Fig. 26.1
(a, b) Isodose comparison of proton and photon craniospinal irradiation (CSI) with elimination of exit dose through the patient’s neck, chest, abdomen and pelvis. (c) Isodose distribution of typical medulloblastoma with CSI and boost. No additional dose is delivered to the neuroendocrine structures, brain and thyroid gland. (d) Numerical mean and maximum doses to normal structures with proton CSI
As expected, the cranial doses are not different between PRT and XRT for CSI; however, PRT provides an advantage during the boost portion of treatment. For example, as shown in Fig. 26.1, the PRT boost plan for a typical patient with medulloblastoma would allow a reduction in dose to the neuroendocrine structures, optic apparatus, and supratentorial brain structures such as the hippocampi. The above studies also noted a reduction of the total dose delivered to nontarget intracranial structures, including the hypothalamus, pituitary gland, eyes, optic chiasm, and cochlea with PRT (Lee et al. 2005; St Clair et al. 2004).
There has also been interest in using PRT for non-CSI brain irradiation needed in the management of other common pediatric brain tumors such as ependymoma, low-grade glioma, craniopharyngioma, and spinal tumors. Dosimetric studies have also suggested the ability to reduce the integral dose to the intracranial structures outside of the target volume as demonstrated in several early studies (Archambeau et al. 1992; Merchant et al. 2008; Amsbaugh et al. 2012b; Boehling et al. 2012; Eaton and Yock 2014). The models employed in these studies suggest decreased effect on neurocognition. An example of the dosimetric difference between PRT and IMXT for low grade glioma is demonstrated in Fig. 26.2. There is a significant dose reduction to several critical normal tissues as shown by the isodoses and dose volume histograms of the final plan for a typical low grade right frontal astrocytoma. The advantages of CSI with respect to sparing of organs within the torso would apply for patients with spinal canal tumors.
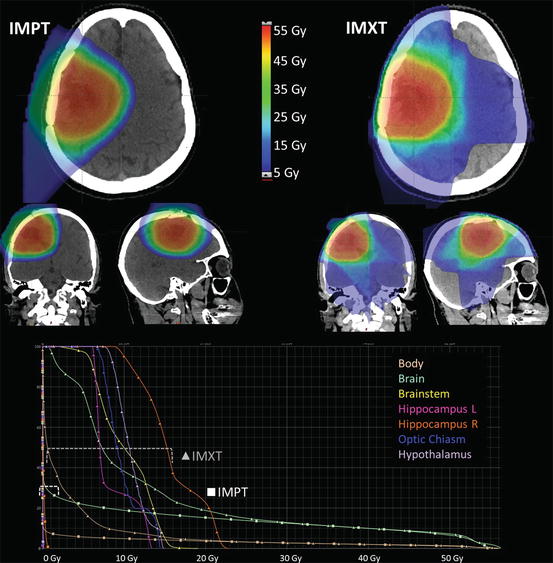
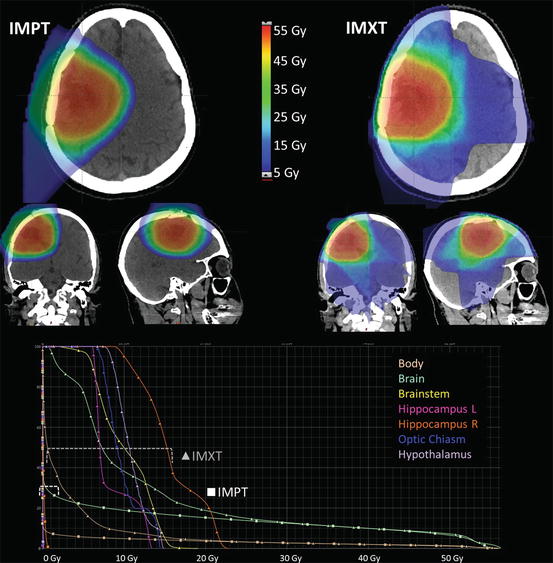
Fig. 26.2
Isodoses and dose volume histogram comparison of proton IMPT and X-ray IMRT radiation plans for a right frontal low grade astrocytoma treated after resection. Overall dose to all adjacent normal structures is lower with the proton plan
26.5 Current Status
PRT has gained acceptance within the pediatric oncology as a treatment option for children with curable brain tumors. PRT is usually not recommended in situations where there is a poor prognosis and limited chance of long-term survival. Efforts have been made to collect annual data on how and why PRT is being used in children. In the United States, the Pediatric Proton Foundation (PPF) collected data indicating an increasing number of children treated with PRT from 465 in 2010 to 989 in 2014. This increase reflects a greater acceptance, awareness, and access to proton centers within the United States. Central nervous system tumors make up approximately 70% of the indications for PRT in the United States with the top three diagnoses being: ependymoma, medulloblastoma, and low-grade glioma. The majority of patients referred to proton centers are younger than 10 years and more than half are under 5 years of age.
