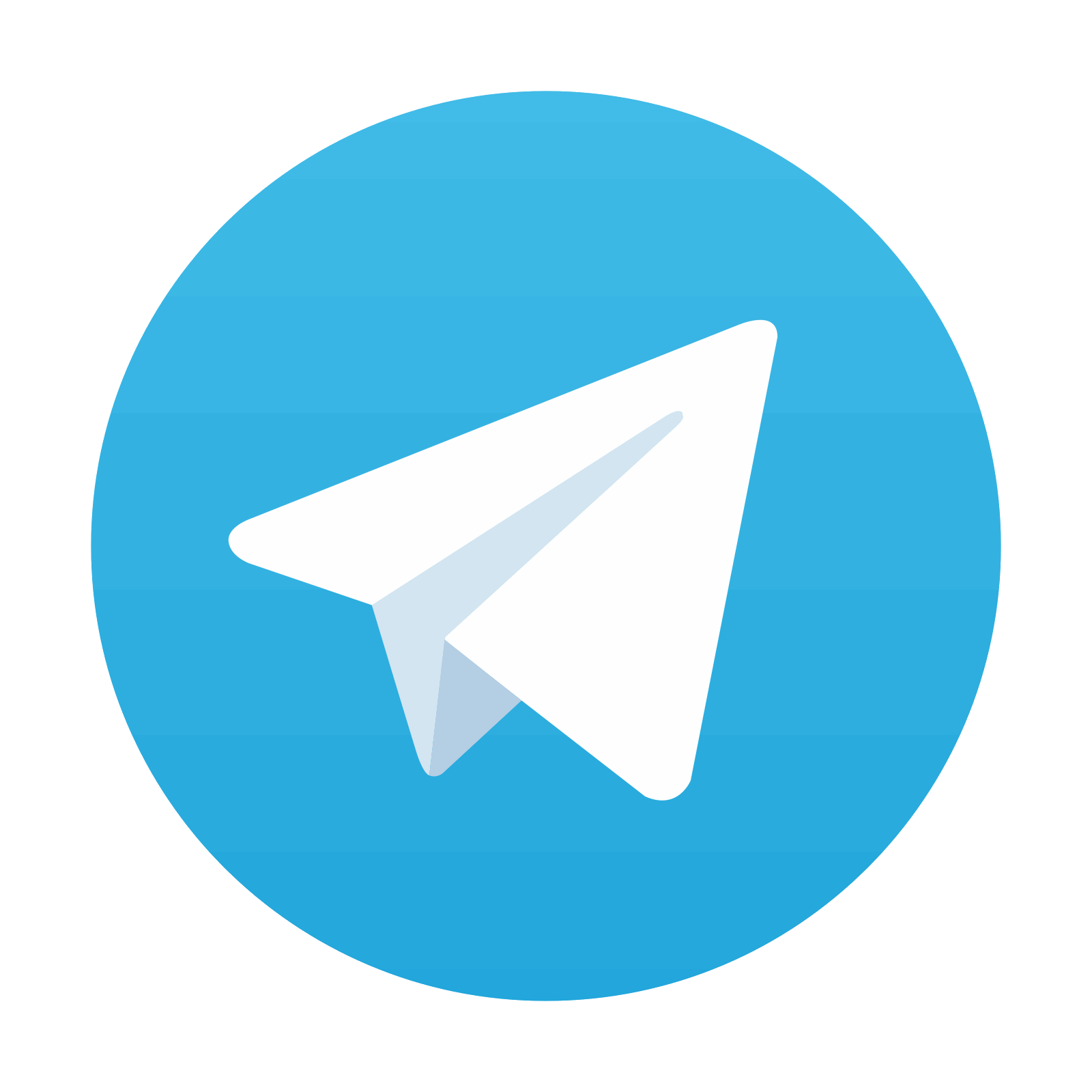
Stay updated, free articles. Join our Telegram channel

Full access? Get Clinical Tree
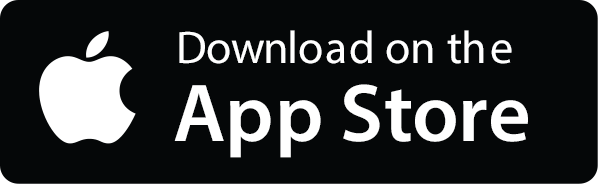
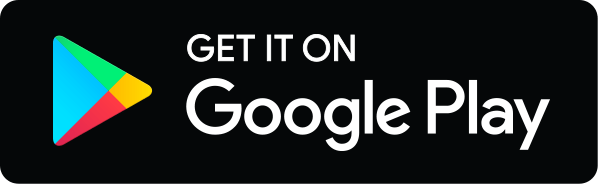