In 2015, the Particle Therapy Co-Operative Oncology Group (PTCOG) collected specific data on pediatric patients being treated worldwide in particle therapy centers for the previous year. A total of 1349 children were treated in 29 centers worldwide with PRT (Courtesy of Martin Jermann PTCOG.ch). Further details of the types of tumors will be collected in future surveys.
26.6 Clinical Outcomes
One of the earliest clinical reports on PRT for pediatric brain tumors was by McAllister et al. who reviewed the outcomes of 28 patients. At a median of 25 months, treatment-related toxicity was low. It was also noted that those histologies with poor outcome continued to be a challenge and further follow-up was warranted to evaluate late effects (McAllister et al. 1997). Since then studies have been published evaluating the efficacy of PRT and early toxicities on various tumor histologies including craniopharyngioma, ependymoma, atypical teratoid rhabdoid tumor, and low-grade glioma (Suneja et al. 2013; Neelima et al. 2012; Mizumoto et al. 2015; McGovern et al. 2014; Luu et al. 2006; Lucas et al. 2015; Hug et al. 2002; Fossati et al. 2009; Eaton and Yock 2014; Bian et al. 2013; Amsbaugh et al. 2012a). All studies have reported similar tumor control rates as X-ray therapy with comparable or better toxicity profiles. Long-term outcomes with robust longitudinal evaluations are now emerging.
Yuh et al. first described the outcomes of three 3-year-old girls who were treated with p-CSI from 2001 to 2003. Expected acute toxicities including odynophagia, hoarseness, dysphagia, leukopenia, nausea, and vomiting were absent, but grade 2 dermatitis was noted. Interestingly, in these young patients, the plan did not encompass the whole vertebral circumference in an interest to spare the bone marrow. The authors reported no vertebral growth asymmetry at a time of the report but they cautioned that only a short follow-up was available at that time (Yuh et al. 2004). Acute toxicities in 48 pediatric patients receiving PRT for brain tumors were reported by Suneja et al. In this report 12 patients received p-CSI and compared to the non-CSI group, they were noted to have a higher frequency of grade 2 and 3 anorexia and nausea, but not vomiting, alopecia, or dermatitis (Suneja et al. 2013). In our experience, 40% of our patients experience nausea requiring antiemetic for nausea prophylaxis. Most patients experience some degree of alopecia and dry skin depending on the final dose. Bone marrow suppression and recovery is being actively evaluated (unpublished data). Owusu-Agyemang reviewed the anesthesia experience at our institution of 340 patients treated between 2006 and 2013. One hundred and thirty (40%) patients were treated with p-CSI and anesthesia for all of part of the course of treatment. The treatments were tolerated well with a very low incidence of unexpected events (Owusu-Agyemang et al. 2014).
Ray et al. have reported clinical efficacy on 22 patients with leptomeningeal spinal metastasis who were treated with p-CSI. The authors concluded of these patients, who were treated with definitive intent, 68% had a durable response at 1 year. Treatment toxicities included grade 1 erythema during treatment (Ray et al. 2013). Bian et al. reported favorable outcomes with good tumor control in five of six patients at 24 months post p-CSI for disseminated pilocytic astrocytoma (Bian et al. 2013). Jimenez et al. reported excellent tolerance of p-CSI in 11 young patients (median age 41 months, range 28–62 months) after maximal surgical debulking and pre-radiotherapy chemotherapy. Only 1 of 15 patients had local tumor failure at a median of 39 months post-RT (Jimenez et al. 2013). Yock et al. reported outcomes on 59 pediatric patients with medulloblastoma who were enrolled on a phase II single arm study. Acceptable rates of rates of ototoxicity, cognitive loss, and endocrinopathies compared to historical controls were reported. Progression-free survival was 83% at 3 years and 80% at 5 years. The authors conclude that PRT is an acceptable treatment option for children with medulloblastoma when considering efficacy with a possible reduction in side effects (Yock et al. 2016).
Additional reports have been published describing the various aspects of p-CSI. Moeller et al. reported favorable 1-year auditory outcomes in patients who received p-CSI for medulloblastoma. The authors describe a reduced rate of high degree of hearing loss in patients who had been followed at least a year in comparison to previously published X-ray based CSI treatments (Moeller et al. 2011). Jimenez also described patient toxicity outcomes in a cohort of young patients treated with chemotherapy followed by PRT. Six of the nine patients who had measurable hearing loss had measurable pre-RT loss. All nine had received cisplatinum pre-RT. Three patients had grade 2 endocrinopathies requiring hormone replacement. Early neurocognitive evaluation has not revealed any significant decline in this cohort (Jimenez et al. 2013). Kulthau et al. reported health-related quality of life (HR-QoL) outcomes of 142 patients of whom 61 received p-CSI. Those patients who received p-CSI versus those who received partial brain PRT were noted to have worse score HR-QoL but similar tumor related HR-QoL. In this study, both CSI and chemotherapy were associated with inferior HR-QoL scores (Kuhlthau et al. 2012). Comparison to patients treated with XRT was not available for this cohort.
For other non-CSI indications, results are emerging suggesting a possible therapeutic benefit of PRT with respect to toxicity profiles and tumor control, but prolonged follow-up is still lacking. In particular, neurocognitive, quality of life, auditory, radiographic evaluation and brain stem injury is of great interest (Eaton and Yock 2014; Gunther et al. 2015; Indelicato et al. 2014; Kahalley et al. 2016; Lassen-Ramshad et al. 2015; Pulsifer et al. 2015). One of the most devastating complications of radiotherapy is brain stem necrosis. Indelicato et al. have quantitatively reviewed their experience of brain stem necrosis in 563 pediatric patients treated with PRT between 2007 and 2013 at their institution. The authors reported a 3.8% cumulative risk at 2 years with a 2.1% risk of a grade three or high brain stem injury. Risk factors include younger age, posterior fossa tumor location, and 17% of the brain stem dose receiving 55 Gy or higher. Overall, the authors suggest similar guidelines as what is established for non-PRT modalities; however, further evaluation is ongoing.
Jones et al. raised concerns regarding the possibility of differential relative biologic effectiveness (RBE) between tumor cells and normal tissue. In current practice, an RBE of 1.1 is used for planning purposes but the authors suggest that it may actually vary between 0.8 and 1.2 depending on the type of tissue that is being irradiated (Jones et al. 2012) Additional concerns regarding a varying RBE within the Bragg peak have also been raised (Mohan et al. 2013). Sethi et al. reported the failure patterns in 109 patients with medulloblastoma treated with PRT. There has been no relationship noted between radiotherapy techniques, proton end of range, linear energy transfer (LET), and RBE in the 16 patients who exhibited a relapse during the median follow-up of 38.8 months (Sethi et al. 2014). Further study is required to determine whether differential RBE or LET planning approaches should be considered (Mohan et al. 2013).
Pencil beam PRT has been used for pediatric indications including medulloblastoma, germ cell tumors, and skull base chordoma (Rutz et al. 2008; Park et al. 2015; Lin et al. 2014; Hill-Kayser and Kirk 2015). Preliminary results are promising and as more experience is gained, additional advances in PRT with scanning beam will allow further gains in the management of pediatric brain tumors.
26.7 Non-proton Charged Particles
Other non-proton charged particles such as carbon ion or helium ions may have a role in this group of patients; however, due to the lack of experience with these particles and their effect on normal tissue in children, experience is limited. In the PTCOG survey for 2016, fewer than 50 children were treated with carbon ion worldwide. Additional study is warranted for radioresistant tumors where dose escalation or hypofractionation is of interest.
26.8 Cost-Effectiveness
Since particle therapy systems require a large infrastructure, expensive equipment, and specialized personnel, cost associated with PRT has been under scrutiny. Several authors have evaluated the cost-effectiveness of PRT at a patient and society level. These studies suggest that PRT is a cost-savings approach for treatment for medulloblastoma in children by the reduction of toxicities in comparison to XRT-based techniques. Contributing factors to cost-effectiveness included IQ loss, hearing loss, osteoporosis, cardiac disease, and SMNs (Hirano et al. 2014; Mailhot Vega et al. 2013; Lundkvist et al. 2005). Hirano et al. reported that there was a 99% probability that p-CSI would be cost-effective at a societal willingness to pay value with respect to hearing loss and cochlear dose (Hirano et al. 2014). Mailhot et al. evaluated the impact of the reduced need for growth hormone in patients where the neuroendocrine structures were spared with PRT. They concluded that PRT was cost-effective with regard to growth hormone replacement needs if the hypothalamus and pituitary gland could be spared in comparison to XRT techniques (Mailhot Vega et al. 2015). Verma et al. reviewed the literature to identify where PRT may be the most cost-effective, and they concluded that PRT was most valuable in several pediatric brain tumors (Verma et al. 2016).
26.9 Ethics
Concern has been raised regarding the ethical considerations of the increased expense and possible lack of access for children to proton therapy centers. Wolden argues that at this time long-term outcomes are lacking to uniformly support PRT for all patients requiring CSI despite the theoretical advantages and early results (Wolden 2013). Johnstone et al. argue that the PRT centers are obliged to treat all patients who are referred to them because of the theoretical advantages and existing clinical data and also stated that parents should be given counseling regarding the option for PRT so they can make an informed decision on their child’s behalf (Johnstone et al. 2013).
Conclusions
PRT for pediatric brain tumors has been studied and increasingly used over the past 15 years. From the (1) preliminary theoretical considerations, (2) multiple dosimetric evaluations, (3) predictive model applications, and (4) clinical outcomes, there is mounting evidence that PRT provides equal tumor control and will be associated with fewer RT-related toxicities. PRT in children can be time-consuming and technically challenging and the utmost attention should be paid to every aspect of the treatment. Future refinements including the use of pencil beam PRT may allow further reduction in normal tissue doses and neutron contamination. Additional thought to RBE or LET weighted planning is also warranted to refine the treatment planning and improve the therapeutic ratio.
References
Amsbaugh MJ, Grosshans DR, McAleer MF, Zhu R, Wages C, Crawford CN, Palmer M, De Gracia B, Woo S, Mahajan A (2012a) Proton therapy for spinal ependymomas: planning, acute toxicities, and preliminary outcomes. Int J Radiat Oncol Biol Phys 83(5):1419–1424. doi:10.1016/j.ijrobp.2011.10.034 CrossrefPubMed
Amsbaugh MJ, Zhu XR, Palmer M, Poenisch F, McAleer MF, Mahajan A, Grosshans DR (2012b) Spot scanning proton therapy for craniopharyngioma. Pract Radiat Oncol 2(4):314–318. doi:10.1016/j.prro.2012.01.001 CrossrefPubMed
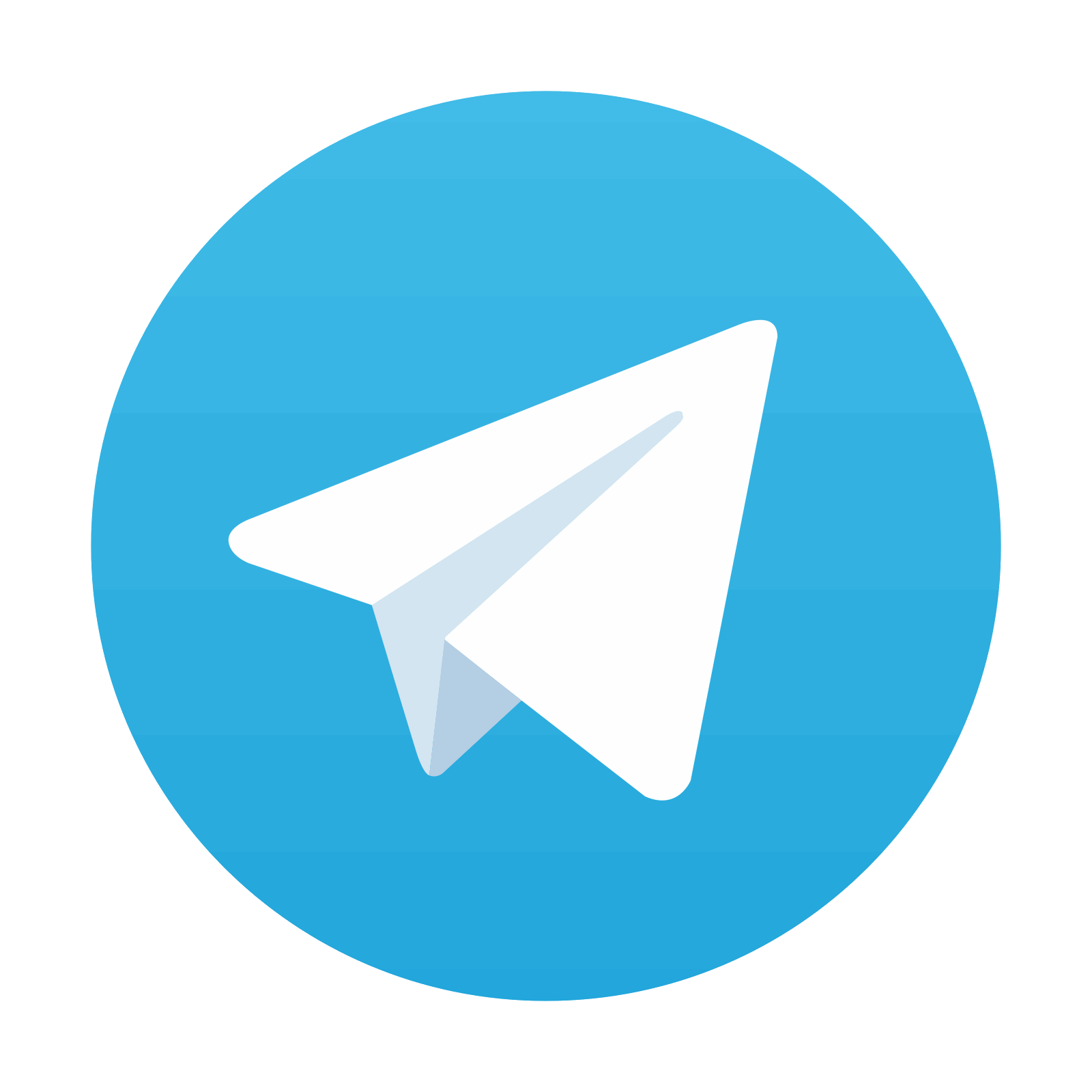
Stay updated, free articles. Join our Telegram channel

Full access? Get Clinical Tree
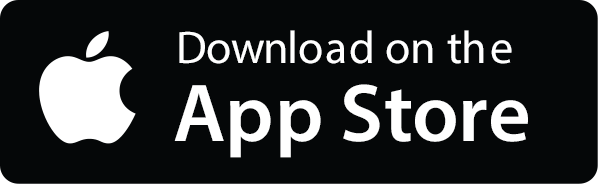
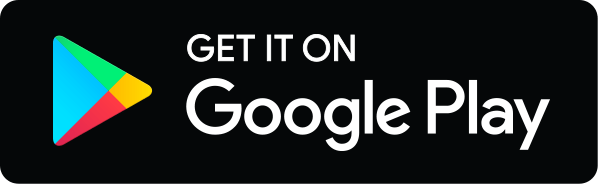